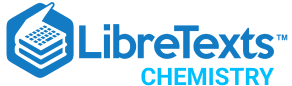
- school Campus Bookshelves
- menu_book Bookshelves
- perm_media Learning Objects
- login Login
- how_to_reg Request Instructor Account
- hub Instructor Commons
- Download Page (PDF)
- Download Full Book (PDF)
- Periodic Table
- Physics Constants
- Scientific Calculator
- Reference & Cite
- Tools expand_more
- Readability
selected template will load here
This action is not available.
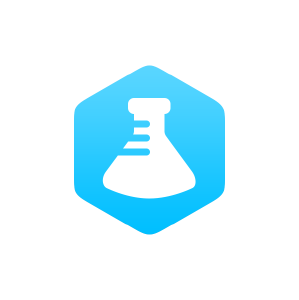
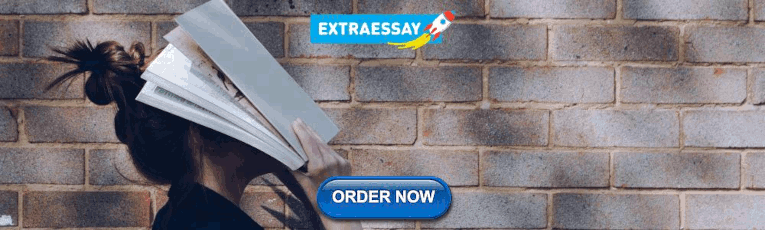
12.7: Electrophoresis
- Last updated
- Save as PDF
- Page ID 70722
Electrophoresis is a class of separation techniques in which we separate analytes by their ability to move through a conductive medium—usually an aqueous buffer—in response to an applied electric field. In the absence of other effects, cations migrate toward the electric field’s negatively charged cathode. Cations with larger charge-to-size ratios—which favors ions of larger charge and of smaller size—migrate at a faster rate than larger cations with smaller charges. Anions migrate toward the positively charged anode and neutral species do not experience the electrical field and remain stationary.
There are several forms of electrophoresis. In slab gel electrophoresis the conducting buffer is retained within a porous gel of agarose or polyacrylamide. Slabs are formed by pouring the gel between two glass plates separated by spacers. Typical thicknesses are 0.25–1 mm. Gel electrophoresis is an important technique in biochemistry where it is frequently used for separating DNA fragments and proteins. Although it is a powerful tool for the qualitative analysis of complex mixtures, it is less useful for quantitative work.
In capillary electrophoresis , the conducting buffer is retained within a capillary tube whose inner diameter is typically 25–75 μm. Samples are injected into one end of the capillary tube. As the sample migrates through the capillary its components separate and elute from the column at different times. The resulting electropherogram looks similar to a GC or an HPLC chromatogram, providing both qualitative and quantitative information. Only capillary electrophoretic methods receive further consideration in this section.
As we will see shortly, under normal conditions even neutral species and anions migrate toward the cathode.
12.7.1 Theory of Capillary Electrophoresis
In capillary electrophoresis we inject the sample into a buffered solution retained within a capillary tube. When an electric field is applied across the capillary tube, the sample’s components migrate as the result of two types of action: electrophoretic mobility and electroosmotic mobility. Electrophoretic mobility is the solute’s response to the applied electrical field. As described earlier, cations move toward the negatively charged cathode, anions move toward the positively charged anode, and neutral species remain stationary. The other contribution to a solute’s migration is electroosmotic flow , which occurs when the buffer moves through the capillary in response to the applied electrical field. Under normal conditions the buffer moves toward the cathode, sweeping most solutes, including the anions and neutral species, toward the negatively charged cathode.
Electrophoretic Mobility
The velocity with which a solute moves in response to the applied electric field is called its electrophoretic velocity , ν ep ; it is defined as
\[ν_\ce{ep}= \mu_\ce{ep}E \tag{12.43}\]
where μ ep is the solute’s electrophoretic mobility, and E is the magnitude of the applied electrical field. A solute’s electrophoretic mobility is defined as
\[\mu_\ce{ep} = \dfrac{q}{6\pi ηr } \tag{12.35}\]
- q is the solute’s charge,
- η is the buffer viscosity, and
- r is the solute’s radius.
Using equation 12.34 and equation 12.35 we can make several important conclusions about a solute’s electrophoretic velocity. Electrophoretic mobility and, therefore, electrophoretic velocity, increases for more highly charged solutes and for solutes of smaller size. Because q is positive for a cation and negative for an anion, these species migrate in opposite directions. Neutral species, for which q is zero, have an electrophoretic velocity of zero.
Electroosmotic Mobility
When an electrical field is applied to a capillary filled with an aqueous buffer we expect the buffer’s ions to migrate in response to their electrophoretic mobility. Because the solvent, H 2 O, is neutral we might reasonably expect it to remain stationary. What we observe under normal conditions, however, is that the buffer solution moves towards the cathode. This phenomenon is called the electroosmotic flow.
Electroosmotic flow occurs because the walls of the capillary tubing are electrically charged. The surface of a silica capillary contains large numbers of silanol groups (–SiOH). At pH levels greater than approximately 2 or 3, the silanol groups ionize to form negatively charged silanate ions (–SiO – ). Cations from the buffer are attracted to the silanate ions. As shown in Figure 12.56, some of these cations bind tightly to the silanate ions, forming a fixed layer. Because the cations in the fixed layer only partially neutralize the negative charge on the capillary walls, the solution adjacent to the fixed layer—what we call the diffuse layer—contains more cations than anions. Together these two layers are known as the double layer. Cations in the diffuse layer migrate toward the cathode. Because these cations are solvated, the solution is also pulled along, producing the electroosmotic flow.
The anions in the diffuse layer, which also are solvated, try to move toward the anode. Because there are more cations than anions, however, the cations win out and the electroosmotic flow moves in the direction of the cathode.

Figure 12.56: Schematic diagram showing the origin of the double layer within a capillary tube. Although the net charge within the capillary is zero, the distribution of charge is not. The walls of the capillary have an excess of negative charge, which decreases across the fixed layer and the diffuse layer, reaching a value of zero in bulk solution.
The rate at which the buffer moves through the capillary, what we call its electroosmotic flow velocity , ν eof , is a function of the applied electric field, E , and the buffer’s electroosmotic mobility, μ eof .
\[\nu_\ce{eof} = \mu_\ce{eof}E \tag{12.36}\]
Electroosmotic mobility is defined as
\[\mu_\ce{eof} = \dfrac{εζ}{4πη} \tag{12.37}\]
- ε is the buffer dielectric constant,
- ζ is the zeta potential, and
- η is the buffer viscosity.
The zeta potential —the potential of the diffuse layer at a finite distance from the capillary wall—plays an important role in determining the electroosmotic flow velocity. Two factors determine the zeta potential’s value. First, the zeta potential is directly proportional to the charge on the capillary walls, with a greater density of silanate ions corresponding to a larger zeta potential. Below a pH of 2 there are few silanate ions, and the zeta potential and electroosmotic flow velocity are zero. As the pH increases, both the zeta potential and the electroosmotic flow velocity increase. Second, the zeta potential is directly proportional to the thickness of the double layer. Increasing the buffer’s ionic strength provides a higher concentration of cations, decreasing the thickness of the double layer and decreasing the electroosmotic flow.
Note: Zeta Potential
The definition of zeta potential given here is admittedly a bit fuzzy. For a much more technical explanation see Delgado, A. V.; González-Caballero, F.; Hunter, R. J.; Koopal, L. K.; Lyklema, J. “Measurement and Interpretation of Electrokinetic Phenomena,” Pure. Appl. Chem. 2005, 77, 1753–1805. Although this a very technical report, Sections 1.3–1.5 provide a good introduction to the difficulty of defining the zeta potential and measuring its value.
The electroosmotic flow profile is very different from that of a fluid moving under forced pressure. Figure 12.57 compares the electroosmotic flow profile with that the hydrodynamic flow profile in gas chromatography and liquid chromatography. The uniform, flat profile for electroosmosis helps minimize band broadening in capillary electrophoresis, improving separation efficiency.
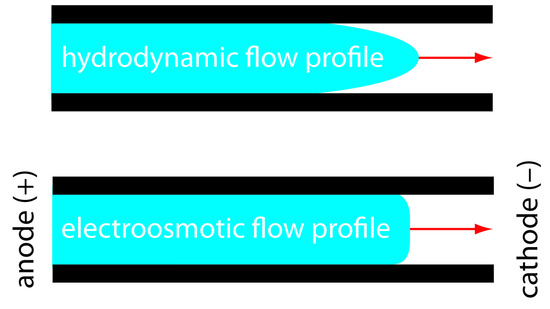
Figure 12.57: Comparison of hydrodynamic flow and electroosmotic flow. The nearly uniform electroosmotic flow profile means that the electroosmotic flow velocity is nearly constant across the capillary.
Total Mobility
A solute’s total velocity, \(v_{tot}\), as it moves through the capillary is the sum of its electrophoretic velocity and the electroosmotic flow velocity.
\[ν_\ce{tot} =ν_\ce{ep} + ν_\ce{eof}\]
As shown in Figure 12.58, under normal conditions the following general relationships hold true.
\[(ν_\ce{tot})_\ce{cations} > ν_\ce{eof}\]
\[(ν_\ce{tot})_\ce{neutrals} = ν_\ce{eof}\]
\[(ν_\ce{tot})_\ce{anions} < ν_\ce{eof}\]
Cations elute first in an order corresponding to their electrophoretic mobilities, with small, highly charged cations eluting before larger cations of lower charge. Neutral species elute as a single band with an elution rate equal to the electroosmotic flow velocity. Finally, anions are the last components to elute, with smaller, highly charged anions having the longest elution time.

