Advances in Melanoma and Other Skin Cancers Research
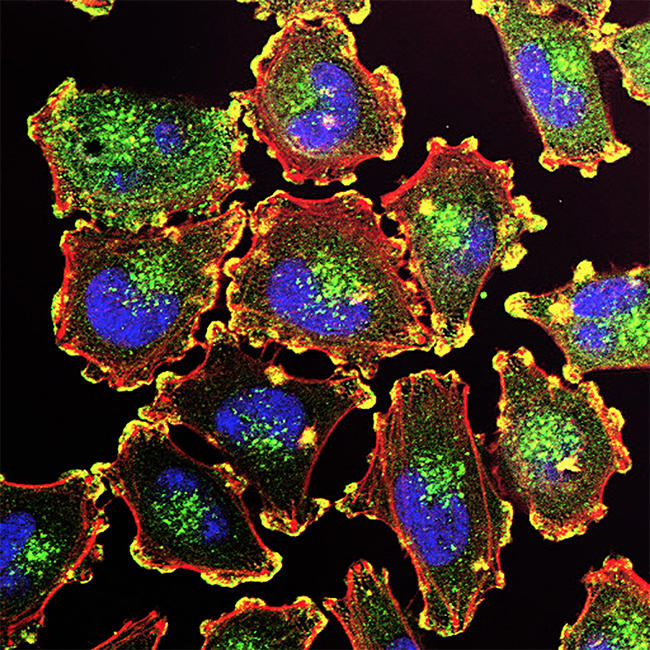
Metastatic melanoma cells.
NCI-funded researchers are working to advance our understanding of how to treat melanoma and other skin cancers. Much progress has been made in treating people with melanoma that has spread in their bodies ( metastatic melanoma). Yet many people still don't benefit from the newest drugs, and others may relapse after initially successful treatment.
This page highlights some of the latest research in treatment for melanoma and other skin cancers, including clinical advances that may soon translate into improved care, NCI-supported programs that are fueling progress, and current research findings from recent studies.
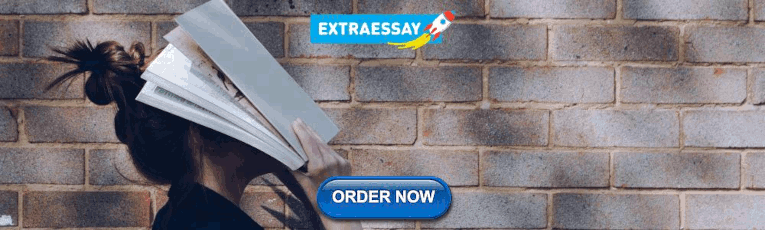
Melanoma Treatment
Surgery remains the standard treatment for early-stage melanoma and may also be used as part of therapy for more advanced disease. However, researchers are now focusing on developing treatments that directly target specific mutations in melanoma cells or that harness the body’s immune system to attack melanoma.
Both of these approaches— targeted therapies and immunotherapies —have led to dramatic improvements in survival for patients with advanced melanoma over the last decade. Researchers are continuing to explore ways to make these treatments more effective for more patients.
Targeted therapies
Targeted therapies use drugs or other substances to attack specific types of cancer cells with less harm to normal cells. About half of people with melanoma that has metastasized or can’t be removed with surgery ( unresectable melanoma) have mutations in the BRAF gene . These mutations result in abnormal B-Raf proteins that can cause uncontrolled growth of melanoma cells.
Drugs have been developed that block the effects of these altered B-Raf proteins. Other new drugs block proteins that work together with altered B-Raf proteins to promote cancer cell growth. These include proteins produced by the MEK genes. The combination of blocking both B-Raf and MEK has been found to be particularly successful in treating melanoma that has a mutation in the BRAF gene. Three such combinations are approved for people with metastatic or unresectable melanoma that has mutations in the BRAF gene:
- dabrafenib (Tafinlar) and t rametinib (Mekinist)
- encorafenib (Braftovi) and b inimetinib (Mektovi)
- vemurafenib (Zelboraf) and c obimetinib (Cotellic)
However, although these drug combinations may be effective initially, most people develop resistance to them within a year. Researchers are studying how melanoma cells manage to grow in the presence of these targeted therapies, with the goal of finding ways to overcome resistance. Ideas being tested include new drug combinations and drugs that target the B-Raf pathway in different ways than existing drugs.