Figure 12.58: Visual explanation for the general elution order in capillary electrophoresis. Each species has the same electroosmotic flow, ν eof . Cations elute first because they have a positive electrophoretic velocity, ν ep . Anions elute last because their negative electrophoretic velocity partially offsets the electroosmotic flow velocity. Neutrals elute with a velocity equal to the electroosmotic flow.
Migration Time
Another way to express a solute’s velocity is to divide the distance it travels by the elapsed time
\[ν_\ce{tot} = \ce{l}{t_\ce{m}}\tag{12.38}\]
where l is the distance between the point of injection and the detector, and t m is the solute’s migration time. To understand the experimental variables affecting migration time, we begin by noting that
\[ν_\ce{tot} = \mu_\ce{tot}E= (\mu_\ce{ep} + \mu_\ce{eof})E\tag{12.39}\]
Combining equation 12.38 and equation 12.39 and solving for t m leaves us with
\[t_\ce{m} = \dfrac{l}{(\mu_\ce{ep} + \mu_\ce{eof})E}\tag{12.40}\]
Finally, the magnitude of the electrical field is
\[E = \dfrac{V}{L}\tag{12.41}\]
where V is the applied potential and L is the length of the capillary tube. Finally, substituting equation 12.41 into equation 12.40 leaves us with the following equation for a solute’s migration time.
\[t_\ce{m}= \dfrac{lL}{(\mu_\ce{ep} + \mu_\ce{eof})V}\tag{12.42}\]
To decrease a solute’s migration time—and shorten the analysis time—we can apply a higher voltage or use a shorter capillary tube. We can also shorten the migration time by increasing the electroosmotic flow, although this decreases resolution.
As we learned in Section 12.2.4, the efficiency of a separation is given by the number of theoretical plates, N . In capillary electrophoresis the number of theoretic plates is
\[N = \dfrac{l^2}{2Dt_\ce{m}} = \dfrac{(\mu_\ce{ep} + \mu_\ce{eof})Vl}{2DL} \tag{12.43}\]
where \(D\) is the solute’s diffusion coefficient.
From equation 12.10 and equation 12.11, we know that the number of theoretical plates for a solute is
\[N = \dfrac{l^2}{\sigma ^2}\]
where l is the distance the solute travels and σ is the standard deviation for the solute’s band broadening. For capillary electrophoresis band broadening is due to longitudinal diffusion and is equivalent to 2 Dt m , where t m is the migration time.
From equation 12.43, the efficiency of a capillary electrophoretic separation increases with higher voltages. Increasing the electroosmotic flow velocity improves efficiency, but at the expense of resolution. Two additional observations deserve comment. First, solutes with larger electrophoretic mobilities—in the same direction as the electroosmotic flow—have greater efficiencies; thus, smaller, more highly charged cations are not only the first solutes to elute, but do so with greater efficiency. Second, efficiency in capillary electrophoresis is independent of the capillary’s length. Theoretical plate counts of approximately 100,000–200,000 are not unusual.
It is possible to design an electrophoretic experiment so that anions elute before cations—more about this later—in which smaller, more highly charged anions elute with greater efficiencies.
Selectivity
In chromatography we defined the selectivity between two solutes as the ratio of their retention factors (see equation 12.9). In capillary electrophoresis the analogous expression for selectivity is
\[α = \dfrac{\mu_\textrm{ep,1}}{\mu_\textrm{ep,2}}\]
where μ ep ,1 and μ ep ,2 are the electrophoretic mobilities for the two solutes, chosen such that α ≥ 1. We can often improve selectivity by adjusting the pH of the buffer solution. For example, NH 4 + is a weak acid with a p K a of 9.75. At a pH of 9.75 the concentrations of NH 4 + and NH 3 are equal. Decreasing the pH below 9.75 increases its electrophoretic mobility because a greater fraction of the solute is present as the cation NH 4 + . On the other hand, raising the pH above 9.75 increases the proportion of the neutral NH 3 , decreasing its electrophoretic mobility.
The resolution between two solutes is
\[R = \dfrac{0.177(\mu_\textrm{ep,1} - \mu_\textrm{ep,2})\sqrt{V}}{\sqrt{D(\mu_\textrm{avg} - \mu_\textrm{eof})}}\tag{12.44}\]
where μ avg is the average electrophoretic mobility for the two solutes. Increasing the applied voltage and decreasing the electroosmotic flow velocity improves resolution. The latter effect is particularly important. Although increasing electroosmotic flow improves analysis time and efficiency, it decreases resolution.
12.7.2 Instrumentation
The basic instrumentation for capillary electrophoresis is shown in Figure 12.59 and includes a power supply for applying the electric field, anode and cathode compartments containing reservoirs of the buffer solution, a sample vial containing the sample, the capillary tube, and a detector. Each part of the instrument receives further consideration in this section.
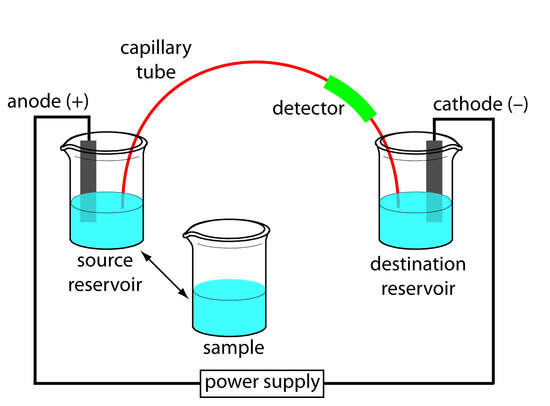
Figure 12.59 Schematic diagram of the basic instrumentation for capillary electrophoresis. The sample and the source reservoir are switched when making injections.
Capillary Tubes
Figure 12.60 shows a cross-section of a typical capillary tube. Most capillary tubes are made from fused silica coated with a 15–35 μm layer of polyimide to give it mechanical strength. The inner diameter is typically 25–75 μm—smaller than the internal diameter of a capillary GC column—with an outer diameter of 200–375 μm.
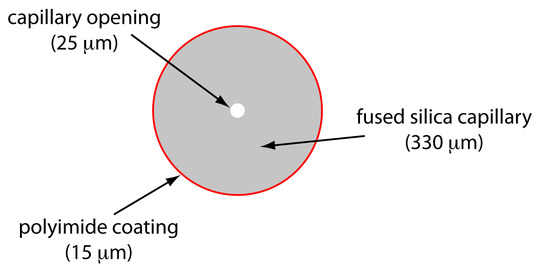
Figure 12.60 Cross section of a capillary column for capillary electrophoresis. The dimensions shown here are typical and are scaled proportionally.
The capillary column’s narrow opening and the thickness of its walls are important. When an electric field is applied to the buffer solution within the capillary, current flows through the capillary. This current leads to the release of heat—what we call Joule heating . The amount of heat released is proportional to the capillary’s radius and the magnitude of the electrical field. Joule heating is a problem because it changes the buffer solution’s viscosity, with the solution at the center of the capillary being less viscous than that near the capillary walls. Because a solute’s electrophoretic mobility depends on viscosity (see equation 12.35), solute species in the center of the capillary migrate at a faster rate than those near the capillary walls. The result is an additional source of band broadening that degrades the separation. Capillaries with smaller inner diameters generate less Joule heating, and capillaries with larger outer diameters are more effective at dissipating the heat. Placing the capillary tube inside a thermostated jacket is another method for minimizing the effect of Joule heating; in this case a smaller outer diameter allows for a more rapid dissipation of thermal energy.
Injecting the Sample
There are two commonly used method for injecting a sample into a capillary electrophoresis column: hydrodynamic injection and electrokinetic injection. In both methods the capillary tube is filled with the buffer solution. One end of the capillary tube is placed in the destination reservoir and the other end is placed in the sample vial.
Hydrodynamic injection uses pressure to force a small portion of sample into the capillary tubing. A difference in pressure is applied across the capillary by either pressurizing the sample vial or by applying a vacuum to the destination reservoir. The volume of sample injected, in liters, is given by the following equation
\[V_\ce{inj}= \dfrac{Pd^4πt}{128ηL}× 10^3\tag{12.45}\]
where ∆ P is the difference in pressure across the capillary in pascals, d is the capillary’s inner diameter in meters, t is the amount of time that the pressure is applied in seconds, η is the buffer’s viscosity in kg m –1 s –1 , and L is the length of the capillary tubing in meters. The factor of 10 3 changes the units from cubic meters to liters.
For a hydrodynamic injection we move the capillary from the source reservoir to the sample. The anode remains in the source reservoir.
A hydrodynamic injection is also possible by raising the sample vial above the destination reservoir and briefly inserting the filled capillary.
If you want to verify the units in equation 12.45, recall from Table 2.2 that 1 Pa is equivalent to 1 kg m -1 s -2 .
Example 12.9
In a hydrodynamic injection we apply a pressure difference of 2.5 × 10 3 Pa (a ∆ P ≈ 0.02 atm) for 2 s to a 75-cm long capillary tube with an internal diameter of 50 μm. Assuming that the buffer’s viscosity is 10 –3 kg m –1 s –1 , what volume and length of sample did we inject?
Making appropriate substitutions into equation 12.45 gives the sample’s volume as
\[\begin{align} V_\ce{inj} &= \mathrm{\dfrac{(2.5×10^3\: kg\: m^{−1}\: s^{−2})(50×10^{−6}\: m)^4(3.14)(2\: s)}{(128)(0.001\: kg\: m^{−1}\: s^{−1})(0.75\:m)} × 10^3\: L/m^3}\\ V_\ce{inj} &= \mathrm{1×10^{−9}\: L = 1\: nL} \end{align}\]
Because the interior of the capillary is cylindrical, the length of the sample, l , is easy to calculate using the equation for the volume of a cylinder; thus
\[l = \dfrac{V_\ce{inj}}{πr^2} = \mathrm{\dfrac{(1.0×10^{−9}\: L)(10^{−3}\: m^3/L)}{(3.14)(25×10^{−6}\: m)^2} = 5×10^{−4}\: m = 0.5\: mm}\]
Exercise 12.9
Suppose that you need to limit your injection to less than 0.20% of the capillary’s length. Using the information from Example 12.9, what is the maximum injection time for a hydrodynamic injection?
Click here to review your answer to this exercise.
In an electrokinetic injection we place both the capillary and the anode into the sample and briefly apply an potential. The volume of injected sample is the product of the capillary’s cross sectional area and the length of the capillary occupied by the sample. In turn, this length is the product of the solute’s velocity (see equation 12.39) and time; thus
- \(r\) is the capillary’s radius,
- \(L\) is the length of the capillary, and
- \(E′\) is effective electric field in the sample.
An important consequence of equation 12.46 is that an electrokinetic injection is inherently biased toward solutes with larger electrophoretic mobilities. If two solutes have equal concentrations in a sample, we inject a larger volume—and thus more moles—of the solute with the larger μ ep .
The electric field in the sample is different that the electric field in the rest of the capillary because the sample and the buffer have different ionic compositions. In general, the sample’s ionic strength is smaller, which makes its conductivity smaller. The effective electric field is
\[E′ = E \times \dfrac{κ_\ce{buf}}{κ_\ce{sam}}\]
where κ buf and κ sam are the conductivities of the buffer and the sample, respectively.
When an analyte’s concentration is too small to detect reliably, it may be possible to inject it in a manner that increases its concentration in the capillary tube. This method of injection is called stacking . Stacking is accomplished by placing the sample in a solution whose ionic strength is significantly less than that of the buffer in the capillary tube. Because the sample plug has a lower concentration of buffer ions, the effective field strength across the sample plug, E ′ is larger than that in the rest of the capillary.
We know from equation 12.34 that electrophoretic velocity is directly proportional to the electrical field. As a result, the cations in the sample plug migrate toward the cathode with a greater velocity, and the anions migrate more slowly—neutral species are unaffected and move with the electroosmotic flow. When the ions reach their respective boundaries between the sample plug and the buffering solution, the electrical field decreases and the electrophoretic velocity of cations decreases and that for anions increases. As shown in Figure 12.61, the result is a stacking of cations and anions into separate, smaller sampling zones. Over time, the buffer within the capillary becomes more homogeneous and the separation proceeds without additional stacking.
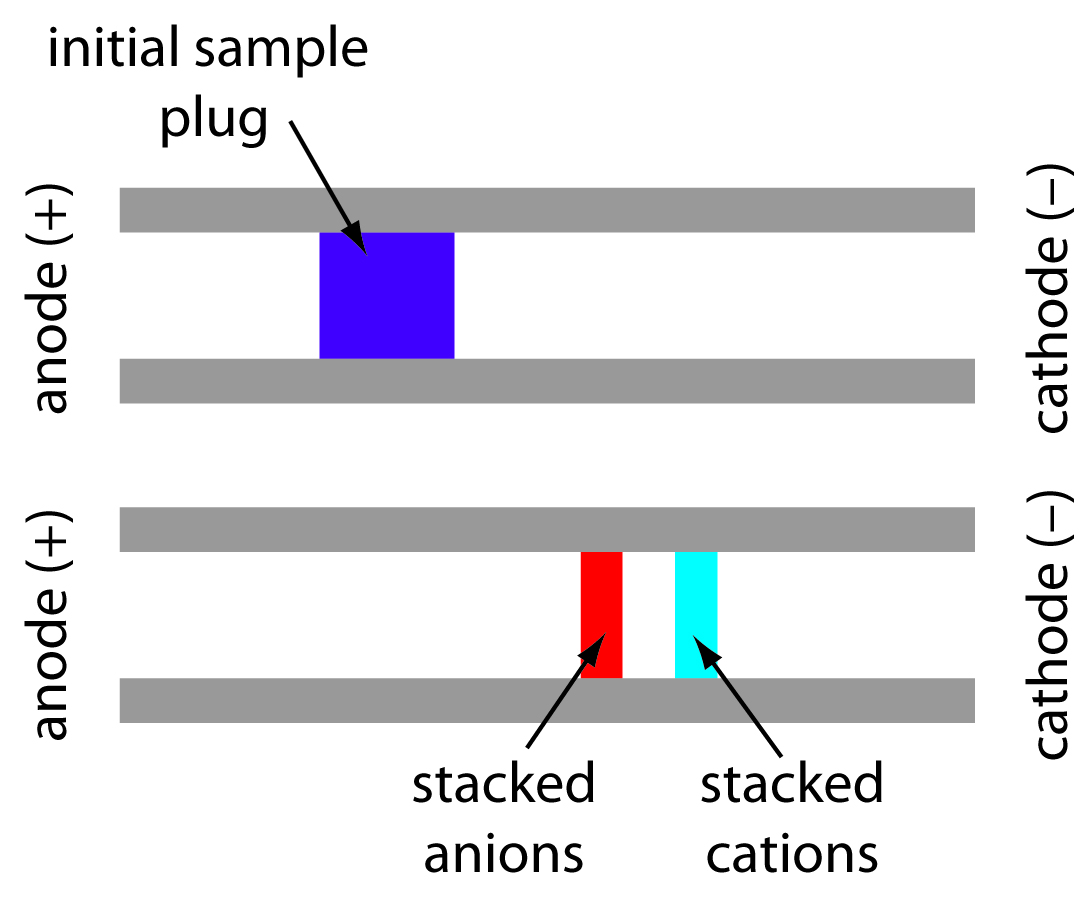
Figure 12.61 The stacking of cations and anions. The top diagram shows the initial sample plug and the bottom diagram shows how the cations and anions become concentrated at opposite sides of the sample plug.
Applying the Electrical Field
Migration in electrophoresis occurs in response to an applied electrical field. The ability to apply a large electrical field is important because higher voltages lead to shorter analysis times (see equation 12.42), more efficient separations (equation 12.43), and better resolution (equation 12.44). Because narrow bored capillary tubes dissipate Joule heating so efficiently, voltages of up to 40 kV are possible.
Because of the high voltages, be sure to follow your instrument’s safety guidelines.
Most of the detectors used in HPLC also find use in capillary electrophoresis. Among the more common detectors are those based on the absorption of UV/Vis radiation, fluorescence, conductivity, amperometry, and mass spectrometry. Whenever possible, detection is done “on-column” before the solutes elute from the capillary tube and additional band broadening occurs.
UV/Vis detectors are among the most popular. Because absorbance is directly proportional to path length, the capillary tubing’s small diameter leads to signals that are smaller than those obtained in HPLC. Several approaches have been used to increase the pathlength, including a Z-shaped sample cell and multiple reflections (see Figure 12.62). Detection limits are about 10 –7 M.
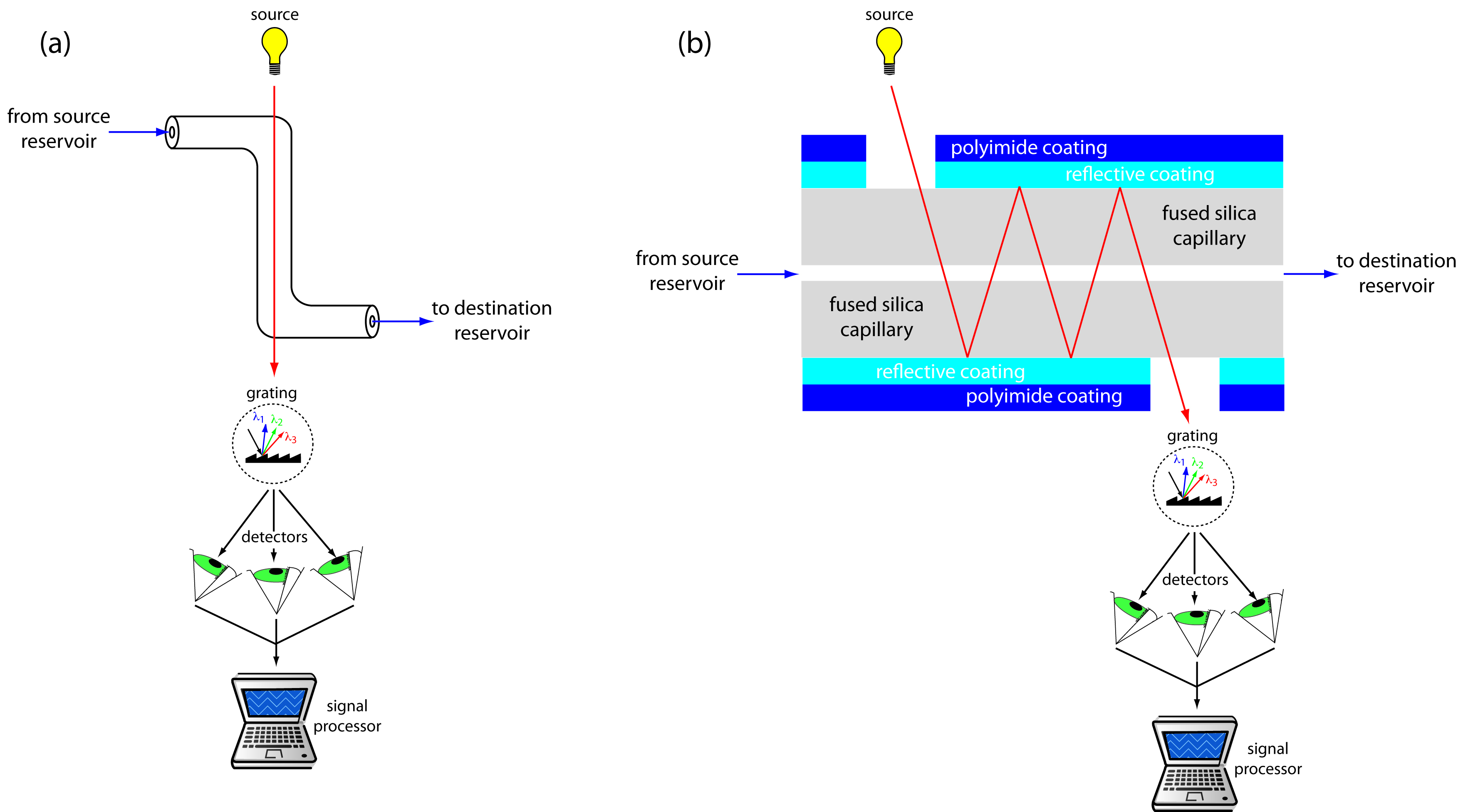
Figure 12.62 Two approaches to on-column detection in capillary electrophoresis using a UV/Vis diode array spectrometer: (a) Z-shaped bend in capillary, and (b) multiple reflections.
Better detection limits are obtained using fluorescence, particularly when using a laser as an excitation source. When using fluorescence detection a small portion of the capillary’s protective coating is removed and the laser beam is focused on the inner portion of the capillary tubing. Emission is measured at an angle of 90 o to the laser. Because the laser provides an intense source of radiation that can be focused to a narrow spot, detection limits are as low as 10 –16 M.
Solutes that do not absorb UV/Vis radiation or that do not undergo fluorescence can be detected by other detectors. Table 12.10 provides a list of detectors for capillary electrophoresis along with some of their important characteristics.
Source: Baker, D. R. Capillary Electrophoresis , Wiley-Interscience: New York, 1995.
12.7.3 Capillary Electrophoresis Methods
There are several different forms of capillary electrophoresis, each of which has its particular advantages. Four of these methods are briefly described in this section.
Capillary Zone Electrophoresis (CZE)
The simplest form of capillary electrophoresis is capillary zone electrophoresis . In CZE we fill the capillary tube with a buffer solution and, after loading the sample, place the ends of the capillary tube in reservoirs containing additional buffer solution. Usually the end of the capillary containing the sample is the anode and solutes migrate toward the cathode at a velocity determined by their electrophoretic mobility and the electroosmotic flow. Cations elute first, with smaller, more highly charged cations eluting before larger cations with smaller charges. Neutral species elute as a single band. Anions are the last species to elute, with smaller, more negatively charged anions being the last to elute.
We can reverse the direction of electroosmotic flow by adding an alkylammonium salt to the buffer solution. As shown in Figure 12.63, the positively charged end of the alkyl ammonium ions bind to the negatively charged silanate ions on the capillary’s walls. The tail of the alkyl ammonium ion is hydrophobic and associates with the tail of another alkyl ammonium ion. The result is a layer of positive charges that attract anions in the buffer solution. The migration of these solvated anions toward the anode reverses the electroosmotic flow’s direction. The order of elution is exactly opposite of that observed under normal conditions.
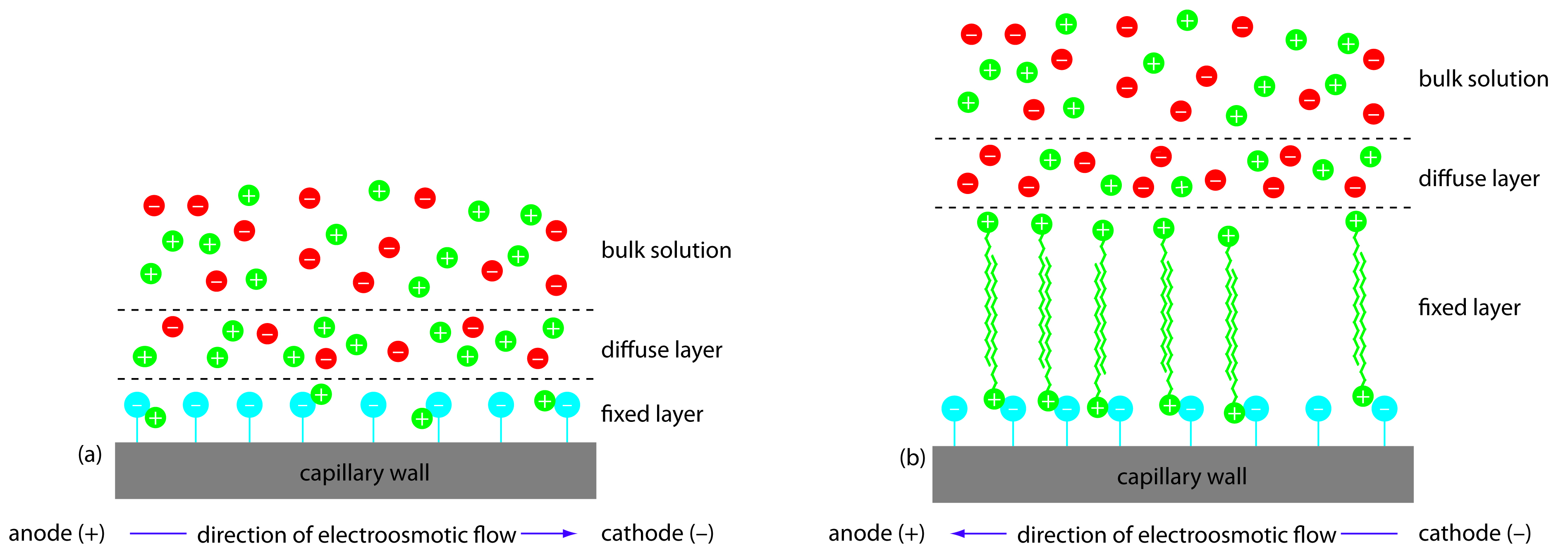
Figure 12.63 Two modes of capillary zone electrophoresis showing (a) normal migration with electroosmotic flow toward the cathode and (b) reversed migration in which the electroosmotic flow is toward the anode.
Coating the capillary’s walls with a nonionic reagent eliminates the electroosmotic flow. In this form of CZE the cations migrate from the anode to the cathode. Anions elute into the source reservoir and neutral species remain stationary.
Capillary zone electrophoresis provides effective separations of charged species, including inorganic anions and cations, organic acids and amines, and large biomolecules such as proteins. For example, CZE has been used to separate a mixture of 36 inorganic and organic ions in less than three minutes. 15 A mixture of neutral species, of course, can not be resolved.
Micellar Electrokinetic Capillary Chromatography (MEKC)
One limitation to CZE is its inability to separate neutral species. Micellar electrokinetic capillary chromatography overcomes this limitation by adding a surfactant, such as sodium dodecylsulfate (Figure 12.64a) to the buffer solution. Sodium dodecylsulfate, or SDS, has a long-chain hydrophobic tail and a negatively charged ionic functional group at its head. When the concentration of SDS is sufficiently large a micelle forms. A micelle consists of a spherical agglomeration of 40–100 surfactant molecules in which the hydrocarbon tails point inward and the negatively charged heads point outward (Figure 12.64b).
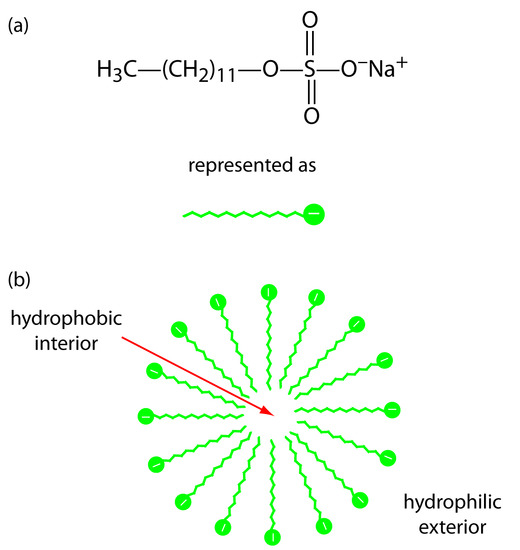
Figure 12.64 : (a) Structure of sodium dodecylsulfate and its representation, and (b) cross section through a micelle showing its hydrophobic interior and its hydrophilic exterior.
Because micelles have a negative charge, they migrate toward the cathode with a velocity less than the electroosmotic flow velocity. Neutral species partition themselves between the micelles and the buffer solution in a manner similar to the partitioning of solutes between the two liquid phases in HPLC. Because there is a partitioning between two phases, we include the descriptive term chromatography in the techniques name. Note that in MEKC both phases are mobile.
The elution order for neutral species in MEKC depends on the extent to which each partitions into the micelles. Hydrophilic neutrals are insoluble in the micelle’s hydrophobic inner environment and elute as a single band, as they would in CZE. Neutral solutes that are extremely hydrophobic are completely soluble in the micelle, eluting with the micelles as a single band. Those neutral species that exist in a partition equilibrium between the buffer solution and the micelles elute between the completely hydrophilic and completely hydrophobic neutral species. Those neutral species favoring the buffer solution elute before those favoring the micelles. Micellar electrokinetic chromatography has been used to separate a wide variety of samples, including mixtures of pharmaceutical compounds, vitamins, and explosives.
Capillary Gel Electrophoresis (CGE)
In capillary gel electrophoresis the capillary tubing is filled with a polymeric gel. Because the gel is porous, a solute migrates through the gel with a velocity determined both by its electrophoretic mobility and by its size. The ability to effect a separation using size is helpful when the solutes have similar electrophoretic mobilities. For example, fragments of DNA of varying length have similar charge-to-size ratios, making their separation by CZE difficult. Because the DNA fragments are of different size, a CGE separation is possible.
The capillary used for CGE is usually treated to eliminate electroosmotic flow, preventing the gel’s extrusion from the capillary tubing. Samples are injected electrokinetically because the gel provides too much resistance for hydrodynamic sampling. The primary application of CGE is the separation of large biomolecules, including DNA fragments, proteins, and oligonucleotides.
Capillary Electrochromatography (CEC)
Another approach to separating neutral species is capillary electrochromatography . In CEC the capillary tubing is packed with 1.5–3 μm particles coated with a bonded stationary phase. Neutral species separate based on their ability to partition between the stationary phase and the buffer, which is moving as a result of the electroosmotic flow; Figure 12.65 provides a representative example for the separation of a mixture of hydrocarbons. A CEC separation is similar to the analogous HPLC separation, but without the need for high pressure pumps. Efficiency in CEC is better than in HPLC, and analysis times are shorter.

Figure 12.65 Capillary electrochromatographic separation of a mixture of hydrocarbons in DMSO. The column contains a porous polymer of butyl methacrylate and lauryl acrylate (25%:75% mol:mol) with butane dioldacrylate as a crosslinker. Data provided by Zoe LaPier and Michelle Bushey, Department of Chemistry, Trinity University.
The best way to appreciate the theoretical and practical details discussed in this section is to carefully examine a typical analytical method. Although each method is unique, the following description of the determination of a vitamin B complex by capillary zone electrophoresis or by micellar electrokinetic capillary chromatography provides an instructive example of a typical procedure. The description here is based on Smyth, W. F. Analytical Chemistry of Complex Matrices , Wiley Teubner: Chichester, England, 1996, pp. 154–156.
Representative Method 12.3
Determination of a Vitamin B Complex by CZE or MEKC
Description of Method
The water soluble vitamins B 1 (thiamine hydrochloride), B 2 (riboflavin), B 3 (niacinamide), and B 6 (pyridoxine hydrochloride) are determined by CZE using a pH 9 sodium tetraborate-sodium dihydrogen phosphate buffer or by MEKC using the same buffer with the addition of sodium dodecyl sulfate. Detection is by UV absorption at 200 nm. An internal standard of o -ethoxybenzamide is used to standardize the method.
Crush a vitamin B complex tablet and place it in a beaker with 20.00 mL of a 50 % v/v methanol solution that is 20 mM in sodium tetraborate and 100.0 ppm in o -ethoxybenzamide. After mixing for 2 min to ensure that the B vitamins are dissolved, pass a 5.00-mL portion through a 0.45-μm filter to remove insoluble binders. Load an approximately 4 nL sample into a capillary column with an inner diameter of a 50 μm. For CZE the capillary column contains a 20 mM pH 9 sodium tetraborate-sodium dihydrogen phosphate buffer. For MEKC the buffer is also 150 mM in sodium dodecyl sulfate. Apply a 40 kV/m electrical field to effect both the CZE and MEKC separations.
1. Methanol, which elutes at 4.69 min, is included as a neutral species to indicate the electroosmotic flow. When using standard solutions of each vitamin, CZE peaks are found at 3.41 min, 4.69 min, 6.31 min, and 8.31 min. Examine the structures and p K a information in Figure 12.66 and identify the order in which the four B vitamins elute.
Vitamin B 1 is a cation and elutes before the neutral species methanol; thus it is the compound that elutes at 3.41 min. Vitamin B 3 is a neutral species and elutes with methanol at 4.69 min. The remaining two B vitamins are weak acids that partially ionize to weak base anions in the pH 9 buffer. Of the two, vitamin B 6 is the stronger acid (a p K a of 9.0 versus a p K a of 9.7) and is present to a greater extent in its anionic form. Vitamin B 6 , therefore, is the last of the vitamins to elute.
2. The order of elution when using MEKC is vitamin B 3 (5.58 min), vitamin B 6 (6.59 min), vitamin B 2 (8.81 min), and vitamin B 1 (11.21 min). What conclusions can you make about the solubility of the B vitamins in the sodium dodecylsulfate micelles? The micelles elute at 17.7 min.
The elution time for vitamin B 1 shows the greatest change, increasing from 3.41 min to 11.21 minutes. Clearly vitamin B 1 has the greatest solubility in the micelles. Vitamin B 2 and vitamin B 3 have a more limited solubility in the micelles, showing only slightly longer elution times in the presence of the micelles. Interestingly, the elution time for vitamin B 6 decreases in the presence of the micelles.
3. For quantitative work an internal standard of o-ethoxybenzamide is added to all samples and standards. Why is an internal standard necessary?
Although the method of injection is not specified, neither a hydrodynamic injection nor an electrokinetic injection is particularly reproducible. The use of an internal standard compensates for this limitation.
(You can read more about the use of internal standards in capillary electrophoresis in the following paper: Altria, K. D. “Improved Performance in Capillary Electrophoresis Using Internal Standards,” LC.GC Europe, September 2002 .)
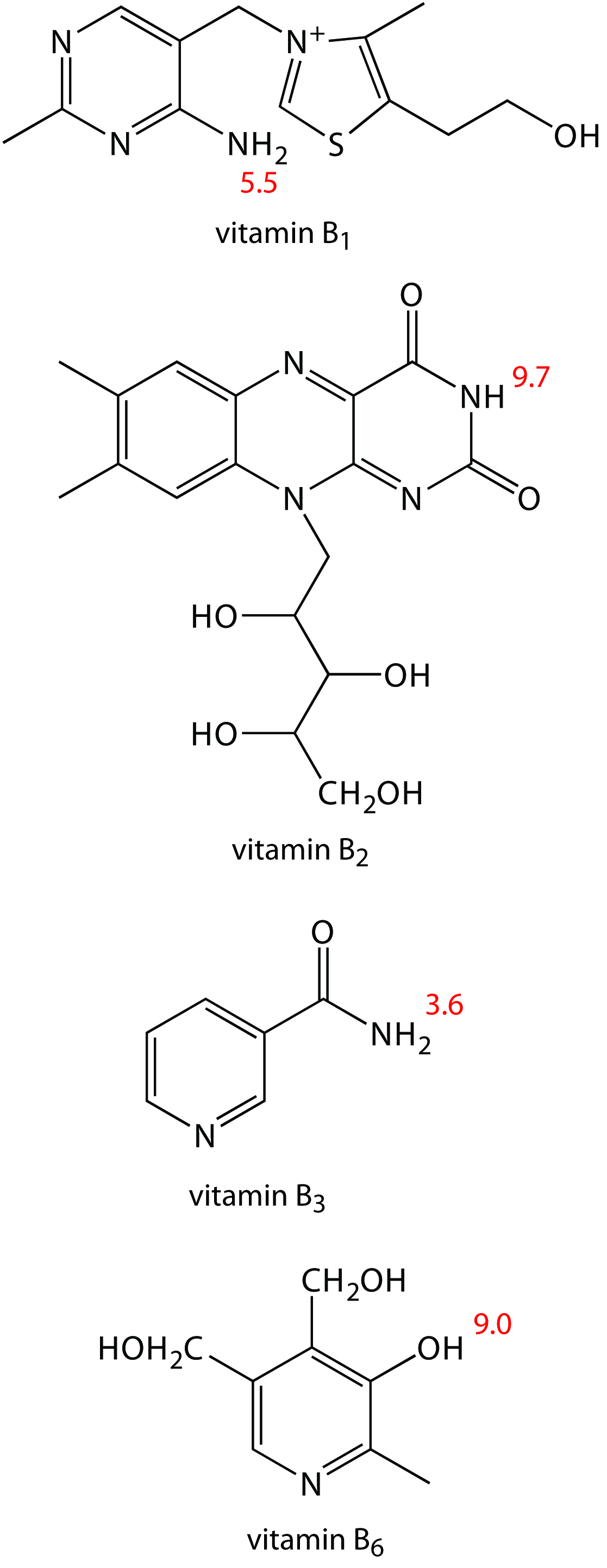
Figure 12.66: Structures of the four water soluble B vitamins in their predominate forms at a pH of 9; pK a values are shown in red .
12.7.4 Evaluation
When compared to GC and HPLC, capillary electrophoresis provides similar levels of accuracy, precision, and sensitivity, and a comparable degree of selectivity. The amount of material injected into a capillary electrophoretic column is significantly smaller than that for GC and HPLC—typically 1 nL versus 0.1 μL for capillary GC and 1–100 μL for HPLC. Detection limits for capillary electrophoresis, however, are 100–1000 times poorer than that for GC and HPLC. The most significant advantages of capillary electrophoresis are improvements in separation efficiency, time, and cost. Capillary electrophoretic columns contain substantially more theoretical plates (≈10 6 plates/m) than that found in HPLC (≈10 5 plates/m) and capillary GC columns (≈10 3 plates/m), providing unparalleled resolution and peak capacity. Separations in capillary electrophoresis are fast and efficient. Furthermore, the capillary column’s small volume means that a capillary electrophoresis separation requires only a few microliters of buffer solution, compared to 20–30 mL of mobile phase for a typical HPLC separation.
See Section 12.4.8 for an evaluation of gas chromatography, and Section 12.5.6 for an evaluation of high-performance liquid chromatography.
Contributors
David Harvey (DePauw University)
Thank you for visiting nature.com. You are using a browser version with limited support for CSS. To obtain the best experience, we recommend you use a more up to date browser (or turn off compatibility mode in Internet Explorer). In the meantime, to ensure continued support, we are displaying the site without styles and JavaScript.
- View all journals
Electrophoresis articles from across Nature Portfolio
Electrophoresis is the process by which large charged molecules travel through a medium under a uniform electric field. The most common implementation, gel electrophoresis, is a method used to separate nucleic acids by length or proteins by size, conformation and charge based on their migration through a porous matrix.
Latest Research and Reviews
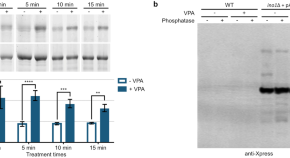
Valproate regulates inositol synthesis by reducing expression of myo -inositol-3-phosphate synthase
- Kendall C. Case
- Rachel J. Beltman
- Miriam L. Greenberg
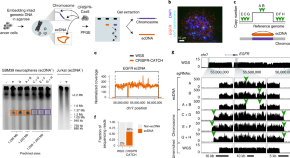
Targeted profiling of human extrachromosomal DNA by CRISPR-CATCH
CRISPR-CATCH is used to isolate extrachromosomal DNA (ecDNA) molecules containing oncogenes from human cancer cells. CRISPR-CATCH followed by nanopore sequencing allows for methylation profiling, highlighting differences from the native chromosomal loci.
- King L. Hung
- Jens Luebeck
- Howard Y. Chang
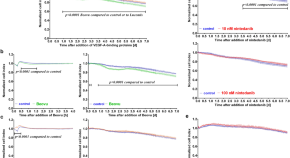
Beovu, but not Lucentis impairs the function of the barrier formed by retinal endothelial cells in vitro
- Heidrun L. Deissler
- Catharina Busch
- Matus Rehak
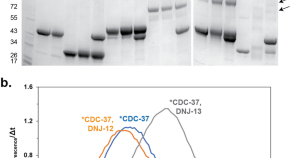
Nematode CDC-37 and DNJ-13 form complexes and can interact with HSP-90
- Lukas Schmauder
- Eva Absmeier
- Klaus Richter
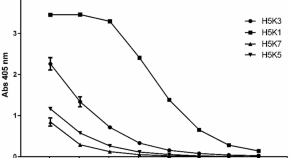
A new humanized antibody is effective against pathogenic fungi in vitro
- Tomas Di Mambro
- Tania Vanzolini
- Mauro Magnani
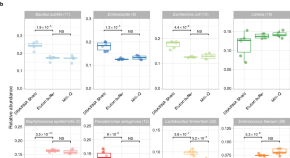
Benchmarking laboratory processes to characterise low-biomass respiratory microbiota
- Raiza Hasrat
- Jolanda Kool
- Thijs Bosch
News and Comment
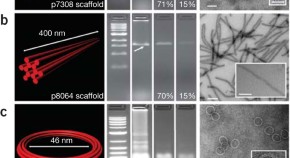
Recovery of intact DNA nanostructures after agarose gel–based separation
- Gaëtan Bellot
- Mark A McClintock
- William M Shih
Guidelines for reporting the use of gel image informatics in proteomics
- Christine Hoogland
- Martin O'Gorman
- Andrew R Jones
Guidelines for reporting the use of capillary electrophoresis in proteomics
- Paula J Domann
- Satoko Akashi
- Chris F Taylor
Quick links
- Explore articles by subject
- Guide to authors
- Editorial policies

Open Access is an initiative that aims to make scientific research freely available to all. To date our community has made over 100 million downloads. It’s based on principles of collaboration, unobstructed discovery, and, most importantly, scientific progression. As PhD students, we found it difficult to access the research we needed, so we decided to create a new Open Access publisher that levels the playing field for scientists across the world. How? By making research easy to access, and puts the academic needs of the researchers before the business interests of publishers.
We are a community of more than 103,000 authors and editors from 3,291 institutions spanning 160 countries, including Nobel Prize winners and some of the world’s most-cited researchers. Publishing on IntechOpen allows authors to earn citations and find new collaborators, meaning more people see your work not only from your own field of study, but from other related fields too.
Brief introduction to this section that descibes Open Access especially from an IntechOpen perspective
Want to get in touch? Contact our London head office or media team here
Our team is growing all the time, so we’re always on the lookout for smart people who want to help us reshape the world of scientific publishing.
Home > Books > Biochemical Testing - Clinical Correlation and Diagnosis
Serum Protein Electrophoresis and Its Clinical Applications
Submitted: 10 April 2018 Reviewed: 03 July 2019 Published: 11 October 2019
DOI: 10.5772/intechopen.88367
Cite this chapter
There are two ways to cite this chapter:
From the Edited Volume
Biochemical Testing - Clinical Correlation and Diagnosis
Edited by Varaprasad Bobbarala, Gaffar Sarwar Zaman, Mohd Nasir Mohd Desa and Abdah Md Akim
To purchase hard copies of this book, please contact the representative in India: CBS Publishers & Distributors Pvt. Ltd. www.cbspd.com | [email protected]
Chapter metrics overview
2,824 Chapter Downloads
Impact of this chapter
Total Chapter Downloads on intechopen.com

Total Chapter Views on intechopen.com
This chapter focuses on the principle of electrophoresis and its utilization in a clinical laboratory. A sincere attempt has been made to discuss about clinical applications of serum protein electrophoresis, throwing light on the significance of serum protein electrophoresis in the management of multiple myeloma. Emphasis has been made on quality assurance in terms of accuracy and precision in electrophoresis to ensure reliability of patient results. A note on issues with lack of standardization of reporting of electrophoresis and an insight into global efforts to standardize the reporting of the assay has been included in this chapter.
- electrophoresis
- gamma globulins
- oligoclonal
Author Information
Satish ramanathan *.
- Division of Clinical Biochemistry, MIOT Hospitals, India
Chakravarthy Narasimhachar Srinivas
- Laboratory Medicine, MIOT Hospitals, India
*Address all correspondence to: [email protected]
1. Introduction
Serum protein electrophoresis is an electrophoretic method of separating proteins present in the serum to various fractions based on their molecular weight and electric charges. Electrophoresis had been widely used in clinical medicine for aiding in diagnosis of various clinical conditions like acute and chronic inflammations, monoclonal gammopathies, nephropathy, liver diseases, etc. This chapter discusses the clinical applications of serum protein electrophoresis [ 1 ] including the quality control practices and its implications [ 2 ].
2. Principle
The separation of proteins by electrophoresis is based on the fact that charged molecules usually migrate through a matrix/medium upon application of an electrical field [ 3 ]. The rate at which proteins move in an electric field is determined by a number of factors of the electrophoretic system and the nature of proteins itself. Some factors to mention are the strength of the electric field, temperature of the system, pH of the ions, concentration of buffer etc. [ 4 ]. Proteins vary in their size and shape and have the charges determined by the dissociation contents of their amino acids. Smaller proteins usually migrate faster, and larger proteins take a longer time. This physical property of proteins is exploited for its separation by employing the electrophoretic technique.
The use of “electroendosmosis” principle which improves the resolution of separation
Employing a “high-voltage” electric current which aids in improving the throughput (the processing time) and the resolution of protein separation.
Below is an illustration of capillary electrophoresis (Sebia Minicap Flex Piercing) ( Figure 1 ). Sebia Minicap Flex Piercing capillary electrophoresis works on the principle of capillary electroendosmosis under high-voltage electric current. The Flex Piercing model of Sebia CZE aids in testing of human blood with capped tubes which in turn eliminates the biohazard xassociated with handling of uncapped samples.

Sebia Minicap flex piercing capillary electrophoresis.
3. Revisiting the basics: an insight into the protein family
Serum proteins are a family of albumin and globulins. Albumin is the major fraction synthesized from human liver endogenously and available through various dietary sources exogenously including egg, meat, pulses, milk etc. Globulins are a group of proteins subclassified into alpha-1, alpha-2, beta-1, beta-2, and gamma globulins based on the electrophoretic mobility ( Figure 2 ). The normal biological interval of serum total proteins in a healthy adult ranges between 6 and 8 g/dl which includes Serum Albumin: 3.5–4.5 g/dl and Globulins: 2.5–3.5 g/dl.