Immune checkpoint inhibitors
Immunotherapies are treatments that help the body’s immune system fight cancer more effectively. Melanoma tends to have a relatively high number of genetic mutations that can be recognized by the immune system compared with other cancer types. This makes it more likely that melanoma will respond to immunotherapy.
One type of immunotherapy, called immune checkpoint inhibition , has shown impressive results in some people with advanced melanoma. Four immune checkpoint inhibitors are now approved for the treatment of melanoma that can’t be removed with surgery or that has metastasized:
- i pilimumab (Yervoy)
- pembrolizumab (Keytruda)
- nivolumab (Opdivo)
- atezolizumab (Tecentriq) , when used in combination with two targeted drugs
The combination of ipilimumab and nivolumab is also approved for some patients with metastatic or unresectable melanoma. In the study that led to its approval, more than half of the people who received the combination were alive 5 years after treatment . Another clinical trial showed that this combination can also shrink melanoma that has spread to the brain in some patients.
The combination of nivolumab with a new type of immune checkpoint inhibitor called relatlimab also improved the amount of time people with advanced melanoma lived without their cancer getting worse . This combination received FDA approval in 2022, under the name Opdualag , for people aged 12 or older with untreated melanoma that can't be removed surgically or has spread within the body.
Scientists are looking for ways for more people to have success with these drugs. Unfortunately, even when used in combination, immune checkpoint inhibitors don't work for all patients with metastatic or unresectable melanoma. However, patients whose tumors do shrink or disappear often have responses that last for years. Researchers are now testing ways to increase the number of people with melanoma who benefit from this type of treatment, such as these below.
- Combining immune checkpoint inhibitors with immunostimulant s. Immunostimulants produce a type of chemical alarm in the body that tells the immune system that a threat exists. In a small clinical trial that combined pembrolizumab with an immunostimulant, tumors shrank in almost 80% of people who received the two treatments together . Larger trials of this and other combinations of immunotherapy drugs are underway.
- Testing new and existing immune checkpoint inhibitors in combination with targeted therapies and other types of drugs.
- Changing people’s gut microbes before treatment with an immune checkpoint inhibitor. For example, a study led in part by NCI researchers found that changing some people’s gut microbes could make their melanoma more likely to shrink during treatment with an immune checkpoint inhibitor.
Learning what treatments to give first
Melanoma researchers are also looking to understand how best to use existing therapies. One pressing question had been whether it is better for people who have advanced melanoma with mutations in the BRAF gene to receive targeted drugs or immune checkpoint inhibitors first.
An NCI-sponsored trial, DREAMseq, has helped answer this question. Patients with advanced melanoma were randomly assigned to receive either a combination of targeted drugs or a combination of immune checkpoint inhibitors. When their cancer recurred, they received the other combination. The study found that more people who received the immune checkpoint inhibitor combination first were still alive 2 years later than people who received the combination of the targeted drugs first.
Researchers are also searching for biomarkers in melanoma that can predict which tumors might respond to other immunotherapies or drug combinations.
Harnessing the body’s immune cells
Adoptive cell therapy. Another type of immunotherapy, called adoptive cell therapy (ACT), is also being tested in patients with metastatic melanoma. In ACT, T cells (a type of immune cell) are given to a patient to help the body fight diseases, such as cancer.
In a small, early-phase clinical trial of ACT, about half of patients with metastatic melanoma saw their tumors shrink, and a quarter remained in remission for as long as the study tracked them—in some cases for up to a decade. But the procedure is complicated and expensive, and half of people do not benefit from the treatment at all or experience dangerous or even fatal side effects. Researchers are looking for ways to make ACT work for more patients such as the examples below.
- One idea being tested is the use of immune cells that have been collected from patients, altered to make them better at killing cancer cells, and then infused back into patients. Such therapies include CAR T cells, a type of treatment where a patient's T cells are changed in the lab so they will attack cancer cells . Researchers are also testing other ways to boost the ability of T cells to kill tumor cells.
- Another idea is to find common proteins that are present in many people's tumors. This could allow for the creation of “off-the-shelf” T-cell therapies that don’t have to be made on a custom basis for each patient.
Immunotherapy following surgery for advanced melanoma
Adjuvant therapy is additional cancer treatment given after primary surgical treatment. Nivolumab, ipilimumab, and pembrolizumab have all been approved as adjuvant therapies for melanoma that has spread to nearby lymph nodes but can be removed with surgery. In clinical trials, all three immune checkpoint inhibitors reduced the risk of recurrence for some patients when given after surgery, although many patients experienced serious side effects.