Serum protein family with fractions.
3.1 Albumin
Albumin is a 69 kDa protein. It is the most abundant protein in serum. Albumin is synthesized in the liver and functions as a transport protein of various substances like bilirubin, enzymes, hormones, drugs etc. It also maintains fluid volume within the vascular space. Albumin is the first protein fraction to appear near the anode in SPE. Altered levels of serum albumin are associated with various clinical conditions. Low levels of albumin are clinically significant and are termed as hypoalbuminemia.
Decreased concentration of serum albumin (hypoalbuminemia) indicates either a poor dietary intake (malnutrition) or a decreased production or an increased loss. Chronic liver disease is a common clinical condition associated with decreased albumin production, and chronic kidney disease (CKD) is the most common disease associated with an increased loss of albumin in urine (proteinuria). This clinical condition is otherwise known as nephropathy. Other causes of hypoalbuminemia include acute and chronic inflammation, critical illness, pregnancy etc.
Bilirubin, Triglycerides if present in high levels in serum may appear as a blunt peak which is seen adjacent to the cathode near the albumin peak.
Prealbumin (transthyretin)—increased levels of pre albumin, if present due to various clinical conditions including several inflammatory diseases is seen as a blunt anodal peak distinctly separated from the peak of albumin.
A rare variant observed in the albumin peak is bisalbuminemia which is a rare condition, with no clinical features, in which the serum contains two albumin variants of different electrophoretic mobilities, usually in equal concentrations, though the total concentration of albumin is normal. Bisalbuminemia may be hereditary or acquired. The acquired type has been more frequently reported in chronic renal disease and pancreatitis and in patient with chronic renal disease. Two (rather than one) albumin bands may represent bisalbuminemia. Hereditary condition is a rare anomaly caused by a genetic lesion in the albumin gene usually a point mutation.
Analbuminemia (absence of albumin) is another genetically inherited metabolic disorder and was first described in 1954. This disorder is rare and affects less than 1 in 1 million births.
The most important aspect of such albumin variants lies in quantification of an albumin peak in such scenarios followed by interpretation and clinical correlation ( Figure 3 ).

Abnormal electrophoretic patterns of albumin zone.
3.2 Alpha fraction
As electrophoresis proceeds toward the negative portion of the gel (cathode), the alpha zone is the next band after albumin. The alpha zone is subdivided into two zones: the alpha-1 peak and alpha-2 peak.
The alpha-1 peak consists of alpha-1 antitrypsin (AT), alpha-1-chymotrypsin, and thyroid-binding globulin. Alpha-1 antitrypsin is an acute-phase reactant. The concentration of alpha-1 antitrypsin increases in conditions of inflammation and is usually decreased in patients with alpha-1 antitrypsin deficiency or decreased production of globulin in patients with severe liver disease. A rare variant of alpha-1 antitrypsin is encountered occasionally characterized by a split peak pattern of alpha-1 globulins.
The alpha-2 peak consists of alpha-2 macroglobulin, haptoglobin, and ceruloplasmin. Alpha-2 macroglobulin accounts for about 3% of the total protein in the serum. Because of the variable migration of the haptoglobin types, a2-macroglobulin is often adjacent to, or co migrating with, haptoglobin and is therefore not seen as a discrete band.
A distorted pattern of alpha-2 region in electrophoresis is seen commonly in conditions of hemolysis, including in vivo and in vitro. The pathophysiology behind this pattern is the formation of hemoglobin-haptoglobin complexes in these conditions. This is a physiological adaptive response by human physiology to conserve hemoglobin released as a result of RBC breakdown into circulation and hemoglobin being a smaller globular protein is bound to be lost in urine. Hence to preserve it, haptoglobin is consumed to form complex with hemoglobin which results in the formation of a macromolecular protein which is retained in circulation making hemoglobin available for the production of RBCs and prevention of anemia.
Haptoglobin and ceruloplasmin are acute-phase reactants, and hence increased in acute inflammatory states.
Alpha-2-macroglobulin is increased in nephrotic syndrome and cirrhosis of the liver. The elevation of alpha-2 macroglobulin is distinctly evident in nephritic syndrome, since it is a bulky molecule, and hence retained in circulation to compensate for the loss of other proteins in urine which is evident in form of proteinuria in urine microscopic examination.
Ceruloplasmin is an important copper-binding transport protein produced by the liver. Ceruloplasmin concentrations are markedly decreased in conditions of Wilson’s disease. The disadvantage of serum protein electrophoresis is that it will not aid in the detection of a decreased ceruloplasmin.
3.3 Beta fraction
The beta zone usually is subdivided into two peaks, beta-1 and beta-2 in CZE. Beta-1 zone comprises proteins like transferrin and low-density lipoprotein (LDL).
Transferrin functions to transport non-heme ferric iron from the gastrointestinal tract. Each Transferrin molecule can bind two molecules of free iron. An increased beta-1 band is observed in iron deficiency anemia due to an increased level of free transferrin and also in pregnancy. Determinations of the transferrin levels are useful in distinguishing between iron deficiency anemia (inadequate intake or chronic hemorrhage with loss of iron stores) and hemolytic anemia, in which transferrin levels are low resulting in a beta-1 peak of low amplitude. Transferrin is usually decreased in alcoholic cirrhosis. Transferrin is also decreased during renal disease and thermal injuries.
The beta-2 band is mostly composed of complement proteins, C3 and C4. Elevated beta-2 zone can be caused in inflammatory states due to activation of complement cascade which include C3 and C4 too.
A reduced beta-2 peak intensity can be encountered in an aged sample, since the immune complexes are used up and low serum levels of complements are evidenced.
Fibrinogen is a protein with molecular weight of 340 kDa protein. Sometimes a small fibrinogen band can be seen in serum protein electrophoresis due to the insufficient clotting or failure to remove the serum from the clot. This fibrinogen band is seen between beta-1 and beta-2 regions. This band is also seen in patients who are receiving heparin therapy. It is also an important indicator of the sample type being analyzed. When plasma is used in the place of serum for protein electrophoresis, fibrinogen present in plasma appears in the beta-2 region, and this has the potentiality to interfere with the detection of monoclonal gammopathies in such patients ( Figure 4 ).

Fibrinogen producing a peak in beta 2 region (from a plasma sample).
3.4 Gamma fraction
One of the main clinical implications of serum protein electrophoresis is to aid diagnosis of disorders associated with alterations of gamma globulins. Gamma region comprises mainly of serum immunoglobulins. The five major classes of immunoglobulins are IgG, IgA, IgM, IgD, and IgE. The immunoglobulins are characterized by the presence of two protein moieties named as heavy chain and light chain. The classification of immunoglobulin had been made based on the composition of heavy chains, while the light chains are of two types including kappa or lambda. Physiologically, kappa forms the major light chain fraction among the two.
Hypergammaglobinemia (increased serum gamma globulin levels)
Hypogammaglobinemia (decreased serum gamma globulin levels)
Hypergammaglobinemia (gammopathies):
Gammopathy is defined as abnormal proliferation of the lymphoid cells producing immunoglobulins. There are four types of gammopathies: polyclonal, monoclonal, biclonal, and oligoclonal.
Polyclonal gammopathies are defined as heterogeneous increase in immunoglobulins involving more than one cell line, commonly caused by a variety of inflammatory conditions (chronic inflammation), infections, chronic liver diseases (cirrhosis), chronic kidney diseases, etc.
Monoclonal gammopathies are characterized by a homogenous increase produced by clonal population of mature B cells, most commonly plasma cells. Monoclonal immunoglobulins seen in these conditions are also known as Para proteins. The classic interpretative terminology used in clinical laboratory medicine for describing a monoclonal immunoglobulin in SPE is “M” band where M stands for monoclonal. Common clinical disorders producing “M” Band in SPE include multiple myeloma and plasmacytoma in usually 60% of cases and Waldenströms Macroglobulinemia, lymphomas, and leukemia in approximately 10% of cases. Certain monoclonal gammopathies produce “M” band in electrophoretic regions other than in gamma regions, commonly being beta region especially in case of IgA and IgG myeloma.
Biclonal gammopathies are characterized by a double peak in the gamma region. This electrophoretic pattern is seen when there is a biclonal proliferation of immunoglobulins encountered in multiple myeloma. A biclonal pattern is also seen in monoclonal gammopathies associated with IgA and IgG. In such scenarios, these immunoglobulins appear as polymerized and monomerized forms which elute as biclonal peaks in gamma region or in beta region, respectively ( Figure 5 ).

Abnormal electrophoretic patterns of gamma zone.
The oligoclonal pattern of gamma region is characterized by more than two peaks evident in the gamma region. This pattern is commonly seen in autoimmune disorders, light chain myelomas (characterized by clonal proliferation of light chains), amyloidosis, etc. ( Figure 5 ).
Apart from serum immunoglobulin, C-reactive protein (CRP) also is evident in the gamma region. C-reactive proteins levels usually increase during inflammatory responses.
Apart from the common causes of altered electrophoresis picture specific to the particular zones, a sharp distinct peak when evident especially in beta or alpha region should raise a high index of diagnostic suspicion of multiple myeloma since a few monoclonal immunoglobulins shall migrate in these zones too, in contrary to the classical gamma zone M protein pattern, which is commonly reported in these conditions.
4. Role of SPE in multiple myeloma work-up
MGUS—monoclonal gammopathy of undetermined significance
MGRS—monoclonal gammopathy of renal significance
Smoldering myeloma
Multiple myeloma (which includes various subtypes including nonsecretory myeloma (NSMM), light chain myeloma, secretory multiple myeloma)
Monoclonal gammopathy of undetermined significance (MGUS)
M protein (Monoclonal band)—<3 g/dl
Bone marrow biopsy—<10% plasma cells seen
No clinical symptoms/signs
Normbal free light chain ratio in serum
Monoclonal gammopathy of renal significance (MGRS)
M protein (monoclonal band)—<3 g/dl
Renal disease with elevated serum creatinine
Normal Free light chain ratio in serum
Bone marrow biopsy—>10% plasma cells seen
Abnormal free light chain ratio in serum
Clinically significant. Clinical diagnosis includes a tetrad of “ÇRAB” which stands for (one of the four shall be present):
C—hypercalcemia
R—renal abnormalities (elevated creatinine)
A—anemia
B—bone lesions
Multiple myeloma
M protein (monoclonal band)—>3 g/dl
Clinical diagnosis includes a tetrad of “ÇRAB” which stands for (one of the four shall be present):
A —anemia
There are exceptions in SPE findings in certain cases of multiple myeloma wherein the SPE does not reveal any significant alteration or a clue toward the diagnosis.
These variants of multiple myeloma characterized by an abnormal bone marrow (increased plasma cells) but a normal SPE are termed as nonsecretory myelomas which account to 1–2% of multiple myelomas. In such cases, an immunoassay of free light chains (FLC) in serum provides a diagnostic clue toward NSMM which show a significant disproportionate elevation of usually a clone of light chains (kappa or lambda) with an alteration in kappa/lambda ratio (normal Ratio is between 0.60 and 1.65). A commonly encountered phenomenon with laboratory testing of FLC includes “prozone” effect or “hook” effect which occurs due to antigen excess and requires appropriate dilution to obtain reliable results.
Bence-Jones protein estimation in urine is an antique piece of laboratory evidence toward multiple myeloma, which is characterized by detection of light chains in urine. But since the methodology of testing is manual and does not provide standardization, this has been replaced by urine FLC analysis in laboratories practicing good clinical laboratory practices (GCLP).
One more valiant laboratory investigation which is an essential requisite for multiple myeloma work-up includes immunoelectrophoresis.
One common principle employed in immunoelectrophoretic technique involves the use of specific antihuman immunoglobulins (e.g., Anti-IgG, Anti-IgA, Anti-Kappa, etc.) as a preprocessing step which results in precipitation of immunoglobulins if present and disappearance of the band/peak contributed by that specific immunoglobulin. Hence this technique is also known as immunosubtraction. This technique aids in typing the specific type of immunoglobulin (including the type of light chain) contributing to myeloma. This technique is supplemented by quantification of serum immunoglobulins by an immunoassay.
4.1 SPE and its clinical significance
SPE is a semiquantitative investigation which involves technical expertise to recognize the specific electrophoretic patterns and associate with various clinical conditions. This requires a laboratory practice integrated across various divisions of laboratory and with respective clinical and ancillary divisions of clinical medicine [ 1 ].
With respect to SPE, the laboratory professionals shall act as consultants to the clinical consultants. This is possible in scenarios where the clinician does not arrive at a provisional diagnosis of a gammopathy and the laboratory picks up the diagnostic clue toward gammopathy through an increased serum total protein level (<8 g/dl) and an altered serum albumin globulin (AG) ratio (which is usually altered in gammopathy). A normal AG ratio ranges between 1.2 and 1.8, while there is a significant reduction in the ratio in patients with gammopathy. This becomes an incidental finding which leads to a concept of “reflex” testing for multiple myeloma work-up including SPE, upon consent from the treating clinician and the patient.
Inflammation: Increased intensity of alpha-1 and alpha-2 with a sharp leading edge of alpha-1 may be observed, but with chronic inflammation the albumin band may be decreased with increased gamma zone due to the polyclonal gammopathy.
Nephrotic syndrome: The albumin band is decreased due to hypoalbuminemia. In addition, the alpha-2 band may be more distinct.
Cirrhosis or chronic liver disease: A low albumin band due to significant hypoalbuminemia with a prominent beta-2 band and beta-gamma bridging is a characteristic feature. In addition, polyclonal hypogammaglobinemia is observed.
Malnutrition: Decreased albumin levels [ 1 ].
Alpha-1 antitrypsin deficiency : Inflammatory condition, pregnancy.
Hemolysis: Altered electrophoretic pattern of small indistinct peaks in alpha-2 region.
4.2 Quality assurance in SPE
Quality assurance in SPE is an essential prerequisite to ensure reliability of an SPE result [ 2 ]. There are two major aspects of analytical quality including precision (measure of precision) and accuracy (measure of trueness).
Good clinical laboratory practices demand processing of an internal quality control (IQC) for assessment of precision and external quality assurance (EQA)/proficiency testing (PT testing) for accuracy assessment. IQC is a material which can be prepared in house (patient sample) or available commercially and is to be processed before a patient sample is taken up for processing.
The clinical laboratory has its responsibility to select and use an IQC which has a matrix comparable to patient sample, preferably covering the clinical decision point (cut off value that differentiates between a normal and abnormal result). EQA is an external assessment of the analytical quality wherein the laboratory processes a blinded sample and the results are compared against a reference method and/or against the consensus value of other participant laboratories for that specific sample.
The laboratory has to hold responsibility in selecting a suitable EQA provider who shall preferably be accredited to ISO 17043. If an EQA program is not available, the laboratories shall participate in exchange of samples with referral laboratories with a similar methodology and a comparable quality of testing standard.
5. Reporting of results and its standardization
Reporting SPE requires interpretation of the electrophoretic pattern which is followed by comments of such an interpretation along with the piece of advice to the clinician if indicated. There is a big lacuna in the format of reporting of SPE, each laboratorian using his/her own means of interpreting and communicating. It is the need of the hour to have a standardized format of reporting SPE for ensuring patient safety and clinician follow-up. There are no international guidelines, though the working party on standardized reporting of protein electrophoresis which is an initiative of the Australasian Association of Clinical Biochemists has come out with a standardized format of reporting SPE.
6. Conclusion
In the current scenario, it becomes the responsibility of each and every laboratory to ensure that all relevant information is available in a SPE report, easily read, understood, and interpreted by a clinician. This becomes the core of a clinical laboratory practice.
Acknowledgments
We would wish to acknowledge and thank the management of MIOT hospitals for providing us with the infrastructure and technology to explore, learn, and contribute to our patients’ well-being.
We would wish to thank our technical staff, Mr. Mathivanan Durairaj, and his team for their invaluable contribution by sharing clinical cases.
We wish to acknowledge Trivitron technologies for their valuable support in installation and continual service and application support for Sebia CZE.
- 1. O’Connell TX, Horita TJ, Kasravi B. Understanding and interpreting serum protein electrophoresis. American Family Physician. 2005; 71 (1):105-112
- 2. Jenkins MA. Electrophoresis. Quality Control and Quality Assurance Aspects of the Routine use of Capillary Electrophoresis for Serum and Urine Protein in Clinical Laboratories. Wiley Online Library. Jun 2004; 25 (10-11):1555-1560
- 3. Jenkins MA. Serum Protein Electrophoresis. Clinical Applications of Capillary Electrophoresis. Molecular Biotechnology; 2000. pp. 11-19
- 4. Ninfa AJ, Ballou DP, Benore M. Fundamental Laboratory Approaches for Biochemistry and Biotechnology. Hoboken, NJ: Wiley; 2009. p. 161
- 5. Smith I, editor. Zone Electrophoresis: Chromatographic and Electrophoretic Techniques. 4th ed. Elsevier Ltd.; 1976
- 6. Tate J, Caldwell G, Daly J, Gillis D, Jenkins M, et al. Recommendations for standardized reporting of protein electrophoresis in Australia and New Zealand. Annals of Clinical Biochemistry. 2012; 49 :242-256
© 2019 The Author(s). Licensee IntechOpen. This chapter is distributed under the terms of the Creative Commons Attribution 3.0 License , which permits unrestricted use, distribution, and reproduction in any medium, provided the original work is properly cited.
Continue reading from the same book
Biochemical testing.
Edited by Varaprasad Bobbarala PhD
Published: 29 April 2020
By Husniza Hussain, Rusidah Selamat, Lim Kuang Kuay, ...
2081 downloads
By Khushaboo Pandey and Om Prakash Mishra
1197 downloads
By Nataša Gros
1313 downloads
If you're seeing this message, it means we're having trouble loading external resources on our website.
If you're behind a web filter, please make sure that the domains *.kastatic.org and *.kasandbox.org are unblocked.
To log in and use all the features of Khan Academy, please enable JavaScript in your browser.
AP®︎/College Biology
Course: ap®︎/college biology > unit 6.
- Introduction to genetic engineering
- Intro to biotechnology
- DNA cloning and recombinant DNA
- Overview: DNA cloning
- Polymerase chain reaction (PCR)
Gel electrophoresis
- DNA sequencing
- Applications of DNA technologies
- Biotechnology
Key points:
- Gel electrophoresis is a technique used to separate DNA fragments according to their size.
- DNA samples are loaded into wells (indentations) at one end of a gel, and an electric current is applied to pull them through the gel.
- DNA fragments are negatively charged, so they move towards the positive electrode. Because all DNA fragments have the same amount of charge per mass, small fragments move through the gel faster than large ones.
- When a gel is stained with a DNA-binding dye, the DNA fragments can be seen as bands , each representing a group of same-sized DNA fragments.
Introduction
What is a gel, how do dna fragments move through the gel, visualizing the dna fragments, check your understanding, explore outside of khan academy, attribution:.
- " DNA cloning - Advanced ," by CK-12 Foundation ( CC BY-NC 3.0 ).
- " Biotechnology , by OpenStax College, Biology ( CC BY 4.0 ). Download the original article for free at http://cnx.org/contents/[email protected] .
Works cited:
- Phoresis. (2011, April 14). Retrieved May 31, 2015 from Wikipedia: https://en.wikipedia.org/wiki/Phoresis .
- Reece, J. B., Taylor, M. R., Simon, E. J., and Dickey, J. L. (2012). Figure 12.13. Gel electrophoresis of DNA. In Campbell biology: Concepts & connections (7th ed., p. 243).
References:
Want to join the conversation.
- Upvote Button navigates to signup page
- Downvote Button navigates to signup page
- Flag Button navigates to signup page


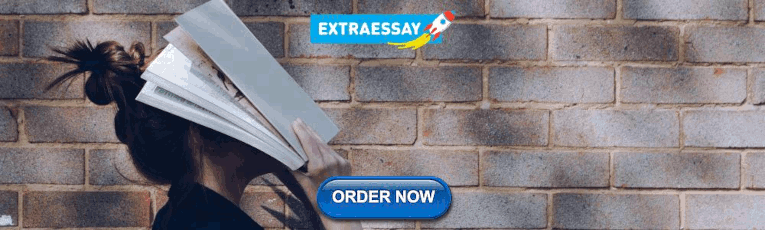
Types of Electrophoresis – Principles and Applications
Electrophoresis is a process that separates charged particles in a fluid with the aid of the field of electrical charge. In life sciences, an electrophoresis is a vital tool that separates protein molecules or DNA according to its size and type.
In a laboratory setting, electrophoresis is used to separate molecules according to heir size, purity, and density.
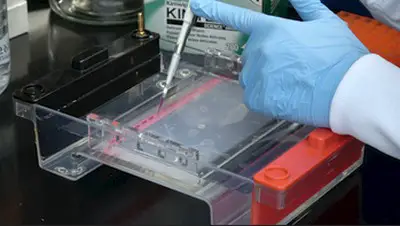
Image 1: The image above shows how an agarose gel electrophoresis is done.
Picture Source: addgene.org
There are different types of electrophoresis and the most common types are as follows:
Paper electrophoresis
It is probably the simplest type of electrophoresis wherein the sample is applied on a strip of filter paper moisturized with a buffer solution.
The end of the strip is dipped into separate tanks that have a buffer solution and different electrodes. A current is applied which will make the sample move towards the electrode with opposite polarity. The strip is dried and checked under the detection system.
Agarose gel electrophoresis
It uses an agarose gel to separate fragments of DNA or RNA of varying lengths. It observes the movement of negatively charged RNA or DNA molecules from the negative electrode to the positive ones. The molecules are differentiated according to the size of molecules.
Polyacrylamide gel electrophoresis
there are two types of gels used – dissociating and non-dissociating. The non-dissociating gel separates proteins in the original form thereby conserving the structure, function, and activity of the protein. On the other hand, the dissociating gel denatures protein into polypeptides to find out the compositionof a given sample. (1, 2, 3, and 4)
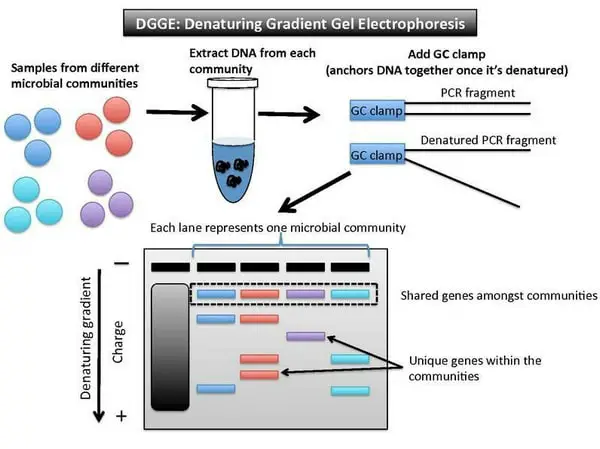
Image 2: The image above described how DGGE works.
Picture Source: pinimg.com
Denaturing gradient gel electrophoresis
also known as DGGE and temperature gradient gel electrophoresis – The former is used to separate PCA generated DNA products, which is vital in molecular fingerprinting. It is distinct from the rest because it separates PCR products according to its size difference and denaturing rate.
On the other hand, temperature gradient gel electrophoresis functions in a way like the denaturing gradient gel electrophoresis. However, its separating function is dependent on the temperature gradient. The temperature gradient enables the sample to denture based on the site mutations.
Through its use, researches can identify a single base pair mutation in a given sample (heterogeneous sample) as the denaturing point could either be lower or higher from the sample. (5, 6, 7, and 8)
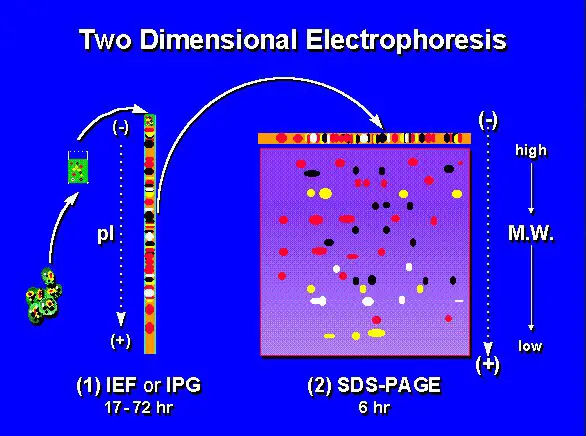
Image 3: An image representation of two-dimensional electrophoresis.
Picture Source: wikimedia.org
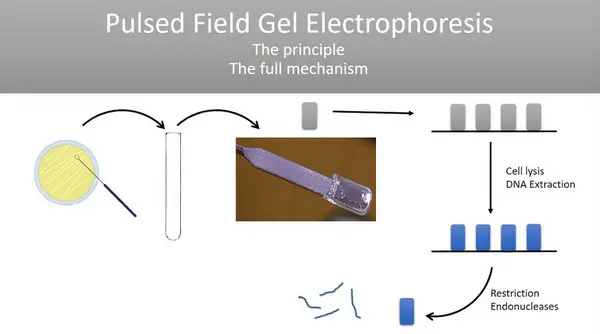
Image 4: The principle of pulse-field gel electrophoresis as shown in the image above.
Picture Source: ytimg.com
Isoelectric focusing and 2-D gel electrophoresis
The former is a method of protein separation according to net charge. A sample of protein is placed in a pH gradient slab generated by an electrical field. It resulted in the migration of protein in the pH gradient field until such time it reaches a pH with isoelectric point of zero.
On the other hand, the latter, 2-D gel electrophoresis combines SDS-PAGE and isoelectric focusing method thereby separating proteins according to their size and isoelectric point. The 2-D gel electrophoresis is the most preferred method of the two because it gives a better protein resolution. It is also useful in separating protein if the protein’s size and charge are unknown.
It is a method of electrophoresis that enables enzymatic activity to be checked in situ after an enzyme underwent electrophoresis. Through this method, researchers can characterize proteins/enzymes present on the gel without the need to purify the protein or enzyme.
Through this process, the researcher will be able to save time by not undergoing anymore the enzyme or protein purification step. Zymograms enables you to check the physical characteristics of the enzymes such as the isoelectric point and molecular weight. (3, 6, and 9)
Pulsed-field electrophoresis
The usual electrophoresis method is not applicable in very large DNA molecules, typically around 30kb to 50kb. However, pulse-field electrophoresis can withstand the examination of even large DNA molecules making it the most suitable type of electrophoresis.
Capillary electrophoresis
It uses a thin capillary tube made of either plastic, quartz, or glass. The tube is filled with the required buffer for agarose work. Capillary electrophoresis is used for analytical work such as genetic analysis, counter-ion analysis, pharmaceuticals with enantiomers, and characterization of protein.
Microchip electrophoresis
It is a more efficient type of electrophoresis because it leads to an increase in throughput by many folds over the capillary electrophoresis system because microchip system has various micro-channels enabling high throughput experiments to be done in the easiest and the most efficient way possible.
This type of electrophoresis is fully automated from the very start (handling of sample) to the end (data analysis). Since it is fully automated, the chances of human error can be reduced significantly.
Fluorophore-assisted carbohydrate electrophoresis
It helps in identifying carbohydrates with the attached fluorescent dye by means of carbohydrate separation with the use of polyacrylamide gel.
It is useful in analyzing various types of carbohydrates like glycolipids, glycoproteins, and polysaccharides both plants and bacteria.
This type of electrophoresis simplifies the process of detecting lipid-linked oligosaccharides, which might be needed in a sample to be labeled with radioactive sugar precursors before the molecules are detected.
Affinity electrophoresis
It is a type of electrophoresis wherein the resolving capability of the capillary electrophoresis is used to differentiate samples that undergo interactions of both specific and non-specific during electrophoresis.
What makes it special is its ability to detect affinity interactions be it in free or immobilized form. It can detect peptides and proteins. It is also vital in detecting small molecules, development of drugs, and for immune-affinity activities.
The sensitivity of affinity electrophoresis enables a more precise detection and discrimination of proteins, both normal and carcinogenic in a given sample.
Automated electrophoresis system
electrophoresis can now be done with the use of a computerized robotics and programming. It allows electrophoresis protocols to be automatically conducted. (2, 5, 9, and 10)
- https://www.medialab.com/electrophoresis.aspx
- https://www.labce.com/spg2145021_types_of_electrophoresis___summary.aspx
- https://www.ukessays.com/essays/biology/application-and-advancement-of-electrophoresis-in-scientific-research-biology-essay.php
- https://sciencing.com/types-electrophoresis-5569711.html
- http://www.biologydiscussion.com/biochemistry/electrochemical-techniques/top-10-types-of-electrophoretic-techniques-used-in-biochemistry/12669
- https://www.slideshare.net/SayantiSau/techniques-of-electrophoresis
- https://byjus.com/chemistry/types-of-electrophoresis/
- https://en.wikipedia.org/wiki/Electrophoresis
- https://www.sciencedirect.com/topics/earth-and-planetary-sciences/electrophoresis
- http://chem-fuuast.weebly.com/uploads/1/2/8/9/12894433/presentation_on_electrophoresis_by_dr_shah_ali-ul-qadir.pdf
Leave a Reply Cancel reply
Your email address will not be published. Required fields are marked *
Save my name, email, and website in this browser for the next time I comment.