Another study tested pembrolizumab in patients with early-stage melanoma that has not spread to the lymph nodes but had a high risk of doing so. It found that giving pembrolizumab after surgery reduced the chance of the cancer coming back or spreading elsewhere in the body . However, the treatment can cause significant side effects. More studies are needed to understand how to identify the people with this type of high-risk, early-stage melanoma who would benefit the most from such treatment.
Researchers are also exploring whether immune checkpoint inhibitors might be more effective if given before surgery. One NCI-sponsored trial compared the outcomes of patients with melanoma at high risk of recurring who receive pembrolizumab both before and after surgery with those in patients who receive the drug only after surgery. That trial found that people who received the drug both before and after surgery had a substantially lower risk of their cancer coming back than those who only received adjuvant treatment.
Rare Melanoma Types
Some rare types of melanoma have lagged behind melanoma of the skin in terms of advances in treatment. These include intraocular (uveal) melanoma , which starts in the eye; desmoplastic melanoma , a rare form of melanoma of the skin; and mucosal melanoma, which begins in the mucosal membranes , such as the linings of the nose and mouth.
However, recent small clinical trials suggest that these types of melanoma may also respond to immunotherapies. One NCI-sponsored trial tested pembrolizumab in people with desmoplastic melanoma . Initial results from this trial showed that the drug shrinks both tumors that can be removed surgically and those that cannot. The trial participants are still being tracked to see if pembrolizumab improves how long they live overall.
Immune checkpoint inhibitors have been less effective in intraocular melanoma than in other types of melanoma. However, a different type of immunotherapy called a bispecific fusion protein has shown promise for treating this rare cancer. These drugs bind to melanoma cells and the body’s own immune cells at the same time, to bring them together. This allows the immune cells to kill the melanoma cells. In a clinical trial, one such drug, called tebentafusp, became the first drug to show an improvement in overall survival for patients with metastatic intraocular melanoma.
Merkel Cell Carcinoma
Another rare type of skin cancer, called Merkel cell carcinoma (MCC), has been shown to be the most sensitive of any tumor type to treatment with a single immune checkpoint inhibitor. In 2017, an immunotherapy called avelumab (Bavencio) received the first-ever FDA approval for a drug to treat MCC. In addition, more than half of patients with MCC in a small clinical trial had their tumors shrink or disappear during treatment with pembrolizumab, which received FDA approval for the treatment of MCC in 2018.
In 2023, a third immunotherapy drug called retifanlimab (Zynyz) received FDA approval for the treatment of MCC that has recurred or spread elsewhere in the body. Other immunotherapy drugs are currently being tested in this rare cancer type.
Treatment for Advanced Basal Cell Carcinoma and Squamous Cell Carcinoma
Basal cell carcinoma (BCC) and squamous cell carcinoma (SCC) of the skin are the most common cancers in the United States. They rarely spread to other organs and are seldom fatal. However, every year many people are diagnosed with advanced BCC or SCC.
Recent breakthroughs in targeted therapies and immunotherapies have changed the way people with advanced BCC and SCC are treated. Ongoing research seeks to build on these breakthroughs such as:
- The targeted drugs sonidegib (Odomzo) and vismodegib (Erivedge) which can control tumors for a long time in some people with BCC. However, resistance often develops. In addition, side effects can cause some patients who need to take the drugs for a long time to stop taking them. Researchers are now looking for ways to change when and how much of these drugs are given, both to delay the development of resistance and to make them easier to tolerate.
- cemiplimab (Libtayo) for some people with metastatic or locally advanced SCC that can't be removed with surgery. Cemiplimab is also being tested as a treatment given before surgery for some people whose cancer can be removed.
- pembrolizumab for some people with recurrent or metastatic SCC
- cemiplimab for some people with advanced BCC whose tumors have become resistant to targeted therapy
New clinical trials are now testing other immunotherapy drugs and combinations in SCC and BCC.
For people whose BCC or SCC has not spread, surgery remains the mainstay of treatment. But less-intensive versions of radiation therapy have been developed for people who can’t tolerate surgery for larger tumors, such as the frail elderly.
NCI-Supported Research Programs
Many NCI-funded researchers at the NIH campus, and across the United States and world, are seeking ways to address melanoma and other skin cancers more effectively. Some research is basic, exploring questions as diverse as the biological underpinnings of cancer and the social factors that affect cancer risk. And some is more clinical, seeking to translate this basic information into improving patient outcomes. The programs listed below are a small sampling of NCI’s research efforts for melanoma and other skin cancers.