Electrophoresis: Overview, Principles and Types
- Electrophoresis involves the migration of charged particle or molecules under the influence of an applied electric field.
- Various important biomolecules such as peptides, amino acids, proteins, nucleic acid and nucleotides has ionizable groups and they exist in solution as electrically charged particles either as cations or as anions at any given pH.
- These charged particles will move towards the cathode or to the anode end based on their net charge in a mixture under the influence of an applied electric field.
- The strength of electric field, shape and size.
- Relative hydrophobicity of the sample.
- Temperature and Ionic strength of the buffer.
- Molecular mass of the taken biomolecule.
- Net charge density of the bio molecule taken.
- Shape of the taken biomolecule.
Electrophoresis is basically of two types
- Free boundary or moving boundary electrophoresis.
- Zone electrophoresis.
Moving boundary electrophoresis
- It is a type of electrophoresis without supporting media, in a free solution.
- Tiselius developed this type of electrophoresis in 1937.
- For the separation of different charged molecules in a mixture, sample is placed in glass, which is connected to the electrodes. On applying electric potential across the tube, charged molecule migrates towards one or another electrode.
Zone electrophoresis
- It involves the separation of charged particles on inert matrix, or supporting or stabilizing media.
On the basis of supporting media, it is of following types
Paper electrophoresis, cellulose acetate electrophoresis, capillary electrophoresis.
- Gel electrophoresis.
The equipment used for the electrophoresis basically consists of two items:
An electrophoretic unit and a power pack.
Electrophoretic unit is of two types:
Vertical electrophoretic unit
Horizontal electrophoretic unit.
- It is also known as vertical slab gel units.
- It is available commercially and mostly used to separate proteins in acrylamide gels.
- In this, gel is formed between the two glass plates that are clamped together but uses plastic spacers to hold them apart.
- Dimensions of gel are typically: 8.5 cm wide X 5 cm high, Thickness = 0.51 mm.
- In this, a gel is cast on a plate, horizontally and submerged in a running buffer.
- This apparatus is mostly used to separate nucleic acid or proteins in agarose gel.
- Filter paper is very popular as a stabilizing media and most commonly used for the study of normal and normal plasma proteins.
- For electrophoresis Chromatography paper is most suitable and it needs no preparation other than to be cut to size.
- Apparatus: It consists basically of two items, a power pack and an electrophoretic cell.
- The power pack provides a stabilized direct current and has controls for both voltage and current output. Power packs, which have an output of 0-500 V and 0-150 rnA are available and can be programmed to give either constant voltage or current.
- Kohn in 1958 introduced, Cellulose acetate as a medium for electrophoresis.
- It was developed from bacteriological cellulose acetate membrane filters and is commercially available as high purity cellulose acetate strips, which are thin and have a uniform micropore structure.
- Cellulose acetate is especially used for clinical investigations such as separation of hemoglobin’s from blood, lipoproteins and glycoproteins.
- Buffers used in both the electrophoresis i.e., in paper and cellulose acetate electrophoresis are same
- It can be described as the new generation electrophoretic technique.
- An electrolyte-filled capillary, which passes through the optical center of a detector
- A sample injector
- A high voltage power supply
- an auto-sampler. The entire instrument is computer controlled.
- This technique of electrophoresis has become very popular over the years because of the many advantages that it has over conventional electrophoresis techniques.
These advantages are listed below:
- Very high-level automation is possible.
- Fast analysis times.
- Detection of separated peaks is done online; thus, detection is a process that goes hand in hand with separation and not post-separation as is the case with conventional techniques.
- Heat generated inside the capillary is effectively dissipated through the walls of the capillary; therefore, high voltages can be applied.
- High voltages mean a rapid separation.
- This faster separation along with online detection makes this technique considerably faster as compared to the conventional techniques.
Gel electrophoresis
- It is simple, rapid and sensitive analytical technique for the separation of charged particle.
- The gels, however, are porous and the size of the pores relative to that of the molecule determines whether the molecule will enter the pore and be retarded or will bypass it. The separation thus not only depends on the charge on the molecule but also on its size.
- That resolution of a sample is sharper and better in a gel than in any other type of medium.
- Gel electrophoresis uses the gel as supporting media for separation of DNA , RNA and protein under the influence of electric charge.
Basically, gel electrophoresis apparatus is of two kinds:
- Vertical gel apparatus : It is commonly used for the separation of proteins in SDS-PAGE.
- Horizontal gel apparatus : It is used for immune electrophoresis, iso-electric focusing and electrophoresis of DNA and RNA in the agarose gel.
Type of gel electrophoresis:
Agarose gel electrophoresis.
- Pulse field gel electrophoresis (PFGE)
2D gel electrophoresis
- The supporting media in this type of electrophoresis is agarose gel.
- It is routinely used for the electrophoresis of nucleic acid like DNA and RNA.
When potential difference is applied across the electrodes in a horizontal electrophoretic tank containing agarose gel and biomolecules (such as nucleic acid) is loaded, then molecules separated accordingly by their molecular size and move to their respective electrodes which is tracked with the help of dye.
Chemical component such as:
- Media : agarose gel
- Buffer : TAE/TBE (tris acetate EDTA/ Tris borate EDTA).
SDS-PAGE Electrophoresis
- Sodium dodecyl sulfate (SDS) polyacrylamide gel electrophoresis is mainly used for the separation of proteins based on their mass.
- Principle: This technique uses anionic detergent sodium dodecyl sulfate (SDS) which disassociates proteins into their individual polypeptide subunits and gives a uniform negative charge along each denatured polypeptide. When these denatured polypeptides are loaded at the cathode end of an electric field, then we get clear bands of proteins arranged in decreasing order of their molecular mass from the cathode to anode.
- To separate proteins it involves the use of vertical gel apparatus.
Chemical component
- Media : SDS-PAGE
- Buffer : lower reservoir: amine buffer with HCl (running gel), Upper reservoir: amine buffer with glycine (stack gel)
- Stains or dye : Coomassie brilliant blue R-250 (CBB) – anionic dye.
Pulse field gel electrophoresis
- It is using to separate large DNA molecule by applying gel matrix as electric field that periodically changes direction.
- It is type of gel electrophoresis, which is generally using to analyze proteins.
Reference and Sources
- https://www.biotechnologynotes.com/electrophoresis/electrophoresis-meaning-definition-and-classification-withdiagram/293
- https://www.slideshare.net/AmruthaHari1/electrophoresispaper-electrophoresisgel-elctrophoresis-pagesds-andnonsds
- https://www.onlinebiologynotes.com/electrophoresis-principle-affecting-factors-and-types/
- ttps://biocyclopedia.com/index/biotechnology_methods/electrophoresis/electrophoresis01.php
- https://www.sciencedirect.com/topics/pharmacology-toxicology-and-pharmaceutical-science/electrophoresis
- https://www.slideshare.net/DipeshTamrakar2/electrophoresis-79640318
- https://en.wikipedia.org/wiki/Pulsed-field_gel_electrophoresis
- http://lmxq.cper.revitradio.co/zone_electrophoresis_ppt.pdf
- Southern Blotting
- Ethanol : production, recovery and uses
- Types of microscopes
- Chromatography: Introduction, Principle, Classification and applications
- Microscopy: Overview, Principles and Its Types
1 thought on “Electrophoresis: Overview, Principles and Types”
Useful. Thank u 😇
Leave a Comment Cancel reply
Save my name, email, and website in this browser for the next time I comment.
- Introduction to Genomics
- Educational Resources
- Policy Issues in Genomics
- The Human Genome Project
- Funding Opportunities
- Funded Programs & Projects
- Division and Program Directors
- Scientific Program Analysts
- Contact by Research Area
- News & Events
- Research Areas
- Research investigators
- Research Projects
- Clinical Research
- Data Tools & Resources
- Genomics & Medicine
- Family Health History
- For Patients & Families
- For Health Professionals
- Jobs at NHGRI
- Training at NHGRI
- Funding for Research Training
- Professional Development Programs
- NHGRI Culture
- Social Media
- Broadcast Media
- Image Gallery
- Press Resources
- Organization
- NHGRI Director
- Mission & Vision
- Policies & Guidance
- Institute Advisors
- Strategic Vision
- Leadership Initiatives
- Diversity, Equity, and Inclusion
- Partner with NHGRI
- Staff Search
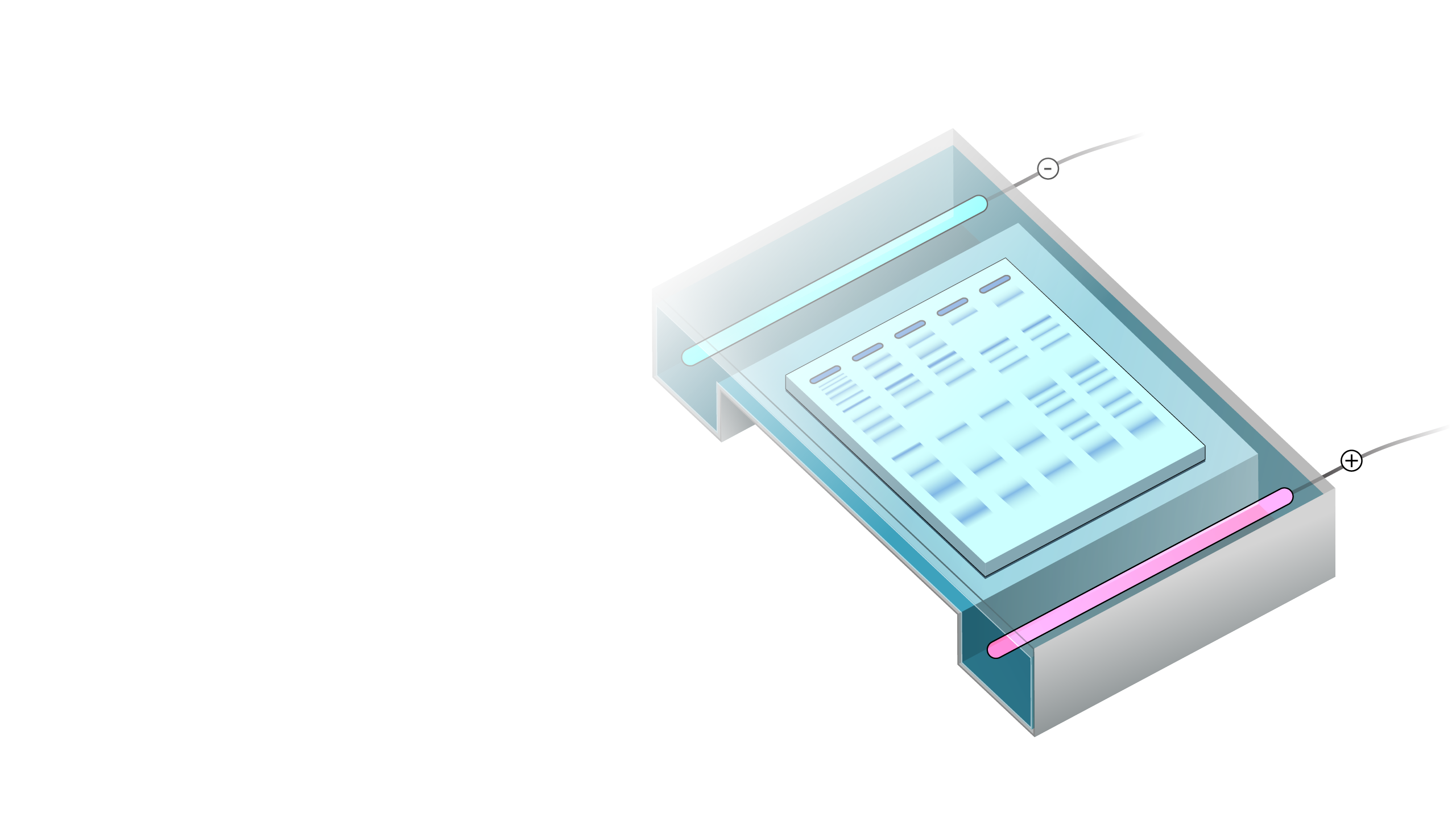
Electrophoresis
Electrophoresis is a laboratory technique used to separate DNA, RNA or protein molecules based on their size and electrical charge. An electric current is used to move the molecules through a gel or other matrix. Pores in the gel or matrix work like a sieve, allowing smaller molecules to move faster than larger molecules. To determine the size of the molecules in a sample, standards of known sizes are separated on the same gel and then compared to the sample.
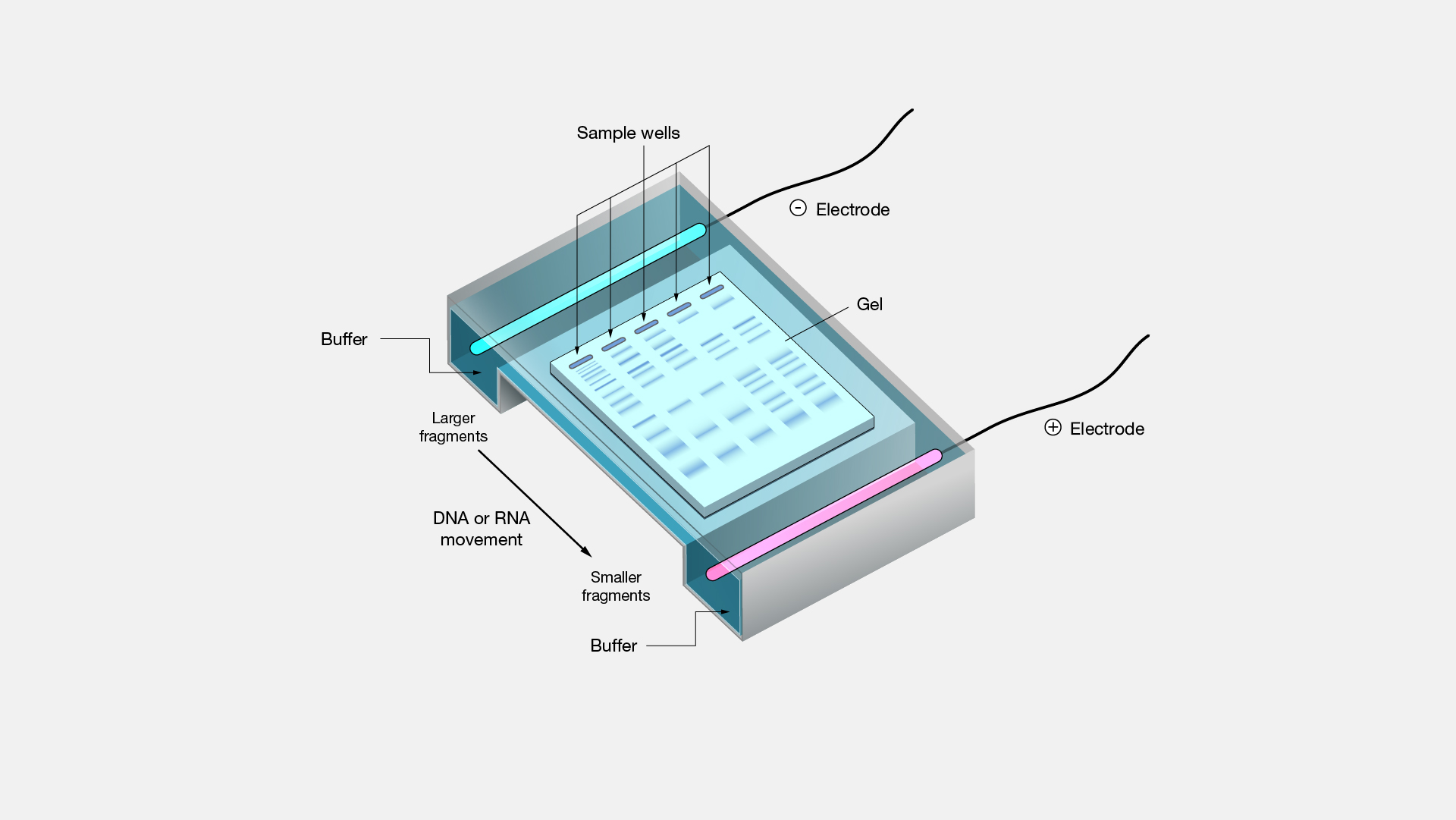
Electrophoresis. I worked in my dad's lab in late high school in college where we use starched electrophoresis to study population genetics. I remember first learning of and then carefully reading the paper where Linnaeus Pauling and colleagues reported using the new technology of electrophoresis in 1946, to show that sickle cell hemoglobin carries more positive charges than the normal version on its surface. Today, electrophoresis is used for so much more across biology and medicine.
Former Program Director, Genome Technology Program
Division of Genome Sciences
Electrophoresis Definition and Explanation
What Electrophoresis Is and How It Works
- Cell Biology
- Weather & Climate
- Ph.D., Biomedical Sciences, University of Tennessee at Knoxville
- B.A., Physics and Mathematics, Hastings College
Electrophoresis is the term used to describe the motion of particles in a gel or fluid within a relatively uniform electric field. Electrophoresis may be used to separate molecules based on charge, size, and binding affinity. The technique is mainly applied to separate and analyze biomolecules, such as DNA , RNA, proteins, nucleic acid s, plasmids, and fragments of these macromolecules . Electrophoresis is one of the techniques used to identify source DNA, as in paternity testing and forensic science.
Electrophoresis of anions or negatively charged particles is called anaphoresis . Electrophoresis of cations or positively charged particles is called cataphoresis .
Electrophoresis was first observed in 1807 by Ferdinand Frederic Reuss of Moscow State University, who noticed clay particles migrated in water subjected to a continuous electric field.
Key Takeaways: Electrophoresis
- Electrophoresis is a technique used to separate molecules in a gel or fluid using an electric field.
- The rate and direction of particle movement in the electric field depends on the molecule's size and electric charge.
- Usually electrophoresis is used to separate macromolecules, such as DNA, RNA, or proteins.
How Electrophoresis Works
In electrophoresis, there are two primary factors that control how quickly a particle can move and in what direction. First, the charge on the sample matters. Negatively charged species are attracted to the positive pole of an electric field, while positively charged species are attracted to the negative end. A neutral species may be ionized if the field is strong enough. Otherwise, it doesn't tend to be affected.
The other factor is particle size. Small ions and molecules can move through a gel or liquid much more quickly than larger ones.
While a charged particle is attracted to an opposite charge in an electric field, there are other forces that affect how a molecule moves. Friction and the electrostatic retardation force slow the progress of particles through the fluid or gel. In the case of gel electrophoresis, the concentration of the gel can be controlled to determine the pore size of the gel matrix, which influences mobility. A liquid buffer is also present, which controls the pH of the environment.
As molecules are pulled through a liquid or gel, the medium heats up. This can denature the molecules as well as affect the rate of movement. The voltage is controlled to try to minimize the time required to separate molecules, while maintaining a good separation and keeping the chemical species intact. Sometimes electrophoresis is performed in a refrigerator to help compensate for the heat.
Types of Electrophoresis
Electrophoresis encompasses several related analytical techniques. Examples include:
- affinity electrophoresis - Affinity electrophoresis is a type of electrophoresis in which particles are separated based on complex formation or biospecific interaction
- capillary electrophoresis - Capillary electrophoresis is a type of electrophoresis used to separate ions depending mainly on the atomic radius, charge, and viscosity. As the name suggests, this technique is commonly performed in a glass tube. It yields quick results and a high resolution separation.
- gel electrophoresis - Gel electrophoresis is a widely used type of electrophoresis in which molecules are separated by movement through a porous gel under the influence of an electrical field. The two main gel materials are agarose and polyacrylamide. Gel electrophoresis is used to separate nucleic acids (DNA and RNA), nucleic acid fragments, and proteins.
- immunoelectrophoresis - Immunoelectrophoresis is the general name given to a variety of electrophoretic techniques used to characterize and separate proteins based on their reaction to antibodies.
- electroblotting - Electroblotting is a technique used to recover nucleic acids or proteins following electrophoresis by transferring them onto a membrane. The polymers polyvinylidene fluoride (PVDF) or nitrocellulose are commonly used. Once the specimen has been recovered, it can be further analyzed using stains or probes. A western blot is one form of electroblotting used to detect specific proteins using artificial antibodies.
- pulsed-field gel electrophoresis - Pulsed-field electrophoresis is used to separate macromolecules, such as DNA, by periodically changing the direction of the electric field applied to a gel matrix. The reason the electric field is changed is because traditional gel electrophoresis is unable to efficiently separate very large molecules that all tend to migrate together. Changing the direction of the electric field gives the molecules additional directions to travel, so they have a path through the gel. The voltage is generally switched between three directions: one running along the axis of the gel and two at 60 degrees to either side. Although the process takes longer than traditional gel electrophoresis, it's better at separating large pieces of DNA.
- isoelectric focusing - Isoelectric focusing (IEF or electrofocusing) is a form of electrophoresis that separates molecules based on different isoelectric points. IEF is most often performed on proteins because their electrical charge depends on pH.
- How Does Electrical Energy Work?
- Understanding the Double-Helix Structure of DNA
- Learn About Nucleic Acids and Their Function
- The Role of Cytoplasm in a Cell
- Voltage Definition in Physics
- Hydrogen Bond Definition and Examples
- An Introduction to Brownian Motion
- Cathode Ray History
- Electron Definition: Chemistry Glossary
- Gas Definition and Examples in Chemistry
- DNA Replication Steps and Process
- Understanding Electrical, Thermal, and Sound Conductors
- Frequently Asked Biology Questions and Answers
- 10 RNA Facts
- How To Extract DNA From a Banana
- What a Lightning Strike Does to Your Body

An official website of the United States government
The .gov means it’s official. Federal government websites often end in .gov or .mil. Before sharing sensitive information, make sure you’re on a federal government site.
The site is secure. The https:// ensures that you are connecting to the official website and that any information you provide is encrypted and transmitted securely.
- Publications
- Account settings
Preview improvements coming to the PMC website in October 2024. Learn More or Try it out now .
- Advanced Search
- Journal List
- Wiley-Blackwell Online Open

The Electrophoretic Revolution in the 1960s: Historical Epistemology Meets the Global History of Science and Technology **
Edna suárez‐díaz.
1 Facultad de Ciencias, Universidad Nacional Autónoma de México (UNAM)
This paper uses zone electrophoresis , one of the most frequently used tools in molecular biology, to explore two ideas derived from Hans‐Jörg Rheinberger's reflections on experiments. First, the constraining role played by technical objects—instrumentation and material conditions—in the production of knowledge or epistemic things. Second, the production of interconnected experimental systems by such technical objects, which results in the unexpected entanglement of research fields and experimental cultures. By the beginning of the 1960s, the inception of zone electrophoresis in laboratories around the world transformed—some say, revolutionized—the study of proteins. Even today, electrophoresis continues to open research venues and questions in biomedicine, molecular biology, human genetics, and in the field of molecular evolution. In my essay, I seek to look at the interconnected lives of zone electrophoresis and address the broader social, and even global context, in which this apparently humble technique became a salient tool in the production of biological knowledge. In so doing, I aim to take the past and present of the history and historiography of experimental systems to the future, where experiments and technologies are interrogated as they are used in different geographies and contexts, including contexts of poverty.
1. Introduction
In 1960, after returning from his graduate studies in Chicago, medical geneticist Rubén Lisker (1931–2015) received a grant of 1,000 Mexican pesos (amounting to less than 100 US dollars) to start a precarious research project on blood types and abnormal hemoglobin in indigenous populations. He also received a small amount from the Mexican Secretaría de Salud (Health Ministry) and the local Carnot Laboratories, 1 but the meager funds did not stop him from pursuing the kind of edgy research that he had become acquainted with at the Michael Reese Hospital in Chicago, Illinois. With the help of a friend, engineer Mauricio Russek‐Bernal from the Instituto Politécnico Nacional ( IPN ), he used part of the money to build a small apparatus of paper electrophoresis, one of the new options to perform the decades old technique of separating macromolecules (proteins) by their electric properties and size. 2 They bought a pair of glass plates, which they then adapted to create an electric power supply with a couple of platinum electrodes and forceps to hold everything together. With this improvised instrument, Lisker managed to perform the first electrophoretic studies of genetic variation in Mexican populations. His primary goal was to identify the same abnormal hemoglobin he had worked with in Chicago, sickle‐cell hemoglobin or HbS for short, as well as the presence of glucose‐6‐phosphate dehydrogenase (G6PD) deficiency, a scarcely understood but prevalent mutation in African and Mediterranean populations. A few studies had been performed before on abnormal hemoglobin in México by Adolfo Karl at the IPN , which had not been successful. Nevertheless, in collaboration with long‐time partner Alvar Loria, also at the Hematology Department of the Instituto Nacional de la Nutrición , Lisker soon published the first of several papers—nineteen to be exact—on the genetic variation of local populations, reporting several genetic variants in blood proteins. Though he continued to use serological tests and blood‐typing surveys, his first report of a G6PD anomaly used electrophoresis to describe the clinical case of a Sephardic boy, whose family had immigrated from Turkey to Mexico. Indeed, the most common G6PD anomaly, favism, was a disease long associated with Mediterranean and Middle Eastern populations who have fava beans as a common ingredient in local diets. 3 Later on, using the technical variant of starch gel electrophoresis, Lisker also reported the presence of abnormal hemoglobins—including a Chiapas variant—in Mexico's indigenous communities. Lisker's research on blood diseases, which continued until the late 1960s and resumed using new technologies in the 1990s, remains to date one of the most comprehensive studies of the genetics of Mexican populations. 4
Zone electrophoresis, one of the most frequently used tools in molecular biology laboratories, provides a lens to explore two ideas derived from Hans‐Jörg Rheinberger's extended account of experiments: first, the constraining role played by technical objects—instrumentation and material conditions—in the production of knowledge; and second, the production of interconnected experimental systems by such technical objects, which results in the unexpected entanglement of research fields and experimental cultures. To do so, this essay presents a historical epistemological analysis combined with an original way to see the role of electrophoresis in connecting experimental systems and cultures. I am talking about studies on the globalization of technologies, and the epistemic spaces opened by this type of inquiry. Writing the history of the uses of a technology in its different contexts, including contexts of poverty, is a much‐needed task for historians of technology and global history, as David Edgerton has forcefully argued. 5 To shift the focus to use requires us to set aside the idea that technological invention and innovation only take place in a privileged setting and pay attention to the adaptations, applications, and resourcefulness required by the actual historical uses of a technology as it travels beyond its place of origin
As the history of studies on blood proteins shows, knowledge on the mechanisms of inheritance of these variations, the establishment of new molecular variants, and empirical data to feed the theoretical models happened far from the usual centers of knowledge production—Bogota, Mexico City, Baghdad, or Tel‐Aviv. These varied experiments cannot be seen as repetitions of ones performed in metropolitan centers. As part of experimental systems set up in different locations, they were able to generate unexpected results and authentic surprises. 6 Moreover, I seek to look beyond the innovation of zone electrophoresis in protein research laboratories in the United States and Western Europe, opting to address the broader social, and even global context, in which this once sophisticated but now apparently humble technique became a salient tool in the production of knowledge. Zone electrophoresis thus can be seen as a material node that tied together the experimental cultures of protein chemistry, human genetics, biomedicine, and evolutionary biology, but also incorporated networks of scientists worldwide, as molecular biology struggled to show its relevance for understanding disease and global medical interventions not just in industrialized countries but as part of the developmentalist agenda. In paying attention to these locations, I aim to engage with the present and future of the history and historiography of the life sciences, where historical epistemology and the global history of science and technology can fruitfully complement each other.
2. Electrophoresis after the Second World War
The clear theoretical implications for physical chemistry and extensive biomedical and military applications during and after WWII are exemplified in Angela Creager's essays on Edwin Cohn's research. 7 The solution‐based or moving‐boundary method of the Tiselius apparatus developed in the 1930s and 1940s, however, was not only difficult to operate and voluminous, but also expensive and beyond the capacities of most laboratories around the world. 8 By contrast, the electrophoresis apparatus that today inhabits any molecular biology or biochemistry lab is a product of the postwar industrialization of biomedical research. In the decades following the war, the industrial manufacture of research instruments expanded massively in the continued efforts to produce functional, compact, and affordable instruments that would meet the specific needs of the growing community of researchers across the globe. 9
Historian of science Lily Kay recounts that by the end of the war in 1945, Klett Industries debuted a compact model of the costly Tiselius apparatus, the antecedent of modern zone electrophoresis. Costing 4,000 USD (compared to the 6,000 USD price tag of the original apparatus). 10 Perhaps more important than its reduced price, was the fact that this new device did not require the intensive work (and salary) of a specialized technician to operate it (about 5,000 USD per year). 11 By 1950, at least four different bench models existed that were manufactured and commercialized by Perkin‐Elmer at the lower, but still far from universally affordable, cost of 3,000 USD, approximately 35,000 USD in today's dollars. 12 These new instruments saved precious laboratory space and required only a one‐week training course before they could be put to use. With the arrival of radioisotopes and scintillation counters in the 1950s, electrophoresis became a relatively accessible though still sophisticated instrument available in laboratories in the United States and Europe.
The pervasiveness of electrophoresis in experimental biology hit its highest peak, however, during the next decade. At the beginning of the 1960s, the development of zone electrophoresis, also called molecular sieving electrophoresis because of its ability to segregate protein molecules in a sustaining material, radically altered the availability of the method. Contemporary scientists and historians alike, have labeled the introduction of the new technology a “revolution” in the study of proteins. 13 Historian of science and medicine Howard Chiang has reconstructed the consistent search for a sustaining medium that would allow the easy and complete molecular separation and visualization of proteins using the principles of electrophoresis. Silicon and agar gels, filter paper, pectin gel, cellulose acetate, and starch gel were tested, used, and eventually discarded, until the choice of polyacrylamide gel standardized the material universally present in today's laboratories. However, as Chiang argues, this was not a simple test and error enterprise: it involved efforts and knowledge from scientists in different fields of research, including physical chemistry, immunology, and biochemistry, all of which had something to say about the properties and behavior of proteins. 14
The establishment of starch gel and polyacrylamide gel electrophoresis, at the end of the 1950s and the beginning of the 1960s, made possible the explosion of applications beyond medicine and biochemistry, in the closely related field of population genetics. Here, the study of human evolution and migration, and of the effects of atomic testing on genetic mutations, converged on the attention to “primitive populations” in contemporary parlance. 15 Population genetics was, indeed, a hot field during those years, linking the study of disease and evolution as two faces of the same quest: the study of genetic variations, and their measurement made possible by zone electrophoresis. In 1966, Harry Harris, Galton Professor of Human Genetics at King's College, published one of three papers considered crucial to contemporary theoretical debates in population genetics, at a time when Cold War anxieties surrounding atomic weapons testing could not be higher. 16 In parallel, for countries in the so‐called Third World and for segregated African‐American populations in the Southern United States, electrophoresis enabled the assessment of genetic abnormalities in blood proteins, and became instrumental for those promoting the molecular approach to disease, encapsulated in the concept of “molecular disease.” 17 The study of “blood diseases in the backyard,” as I have previously referred to this kind of research, linked several of those contemporary preoccupations.
Ruben Lisker, like many other geneticists and medical anthropologists around the world, participated in the international and regional networks organized by the World Health Organization and the Pan American Health Organization round those interrelated topics. They were part of scientific committees created to produce global knowledge and international health policies in the twin fields of human genetic variation and the molecular understanding of medical conditions; at the same time these studies were hailed as an early example of the uses of pharmacogenetics. 18 Blood diseases, including sickle cell anemia, different types of thalassemia, and G6PD deficiencies (one of which is related to favism), targeted populations in Africa, the Mediterranean, East Asia and Latin America, thus becoming a de facto biomedical enterprise alternative to the war on cancer. The extensive network of human geneticists surveying molecular abnormalities, most of them using starch gel electrophoresis, has caught the attention of historians of science, though it deserves to be further explored. 19
3. The Interconnected Lives of Electrophoretic Experiments
In contrast to other big manufacturers of research equipment, 20 the main manufacturer of electrophoresis equipment and consumables in today's laboratories is a US‐based family firm, Bio‐Rad Laboratories, Inc. The company was founded in 1952 in Berkeley, California, and entered the blooming experimental culture of identification, separation, purification, and analysis of chemical and biological materials. Bio‐Rad first made a name for itself in the field of analytical chemistry through ion exchange resins. In 1964, the firm introduced “gel chromatography media […] for separating proteins by molecular weight,” thus saving preparation time for scientists. 21 The company has created an empire in the manufacturing of equipment for life science research and medical diagnostics, and in the last decades has additionally absorbed other industries in the agricultural, chemistry, and biomedical fields. Even today, electrophoresis continues to open research venues and solve relevant questions in biomedicine, molecular biology, human genetics, and molecular evolution. Bio‐Rad equipment is found in laboratories around the globe (Figure 1 ), most probably because the technique provides one of the easiest procedures to visualize the properties of proteins and nucleic acids. The interaction of the mass and electric properties of a macromolecule with the buffer and the supporting material in an electric field is manifested in the characteristic bands—“traces,” in Rheinberger's conceptualization. These traces constitute the elementary material manifestation preceding a scientific representation. 22 Those traces, however, only become data in their comparison with one another; it is only in the comparative alignment of bands that anomalies (variations) can be incorporated in the mathematical or in the mechanistic models of theoretical population genetics, protein chemistry, genetics, and biomedicine.

Bio‐Rad electrophoresis apparatus and power supply at the author's brother laboratory of plant molecular biology in Mexico City, 2022. Photograph taken by the author's brother, Javier Andrés Juárez‐Díaz.
What is, precisely, the place of electrophoretic apparatuses and methods in those different but connected research fields? The technological intervention exerted by electrophoresis on blood materials results in traces that take on one of two roles depending on the specific experimental culture: measuring , as in measuring the molecular weight or the electric valance of a protein, or identifying biological phenomena , as in providing the trace for an abnormal or variant protein. An electrophoresis experiment also provides a more complex type of measurement, or calculation, as it delivers the traces used to infer the amount of genetic variation within a population. In these three different cases, electrophoresis participates in fields of research as different as protein chemistry, molecular biology and biomedicine, and theoretical population genetics, respectively. The epistemic role it plays is also different: identifying biological phenomena—like an abnormal hemoglobin—is a classic case of the laboratory sciences, while providing measures of genetic variation, according to Richard Lewontin, was required to meet the chronic lack of empirical data “to feed the theoretical machine” of mathematical population genetics. 23 This latter role is close to the traditional view of the place of experiments in the philosophy of science, namely, providing empirical evidence to test a hypothesis. At the same time, the history of the contentious debate on the amount of genetic variation in natural populations that followed from these experiments, gives credence to sociological accounts on the difficulties of experimental closure. In 1966, electrophoresis was supposed to revolutionize the whole field of human genetics, but in fact the polemic results only amplified the debate and ended up challenging the very roots of evolutionary theory. 24 This case shows, nevertheless, the fundamental role the seemingly humble technology of electrophoresis played in linking protein chemistry with the preoccupations of biomedicine and human genetics, as it had been promised and promoted by the protagonists in these developments.
4. Concluding Remarks
After the war, electrophoretic apparatuses benefited from the industrialization and commercialization of research instruments, but also from its low relative cost, ease of use, compact size, and mobility—though one should not take for granted the availability of constant electric power supply in many areas of the world in the 1960s and even today! Zone electrophoresis became an affordable and relatively mobile technology. New industries like Bio‐Rad, Perkin‐Elmer (once the parent company of Applied‐Biosystems), and many others, were created, fused, dismantled, or remained relevant and present in today's laboratories, literally around the world. The study of the design, marketing, and distribution of these technologies internationally is by itself a topic for global historians of technology, not reducible to questions of translation or communication, much less to the metaphor of circulation. 25
For historians of science, and in particular the life sciences, the topic of the global interconnection of experiments is a necessary extension of the practice turn of the 1990s and of the conceptualization of experimental systems as the material conditions that enable the generation of knowledge. Indeed, experiments have never been the same for historians and philosophers of biology—especially for those interested in molecular biology—after Rheinberger's early 1990s publications on the historiality of experimental systems. 26 “Experiment, Difference, and Writing (I and II),” published back‐to‐back in 1992, and followed by Toward a History of Epistemic Things 27 were particularly influential and opened a host of debates concerning, among other topics, the indeterminacy of experimental systems, technological determinism, and the place of the broader historical context in Rheinberger's microscopic analyses of experimental practices. Among the many themes introduced in his tireless academic trajectory, the dissection of experimental systems has stood the test of time, and his ideas have been surgically analyzed, transformed, and entangled with novel areas of research in the history and philosophy of science and historical epistemology. Most recently, Rheinberger has extended his fine‐grained dissection of experiments and experimental systems in his book Spalt und Fuge. Eine Phänomenologie des Experiments . 28 Rheinberger's argument aimed, in his words, “to avoid some of the pitfalls of social constructivism with respect to the objects of science,” by placing “the genesis and development of scientific facts […] in the relation between objects themselves.” 29
The same argument is valid in addressing the globalization of knowledge production, a process that cannot be reduced to the mere recognition of the asymmetrical relations between scientific centers, or to the extension of colonial or postcolonial exchanges. During the 1960s, a period also known as the “decade of development,” electrophoresis became one of the most popular and used technologies to survey so‐called primitive populations. This included the study of groups of people resisting and participating in liberation wars, as was the case when the US Army studied the genetics of the Vietnamese populations for malaria resistance and other biomedical conditions of interest for the effectiveness of the military enterprise. 30 Moreover, grounding of the production of knowledge in a global context directs us to recognize the epistemic injustice involved in these transactions, a concept developed in feminist philosophy to account for the downgraded status of an epistemic subject. 31 In this case, the contributions of scientists in regions outside Western Europe and the United States have been discriminated and marginalized, and wrongly perceived as epistemically lesser despite their valuable contributions to knowledge of human genetics. The globalization of zone electrophoresis and its many uses across disciplinary borders and geopolitical lines, remains a fabulous task for those interested in the broader meaning and implications of the interconnected lives of experiments.
E. Suárez-Díaz, Ber. Wissenschaftsgesch. 2022 , 45 , 332. [ PMC free article ] [ PubMed ]
** This paper is part of a special issue, On Epistemic Times: Writing History 25 Years after Synthesizing Proteins in the Test Tube , and was presented at the authors’ workshop “History and Historiography of the Life Sciences: Traces of Hans‐Jörg Rheinberger,” Max Planck Institute for the History of Science, Berlin, 7–8 April 2022. I want to thank Lara Keuck and Kärin Nickelsen for inviting me to this thoroughly planned workshop around the past, present, and future of the history of the life sciences. A special mention is deserved to Hans‐Jörg Rheinberger's enduring intellectual and personal generosity. This research is part of the Wellcome Trust Collaborative Award grant number 221321_Z_20_Z.
n1 Created in 1941, in Mexico City, Laboratorios Carnot was one of the few pharmaceutical laboratories in Mexico committed to scientific research and technological innovation in Mexico. In 1965, Lisker was awarded the Carnot Prize by the National Academy of Medicine for his surveys of indigenous populations.
n2 Kay 1988.
n3 On the relevance of favism in the study of human population genetics in the Middle East, see Burton 2021, chapter 5. Lisker, like many of the geneticists studied by Elise K. Burton, was also an Ashkenazi Jew whose family had migrated from Russia to the United States and ended up in Mexico.
n4 In his long career, Lisker worked also on lactose‐deficiency in Mexican populations and was an early researcher of bioethics. See Suárez‐Díaz 2014 and Suárez‐Díaz 2017.
n5 Edgerton 2010 and Edgerton 2007. See also Schäfer and Valeriani 2021.
n6 Rheinberger 1997.
n7 Creager 1998 and Creager 1999.
n8 Kay 1998, on 51, 67, and 69–70.
n9 The production of laboratory technologies and the specific tasks of the scientists also opened the door to new experimental designs. The close interaction between manufacturers and scientists is recounted in detail in Rheinberger's account of the history of the liquid scintillating counter. Rheinberger 2001. Also, see Alexander von Schwerin's article in this volume.
n10 Kay 1988, on 69.
n11 Ibid., on 51.
n12 Ibid., on 69.
n13 Gottlieb 1971 and Vesterberg 1989 offer personal accounts. Dietrich 2006 also calls attention to the revolutionary effect of electrophoresis in population genetics.
n14 Chiang 2009.
n15 See, for instance, World Health Organization 1964. There is ample historical literature on the subject, including: Ventura‐Santos et al. 2014; Radin 2017; Suárez‐Díaz 2019.
n16 Harris 1966. In 1965, Harris was appointed Galton Professor of Human Genetics and head of the genetics and biometry department at University College in London. From 1961–1976, Harris also directed the human biomedical genetics unit of the Medical Research Council. The other two papers are: Hubby and Lewontin 1966; Johnson et al. 1966. On genetics and atomic testing, see: Beatty 1987; Lindee 2008; de Chadarevian 2006; Hamblin 2007. For the impact of these experiments on evolutionary studies, see: Dietrich 1994; Suárez‐Díaz and Barahona 1996.
n17 Wailoo 1999, on 154–159; Wailoo 2015; Suárez‐Díaz 2017. On the study of blood anomalies by Middle‐Eastern geneticists, see Burton 2018 and Burton 2021.
n18 For instance: World Health Organization 1966; Pan American Health Organization 1968.
n19 De Souza and Ventura Santos 2014; Suárez‐Díaz 2014; Suárez‐Díaz 2017; Burton 2018. An important addition to this literature is Burton 2021.
n20 See von Schwerin's article on the early history of Applied Biosystems in this volume.
n21 Bio‐Rad, “Our History,” online: https://www.bio‐rad.com/en‐de/corporate/our‐history?ID=MR8ISY4VY (accessed 17 April 2022).
n22 Rheinberger 2021, chapters 1 and 3.
n23 Lewontin, 1974.
n24 Lewontin 1991; Dietrich 1994; Suárez‐Diaz and Barahona 1996.
n25 Mateos and Suárez‐Díaz 2019.
n26 Rheinberger 1992a; Rheinberger 1992b.
n27 Rheinberger 1997.
n28 Rheinberger 2021.
n29 Rheinberger 2005, on 408. Rather contradictorily, Rheinberger has been accused of both technological determinism and idealism. See Bloor 2005.
n30 Frischer et al. 1973. I thank Elise K. Burton for providing me this reference in relation to our common interest on James E. Bowman's work around the world.
n31 I thank Lara Keuck for her referral to the original literature on this important concept. See Fricker 2007 and Fricker 2017. More recently, the concept of epistemic injustice has been mobilized and debated in the context of international public health and the COVID‐19 pandemics, see Bhakuni and Abimbola 2021.

Capillary Electrophoresis: Principle and Application
Capillary electrophoresis is a liquid-based separation technique that uses a capillary as a separation channel under the influence of an electric field. It is an analytical technique where the electroosmotic flow helps separately charged ions. It is applicable in various areas like analysis of chemical substances, DNA analysis, identifying specific proteins, and separating coenzymes.
Table of Contents
Principle of Capillary Electrophoresis
The velocity of the analyte when migrating under the influence of an electric field of intensity “E” is given by the analyte’s electrophoretic mobility and the buffer’s electroosmotic mobility inside the capillary.
The electrophoretic mobility of solute depends on the different characteristics of the solute, like electric charge, molecular size, and shapes, and the properties of the buffer, like electrolyte’s ionic strength, pH, viscosity, and additives. The following equation gives the electrophoretic velocity (Vep) of the solute:
Vep= μepE = (q/6πηr) (V/L) where η is the viscosity of the electrolyte solution, V is the applied voltage, L= the length of the capillary, r is the Stoke’s radius of the solute, μep is the electrophoretic mobility of the solute, and q is the effective charge of the solute.
When applied through the capillary filled with the buffer, the electric field generates a solvent flow called the electroosmotic flow inside the capillary. The velocity of which depends on the electrophoretic mobility. Electrophoretic mobility depends on the charge density of the capillary’s internal wall and the buffer’s properties. The following equation gives the electroosmotic velocity (Veo):
Veo = μeoE = (ε𝜁/η) (V/L) where ε= buffer’s dielectric constant, 𝜁= zeta potential of the capillary surface, η is the viscosity of the electrolyte solution, V is the applied voltage, μeo is the electrophoretic mobility, and L is the length of the capillary.
The solute’s velocity (V) is given by: V= Vep+Veo.
The electroosmotic and electrophoretic mobility of the analyte can act in the same or opposite direction based on the solute’s charge. In the case of normal capillary electrophoresis, anions will move in the opposite direction of electroosmotic flow with velocities smaller than the electroosmotic velocity. Whereas, the cations will migrate in the same direction of the electroosmotic flow with velocities higher than the electroosmotic velocity. In conditions with a fast electroosmotic rate compared to the electrophoretic rate of the solutes, the separation of both cations and anions occurs in the same run. The time is taken (t) for the solute to migrate to the detection point from the injection end of the capillary(effective capillary length) is given by:
t = l/ Vep+Veo = l(L)/ V( Vep+Veo)