Scientists in the Division of Cancer Epidemiology and Genetics (DCEG) study families in which multiple members have developed certain cancers. In collaboration with the Melanoma Genetics Consortium (GenoMEL), DCEG researchers are searching for new genes in both melanoma-prone families and through a genome-wide association study to find genes that may increase the risk of melanoma.
The Skin Specialized Programs of Research Excellence (Skin SPOREs) are designed to quickly move basic scientific findings into clinical settings. The Skin SPORE program’s main focus is on melanoma research activities, but it also includes projects in other skin cancer types, such as cutaneous T-cell lymphoma.
NCI's National Clinical Trials Network (NCTN) is a collection of organizations and clinicians that coordinates and supports cancer clinical trials at more than 3,000 sites across the United States and Canada. NCTN currently has a variety of trials testing treatments for skin cancer .
The Division of Cancer Control and Population Sciences (DCCPS) oversees the Cancer Trends Progress Report, an online report that tracks the nation's progress against cancer from prevention through end of life. Topics in the report that inform melanoma and skin cancer research are sun-protective behavior , indoor and outdoor tanning , and sunburn . The division’s Health Behaviors Research Branch (HBRB) supports research in the area of sun protection and reducing indoor tanning practices, through both measurement and intervention studies.
Clinical Trials
NCI funds and oversees both early- and late-stage clinical trials to develop new treatments and improve patient care. Trials are available for melanoma prevention and treatment and non-melanoma skin cancer prevention and treatment .
Melanoma and Other Skin Cancers Research Results
The following are some of our latest news articles about research on melanoma and other skin cancers:
- First Cancer TIL Therapy Gets FDA Approval for Advanced Melanoma
- Rare Melanoma Very Likely to Respond to Treatment with Pembrolizumab
- Immunotherapy before Surgery Appears Effective for Some with Melanoma
- Androgen Receptor May Explain Sex Differences in Melanoma Treatment Response
- Study Adds to Debate about Screening for Melanoma
- Opdualag Becomes First FDA-Approved Immunotherapy to Target LAG-3
View the full list of Melanoma and Other Skin Cancers Research Results and Study Updates .

- Adolescent and Young Adult Cancer
- Bile Duct Cancer
- Bladder Cancer
- Brain Cancer
- Breast Cancer
- Cervical Cancer
- Childhood Cancer
- Colorectal Cancer
- Endometrial Cancer
- Esophageal Cancer
- Head and Neck Cancer
- Kidney Cancer
- Liver Cancer
- Lung Cancer
- Mouth Cancer
- Mesothelioma
- Multiple Myeloma
- Neuroendocrine Tumors
- Ovarian Cancer
- Pancreatic Cancer
- Prostate Cancer
- Skin Cancer/Melanoma
- Stomach Cancer
- Testicular Cancer
- Throat Cancer
- Thyroid Cancer
- Prevention and Screening
- Diagnosis and Treatment
- Research and Clinical Trials
- Survivorship

Request an appointment at Mayo Clinic

Advances in surgery are improving survival for people with melanoma
Share this:.

By Mayo Clinic staff
The National Cancer Institute estimates that 97,610 people will be diagnosed with melanoma in 2023, making up 5% of all new cancer diagnoses. Fortunately, screening and treatment have improved, allowing care teams to catch melanoma earlier when it's easier to treat. As a result, the number of people who survive the disease has steadily increased.
Tina Hieken, M.D. , a Mayo Clinic surgical oncologist with a particular interest in melanoma , answers questions about treatment and advances in surgery that are helping to improve outcomes for people diagnosed with the disease:
Who is diagnosed with early-stage melanoma?
We have seen a shift over the last several decades to an increase in people being diagnosed with earlier-stage disease, along with an overall increase in the incidence of melanoma. Melanoma has historically been one of the most rapidly increasing cancers, but this has varied by age. In adults age 50 and older, rates continue to grow in women by about 1% a year but have stabilized in men. As the population ages, the average age at diagnosis of melanoma is closer to age 60; however, melanoma is one of the most common cancers in adults younger than 30.
What are some important considerations in treating early-stage melanoma?
Finding melanoma at its earliest stage allows us to treat the lesion with surgery — without using systemic therapies that reach and affect cells throughout the body, creating potential toxicities and side effects. But even the earliest stage of the disease requires a specialized, multidisciplinary care team to ensure the patient has the best treatment options, as melanoma care is evolving rapidly. The melanoma care team at Mayo Clinic includes surgical oncologists , medical oncologists , radiation oncologists , dermatologists , radiologists and pathologists . We work together and with other scientists to bring research advancements into care as quickly as possible.
How has research advanced the treatment of early-stage melanoma in recent years?
At Mayo Clinic, we have many open clinical trials in which we're applying new ideas to patient care:
- One study is enrolling patients with stage 1 and 2 melanoma to evaluate whether a melanoma gene signature developed at Mayo Clinic can predict a patient’s sentinel lymph node status. A sentinel lymph node is the first lymph node to which cancer cells spread from a primary tumor. A sentinel node biopsy is often performed to accurately stage patients whose disease appears not to have spread beyond the skin. During the procedure, the sentinel node is removed and a pathologist examines it under a microscope to see if there is evidence that the melanoma has spread. This study aims to determine if a melanoma gene signature can help determine which patients may benefit from sentinel node surgery and which patients may safely avoid it.
- Another study is testing the injection of a type of immunotherapy directly into the tumors of patients with melanoma that involve soft tissue.
- We also are testing new treatments given by injection through a vein for patients with more advanced disease that has spread to the lymph nodes but can still be removed with surgery. These new combinations of drugs are given to patients with a high risk of disease recurrence following surgery.
- For patients with high-risk disease that can be treated with surgery, we are investigating neoadjuvant systemic therapy. Neoadjuvant systemic therapy uses substances that travel through the bloodstream and is given before surgery to shrink tumors. We have a clinical trial testing new immunotherapy combinations for melanoma patients whose cancer has spread to nearby lymph nodes.
How might surgery help a patient with later-stage melanoma?
While we typically think of surgery as an option primarily for early-stage disease, surgical oncologists are increasingly involved in caring for patients with advanced disease. For example, some patients may have a mixed response to systemic therapy and a surgical oncologist will remove any remaining cancer with surgery.
Surgeons may also remove tumors to obtain active immune cells for cellular therapies being tested in clinical trials. We anticipate that these treatments will become approved for clinical use soon.
Surgical oncologists work with the multidisciplinary team to create the best possible treatment plan for each patient. Ultimately, it's all about bringing all the right people into a patient's care team. When working together, we can assess treatment responses and make changes quickly. We work together to provide the best personalized options for patients with melanoma.
Watch this video to hear more about how surgery is used to treat melanoma at Mayo Clinic.
Learn more about melanoma and find a melanoma clinical trial at Mayo Clinic.
Join the Skin Health Support Group on Mayo Clinic Connect, an online community for patients to get answers, ask questions and connect.
Also, read these articles:
- " Treating skin cancer with Mohs surgery "
- " Melanoma misconception — dark skin tones at risk, too "
- " Cancer-free and embracing new adventures thanks to Mayo’s collaborative care "
- " Why men need to take melanoma seriously "
- " Dawn’s journey: Overcoming a near-zero survival rate "
- " Melanoma spread: How lymph nodes play a role in detection "
- " Is a cancer clinical trial right for me? "
A version of this article was originally published on the Mayo Clinic Medical Professionals website .
Related Posts

People with lymphedema may need regular skin cancer screenings, since early detection of skin cancer is critical.

Dr. Melanie Dixon counters top sunscreen myths with facts everyone should know to reduce skin cancer risk.

Dr. Naiara Sbroggio Barbosa explains how Mohs surgery can be used to treat basal cell carcinoma, squamous cell carcinoma, and some types of melanoma.
- Reference Manager
- Simple TEXT file
People also looked at
Review article, advances in melanoma: epidemiology, diagnosis, and prognosis.
- 1 Department of Dermatology, Temple University Hospital, Philadelphia, PA, United States
- 2 Department of Dermatology, Thomas Jefferson University, Philadelphia, PA, United States
Unraveling the multidimensional complexities of melanoma has required concerted efforts by dedicated community of researchers and clinicians battling against this deadly form of skin cancer. Remarkable advances have been made in the realm of epidemiology, classification, diagnosis, and therapy of melanoma. The treatment of advanced melanomas has entered the golden era as targeted personalized therapies have emerged that have significantly altered the mortality rate. A paradigm shift in the approach to melanoma classification, diagnosis, prognosis, and staging is underway, fueled by discoveries of genetic alterations in melanocytic neoplasms. A morphologic clinicopathologic classification of melanoma is expected to be replaced by a more precise molecular based one. As validated, convenient, and cost-effective molecular-based tests emerge, molecular diagnostics will play a greater role in the clinical and histologic diagnosis of melanoma. Artificial intelligence augmented clinical and histologic diagnosis of melanoma is expected to make the process more streamlined and efficient. A more accurate model of prognosis and staging of melanoma is emerging based on molecular understanding melanoma. This contribution summarizes the recent advances in melanoma epidemiology, classification, diagnosis, and prognosis.