Generally, uncoated fused silica capillaries above pH three that have a negative charge are used where the electroosmotic flow occurs from the anode to the cathode. The electroosmotic flow must be constant for each run for good reproducibility in the migration velocity of the solutes. For some experiments, reduction or suppression of electroosmotic glow by changing the inner wall of the capillary, the concentration, composition, or pH of the buffer solution might be necessary.
After adding the sample, an independent zone of each analyte ion of the sample forms. The zone formation is due to the migration within the background electrolyte. The spreading of each solute band occurs as a result of different phenomena. Under ideal conditions, the only phenomenon contributing to the zone’s broadening is molecular diffusion of the solute inside the capillary. Here, the efficiency of the zone is given as the number of theoretical plates (N), which is given by:
N= (μep+μeo) (Vl)/ 2DL, where D is the molecular diffusion coefficient of the solute buffer.
In general practice, other phenomena like the length of the injection plug, detector cell size, unleveled buffer reservoirs, mismatched conductivity between sample and buffer, sample adsorption onto the capillary wall, and heat dissipation, play a significant role in band dispersion. The separation between two bands is gained by modifying the analytes’ electrophoretic mobility, the electroosmotic mobility induced in the capillary, and increasing each analyte’s efficiency for the band as follows;
Rs= N(μepb-μepa)/ 4(μaep+μeo); where μepa and μepb= the two analytes’ electrophoretic mobilities, μaep is the average electrophoretic mobility of the two analytes calculated by: μaep= ½ ( μepb+μepa).
Instruments Used in Capillary Electrophoresis
- Power supply: Capillary electrophoresis requires a high voltage controllable direct current power supply.
- Buffer reservoirs: Capillary electrophoresis requires two buffer reservoirs held at the same level that contain specified anodic and cathodic solutions.
- Electrodes: CE needs two electrodes (cathode and anode) immersed in the buffer reservoirs connected to the power supply.
- Capillary: A capillary made up of fused silica, usually with a diameter of fewer than 100 microns.
- Viewing window: CE requires an optical viewing window that is aligned to the detector.
- Injection system: CE requires a suitable injection system for adding sample and buffer into the capillary. The method of injection can be automated for precision. The common mode of injecting samples is gravity, pressure or vacuum, or electrokinetic.
- Detector: CE requires a detector for monitoring the amount of substance that passes through the capillary at a given time based on conductimetric, fluorimetry, absorption spectrophotometry (UV and visible), amperometric, or mass spectrometric detection.
- Thermostatic system: CE requires a thermostatic system for maintaining a constant temperature inside the capillary.
- Recorder: CE requires a recorder that records the data after the completion of electrophoresis.
- Suitable integrator or computer: CE also needs the right integrator or computer to convert data digitally.
Types of Capillary Electrophoresis Method
There are six types of capillary electrophoresis methods commonly used; CZE (capillary zone electrophoresis), CGE (capillary gel electrophoresis), Micellar electro kinetic capillary chromatography (MEKC), capillary electrochromatography (CEC), capillary isoelectric focusing (CIEF), and capillary isotachophoresis (CITP).
Capillary zone electrophoresis (CZE)
The separation in this type of electrophoresis requires a capillary with only a buffer without any anticonvective medium. The analytes are separated into bands whose velocity depends on the electrophoretic mobility and electroosmotic flow. The electroosmotic flow moves toward the cathode, and when the polarity has reversed, the analytes with electroosmotic mobilities higher than the electroosmotic force will pass the outlet. The coated capillaries help increase the separation capacity of the substances that adhere to the fused-silica surfaces. The CZE is applicable in analyzing small to large (<2000 molecular weight to <100,000 molecular weight). It is a very efficient form of capillary electrophoresis.
Capillary gel electrophoresis (CGE)
The separation in capillary gel electrophoresis occurs in a capillary filled with a gel acting as a molecular sieve quite similar to gel electrophoresis . Molecules are separated according to molecular size. The smaller molecules move more freely through the gel network; hence these migrate faster than larger molecules. Capillary gel electrophoresis is used to separate proteins, DNA fragments, and other biological macromolecules with similar charge-to-mass ratios based on their molecular size.
Micellar electrokinetic capillary chromatography (MEKC)
The separation occurs in an electrolytic solution that consists of a surfactant with a concentration above the critical micellar concentration (CMC). This technique is a hybrid of electrophoresis and chromatography. The method is applicable to both neutral and charged solutes. The solute molecules get distributed between the pseudo-stationary phase made up of micelles and aqueous buffer based on the solute’s partition coefficient. The commonly used surfactant in MKEC is sodium dodecyl sulfate, cetyl trimethyl, and ammonium salts. In neutral and alkaline pH, the electroosmotic flow is strong, and the separation buffer ions move toward the direction of the cathode.
In case sodium dodecyl sulfate (SDS) is used as a surfactant, the anionic micelle’s electrophoretic migration is in the order of the anode, slowing down the overall micelle migration velocity in the bulk flow of the electrolytic solution. Suppose neutral solutes are present; the analyte partitions between the aqueous buffer and the micelle and lacks electrophoretic mobility. In such cases, the analyte’s migration velocity depends on the partition coefficient between the aqueous buffer and the micelle. Because of this, the separation in neutral and weakly ionized solutes in MKEC is chromatographic. In the case of charged solutes, migration velocity depends on the electrophoretic mobility of the solute without a micelle and the partition coefficient between the aqueous buffer and the micelle.
Capillary electrochromatography (CEC)
Capillary electrochromatography is a hybrid separation technique that uses the principle of both capillary electrophoresis and chromatography, especially high-performance liquid chromatography (HPLC) . Analytes separate based on the differences in the partition ratio between the mobile and stationary phase or due to electrophoretic mobility. The mobile phase is run across the chromatographic bed with the help of electroosmotic force instead of pressure. CEC is more advantageous than HPLC and capillary electrophoresis. It applies to enantiomeric separation, separating amino acids, proteins, peptides, and carbohydrates. It is mainly used in pharmaceutical industries to identify acidic and basic drugs and in the industrial sector that involves analyzing polymers.
Capillary isoelectric focusing (CIEF)
The charged molecules migrate due to the electric field in the isoelectric focusing in a pH gradient obtained from the ampholytes having pI (isoelectric point) value in a wide range that is dissolved in the separation buffer. There are three steps in CIEF; loading, focusing, and mobilization. The introduction of the ampholytes and separation buffer is loaded in the capillary in the loading step. In the focusing step, the voltage is provided in the capillary, causing the ampholytes to move to their respective electrode based on their net charges and creating a pH gradient where the molecules migrate until they reach a pH corresponding to their pI (isoelectric point). The mobilization can be required for detection, which is obtained by either focusing under the influence of electroosmotic flow, applying positive pressure after focusing, or adding salt to the anode or cathode reservoir, depending on the direction of mobilization.
Capillary isotachophoresis (CITP)
The technique was developed to separate the serum lipoproteins. Compared to other electrophoretic processes, it has negligible molecular sieve effects, requires no gel casting, applicable for whole serum, and can easily differentiate lipoprotein subfractions. Here, the total serum lipoproteins are pre-stained in a free-flow capillary system (with an internal diameter of 0.5 mm) having a discontinuous buffer system for 30 minutes at 4℃ before starting the process. The lipoproteins are separated into HDL (high-density lipoproteins) and LDL (low-density lipoproteins) based on electrophoretic mobility. The method is commonly used in clinical laboratories for determining the lipoprotein level in human serum.
Application of Capillary Electrophoresis
Capillary electrophoresis is commonly applied in various fields. Analyzing food contents, genetic differentiation, and clinical diagnosis are typical applications of capillary electrophoresis. The following is a detailed explanation of the area of applications of capillary electrophoresis:
- Food technology: Capillary electrophoresis is applied to analyze different food, like beverages and fermented food, to detect flavonoids, vitamins, carbohydrates, proteins, pigments, and color. CE is also applicable for detecting DNA and RNA from bacteria and viruses, which indicates contamination.
- Molecular analysis: The DNA, RNA, and amino acids are detected using capillary electrophoresis, especially in the microbiology laboratory and forensic science.
- Disease diagnosis: Lipid profile analysis using CITP is critical for detecting cholesterol levels in clinical laboratories. Likewise, the human serum’s analysis of vitamins and minerals is performed using capillary electrophoresis.
- Environmental monitoring: Capillary electrophoresis has been applied to track inorganic ions in rivers and water. (4) Likewise, nano-capillary electrophoresis is applied to detect environmental pollutants at the nano level. (5)
- Pharmaceutical laboratories: Capillary electrophoresis is used in the analysis of small molecules in drugs during preparatory stages and standard solutions. (6)
Advantages of Capillary Electrophoresis
Capillary electrophoresis has many advantages over other methods of electrophoresis and chromatography due to the use of tiny capillaries for separating. The following is a detailed explanation of the benefits of capillary electrophoresis:
- Highly efficient: Heat dissipation occurs efficiently due to using a small diameter of the capillary. Some articles claim the efficiency of capillary electrophoresis is quite similar to that of liquid chromatography. (7)
- Less time-consuming: If the person is familiar with computer software, it takes no time to read the result of separation. Likewise, the entire process takes only one hour, from setup to separation. So, capillary electrophoresis carries more advantages as compared to other separation techniques.
- Wide area of application: Capillary electrophoresis is used in analyzing macromolecules like proteins, DNA, different drugs, and RNA. So, CE is applied widely from pharmaceutical to environmental monitoring.
- Has the possibility of automation: The injection method, data analysis, buffer, and sample uploading steps can be automated in capillary electrophoresis.
Disadvantages of Capillary Electrophoresis
Although capillary electrophoresis is highly advantageous, it has some disadvantages, which are as follows:
- Changing capillaries is challenging: Due to the size of the capillaries, removing and replacing capillaries can be challenging at times. So, expertise is required for changing capillaries to experiment.
- Less robust: Small changes like the size of capillaries and choice of analytes can be crucial in capillary electrophoresis. So CE is less robust than other methods.
- Capillary electrophoresis . (n.d.). Retrieved December 16, 2022, from https://www.usp.org/sites/default/files/usp/document/harmonization/biotechnology/harmonization-september-2019-m859.pdf
- Borst, C., Belal, F., & Holzgrabe, U. (2013). Possibilities and limitations of capillary electropherosis in pharmaceutical analysis. Die Pharmazie , 68 (7), 526–530.
- Robert, F., Bouilloux, J. P., & Denoroy, L. (1991). L’électrophorèse capillaire: principe et applications [Capillary electrophoresis: principle and applications]. Annales de biologie clinique , 49 (3), 137–148.
- Sirén, H., & Väntsi, S. (2002). Environmental water monitoring by capillary electrophoresis and result comparison with solvent chemistry techniques. Journal of chromatography. A , 957 (1), 17–26. https://doi.org/10.1016/s0021-9673(02)00218-2
- Ali, I., Alharbi, O. M. L., & Marsin Sanagi, M. (2016). Nano-capillary electrophoresis for environmental analysis. Environmental chemistry letters , 14 (1), 79–98. https://doi.org/10.1007/s10311-015-0547-x
- Masár, M., Hradski, J., Schmid, M. G., & Szucs, R. (2020). Advantages and Pitfalls of Capillary Electrophoresis of Pharmaceutical Compounds and Their Enantiomers in Complex Samples: Comparison of Hydrodynamically Opened and Closed Systems. International journal of molecular sciences , 21 (18), 6852. https://doi.org/10.3390/ijms21186852
Ashma Shrestha
Hello, I am Ashma Shrestha. I had recently completed my Masters degree in Medical Microbiology. Passionate about writing and blogging. Key interest in virology and molecular biology.
We love to get your feedback. Share your queries or comments Cancel reply
This site uses Akismet to reduce spam. Learn how your comment data is processed .
Recent Posts
Electrophoresis: Principles, Types, and Uses
Electrophoresis is a simple and sensitive separation technique in clinical and research laboratories. Since its discovery, it has been an essential tool used by biologists and chemists to separate...
Agarose Gel Electrophoresis: Principle, Procedure, Results
Agarose gel electrophoresis is one of the most common electrophoresis techniques which is relatively simple and straightforward to perform but possesses great resolving power. The agarose gel...
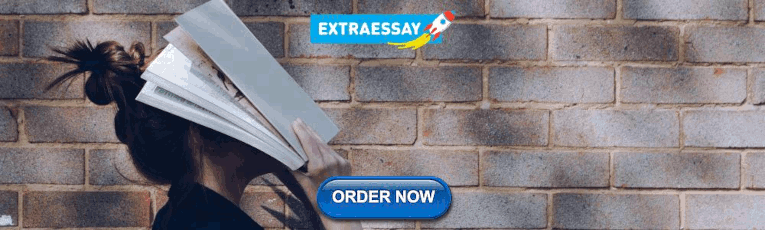
IMAGES
VIDEO
COMMENTS
Electrophoresis is the migration of electrically charged molecules under the effect of the electrical field.[1] In 1937, Tiselius, a biochemist from Sweden, demonstrated that particles with charge could be separated based on their charge with the help of an electrical field. Various biomolecules, such as proteins, peptides, nucleic acids ...
Depending upon the medium available for movement of charged analytes and format used for separation, electrophoresis can be broadly classified into gel electrophoresis, zone electrophoresis, free flow electrophoresis, and capillary electrophoresis (Fig. 1).Gel electrophoresis is the most frequently used method for the separation of nucleic acids and proteins in which supporting media in the ...
Electrophoresis-the migration and separation o f charged particles (ions) under the influence of an electric field. It is the movement of charged particles through an electrolyt e
Electrophoresis is a simple and sensitive separation technique in clinical and research laboratories. Since its discovery, it has been an essential tool used by biologists and chemists to separate mixtures, especially proteins and nucleic acids. Electrophoresis consists of two words; electro, meaning electricity, and phoresis, meaning movement.
ELECTROPHORESIS is an international separation science journal serving as the premier forum for advances in electrophoresis, liquid-phase separations, microfluidics and electrokinetics. We cover both fundamental aspects as well as innovative industrial and clinical applications linked to separation science, welcoming advances in sample preparation, methods, and analysis.
12.7: Electrophoresis is shared under a CC BY-NC-SA 4.0 license and was authored, remixed, and/or curated by LibreTexts. Electrophoresis is a class of separation techniques in which we separate analytes by their ability to move through a conductive medium—usually an aqueous buffer—in response to an applied ….
Electrophoresis is a general term that describes the migration and separation of charged particles (ions) under the influence of an electric field. An electrophoretic system consists of two electrodes of opposite charge (anode, cathode), connected by a conducting medium called an electrolyte. The separation effect on the ionic particles results ...
The most common implementation, gel electrophoresis, is a method used to separate nucleic acids by length or proteins by size, conformation and charge based on their migration through a porous matrix.
ELECTROPHORESIS is an international journal that publishes original manuscripts on all aspects of electrophoresis, and liquid phase separations (e.g., HPLC, micro- and nano-LC, UHPLC, micro- and nano-fluidics, liquid-phase micro-extractions, etc.). Topics include new or improved analytical and preparative methods, sample preparation ...
This chapter discusses electrophoresis in non-restrictive gels, which involves analyzing the response of proteins to electricity, and its applications in personalized medicine and regenerative medicine. Foreword. Preface. Abbreviations. Part I Fundamentals. Introduction. 1 Electrophoresis. 1.0 General. 1.1 Electrophoresis in non-restrictive gels. 1.2 Electrophoresis in restrictive gels. 2 ...
Paper Electrophoresis (Low Voltage) Low-voltage paper electrophoresis is the simplest and cheapest form of electrophoresis. A strip of commercially available chromatography paper is soaked in buffer and placed with one end in each buffer reservoir (connecting wicks may be used). It is important that the paper is saturated with the buffer since ...
Polyacrylamide gel electrophoresis is widely used for studying proteins and protein-containing objects. However, it is employed most frequently as a qualitative method rather than a quantitative one. This paper shows the feasibility of routine digital image acquisition and mathematical processing of electropherograms for protein quantification ...
Electrophoresis is a technique in which an electric current is applied, driving the separation of biological molecules, including DNA/RNA and proteins in a gel-based process. ... Please use one of the following formats to cite this article in your essay, paper or report: APA. Savage, Bryan. (2021, September 09). Electrophoresis: An Overview ...
This chapter focuses on the principle of electrophoresis and its utilization in a clinical laboratory. A sincere attempt has been made to discuss about clinical applications of serum protein electrophoresis, throwing light on the significance of serum protein electrophoresis in the management of multiple myeloma. Emphasis has been made on quality assurance in terms of accuracy and precision in ...
ELECTROPHORESIS offers a web-based manuscript submission and peer review system. Using this system is obligatory; conventional submission of manuscripts is not accepted. Date Protection Policy: By submitting a manuscript to or reviewing for this publication, your name, email address, and affiliation, and other contact details the publication ...
Gel electrophoresis is a technique used to separate DNA fragments (or other macromolecules, such as RNA and proteins) based on their size and charge. Electrophoresis involves running a current through a gel containing the molecules of interest. Based on their size and charge, the molecules will travel through the gel in different directions or at different speeds, allowing them to be separated ...
In this method, the electrophoresis is carried in solution, without a supporting media. The sample is dissolved the buffer and molecules move to their respective counter charge electrodes. Moving boundary electrophoresis is carried out in a U shape tube with platinum electrodes attached to the end of both arms (Figure 13.2).
In life sciences, an electrophoresis is a vital tool that separates protein molecules or DNA according to its size and type. Table of Contents. Paper electrophoresis. Agarose gel electrophoresis. Polyacrylamide gel electrophoresis. Denaturing gradient gel electrophoresis. Isoelectric focusing and 2-D gel electrophoresis.
On the basis of supporting media, it is of following types. Paper electrophoresis. Cellulose acetate electrophoresis. Capillary electrophoresis. Gel electrophoresis. The equipment used for the electrophoresis basically consists of two items: An electrophoretic unit and a power pack. Electrophoretic unit is of two types: Vertical electrophoretic ...
Definition. 00:00. …. Electrophoresis is a laboratory technique used to separate DNA, RNA or protein molecules based on their size and electrical charge. An electric current is used to move the molecules through a gel or other matrix. Pores in the gel or matrix work like a sieve, allowing smaller molecules to move faster than larger molecules.
Electrophoresis is a technique used to separate molecules in a gel or fluid using an electric field. The rate and direction of particle movement in the electric field depends on the molecule's size and electric charge. Usually electrophoresis is used to separate macromolecules, such as DNA, RNA, or proteins. Read More.
3. The Interconnected Lives of Electrophoretic Experiments. In contrast to other big manufacturers of research equipment, 20 the main manufacturer of electrophoresis equipment and consumables in today's laboratories is a US‐based family firm, Bio‐Rad Laboratories, Inc. The company was founded in 1952 in Berkeley, California, and entered the blooming experimental culture of identification ...
Capillary electrophoresis is a liquid-based separation technique that uses a capillary as a separation channel under the influence of an electric field. It is an analytical technique where the electroosmotic flow helps separately charged ions. It is applicable in various areas like analysis of chemical substances, DNA analysis, identifying ...
Feature papers represent the most advanced research with significant potential for high impact in the field. A Feature Paper should be a substantial original Article that involves several techniques or approaches, provides an outlook for future research directions and describes possible research applications.