Introduction
The word melanoma brings about fear among the public, patients, and clinicians alike. Perceived as the deadliest form of skin cancer, the scientific community of researchers and clinicians has made concerted efforts to bring about meaningful changes in morbidity and mortality associated with the cancer. Early on, the focus has been on screening and early detection of melanoma that have resulted in a rapid rise in the incidence of early thin melanomas in the last 50 years in countries of fair skin individuals. The diagnosis and treatment of melanoma have made significant strides in the past decade, which coincided with the understanding of genomic basis of melanomas. The diagnosis of melanoma is no longer solely relied upon histologic interpretation of skin biopsies, but, rather, more precise molecular based ancillary tests have emerged to aid the pathologists. The treatment of advanced melanoma has entered the golden era as targeted personalized therapies have emerged that have significantly altered the mortality rate. In this contribution, the current advances in melanoma epidemiology, diagnosis, and prognosis are reviewed.
Epidemiology
While the incidence and mortality of most cancers have declined over the past several decades, the incidence of melanoma continues to rise, particularly in the countries of fair-skinned populations of European descent. In 2020, the global burden of melanoma increased to 325,000 cases from 230,000 cases in 2012, a 41% increase ( 1 ). The highest incidence of melanoma is observed in Australia and New Zealand ( 1 ). In the United States (US), melanoma is the fifth most common cancer diagnosed with an estimated 99,780 new cases and 7,650 deaths in 2022, while 97,920 melanoma in-situ new cases are expected, a number that rivals the invasive melanomas ( 2 ).
Incidence and mortality
Invasive melanomas account for about 1% of all skin cancer cases, but they account for over 75% of skin cancer deaths ( 3 ). Though melanoma is perceived as a deadly cancer, the overall 5-year survival rate is 93.5% ( 3 ). The relative high survival rate of melanoma reflects the high proportion of localized disease (78%) that comprise the newly diagnosed invasive melanomas, which has 5-year survival rate of 99.6% ( 3 ). Despite the high survival rate, the small fraction of Stage I disease progression accounts for majority of the melanoma deaths ( 4 ). Stage III and IV disease have a survival rate of 73.9 and 35.1% respectively, a significant improvement since the introduction of targeted therapies and immunotherapies ( 3 ). In 2015, the 5-year survival rate of Stage IV disease was only 15% in comparison.
According to the Surveillance, Epidemiology, End Results (SEER) Program, the median age of melanoma diagnosis in the US is 66 years old ( 3 ). The lifetime risk of developing melanoma in White people is 2.6% (1 in 38), 0.6% (1 in 167) in Hispanic people, and 0.1% (1 in 1000) in Black people ( 5 ). The incidence rate is higher in men, a rate that is 1.6 times higher than women. While melanoma can develop at any skin site, the arms and legs are the most common site of involvement in women and the head, neck, back, and trunk are more commonly involved in men ( 6 ). African-American patients are more likely to develop melanoma on the plantar feet and other sun protected areas ( 3 ). Additionally, African-American patients are likely to have more advanced melanoma at the time of diagnosis and generally have a worse prognosis than their White counterparts.
Epidemic of melanoma
Over the past four decades, there has been a dramatic rise in the incidence of melanoma that has reached epidemic proportions. In the US, a threefold increase in the incidence rate has been observed during this period according to the SEER data all the while the mortality rate remained stable for most of the period ( Figure 1 ) ( 7 ). Similar incidence and mortality trends have been observed in developed countries in Europe and Australia over the same period. In the US, the sharp rise in the incidence has coincided with promotion of skin cancer screening and public awareness campaigns in the early 80s. Cancer screening, in general, has an intuitive appeal for clinicians and the lay public: detect cancers early when it’s more curable and manageable to prevent their expected morbidity and mortality. The late A. B. Ackerman urged clinicians and pathologists to diagnose melanomas early at a stage that is small, flat, and curable ( 8 ). The widespread adoption of dermatoscopy, a diagnostic technique promoted to detect incipient and incognito melanomas, further contributed to the detection of even earlier stage melanomas ( 9 , 10 ).
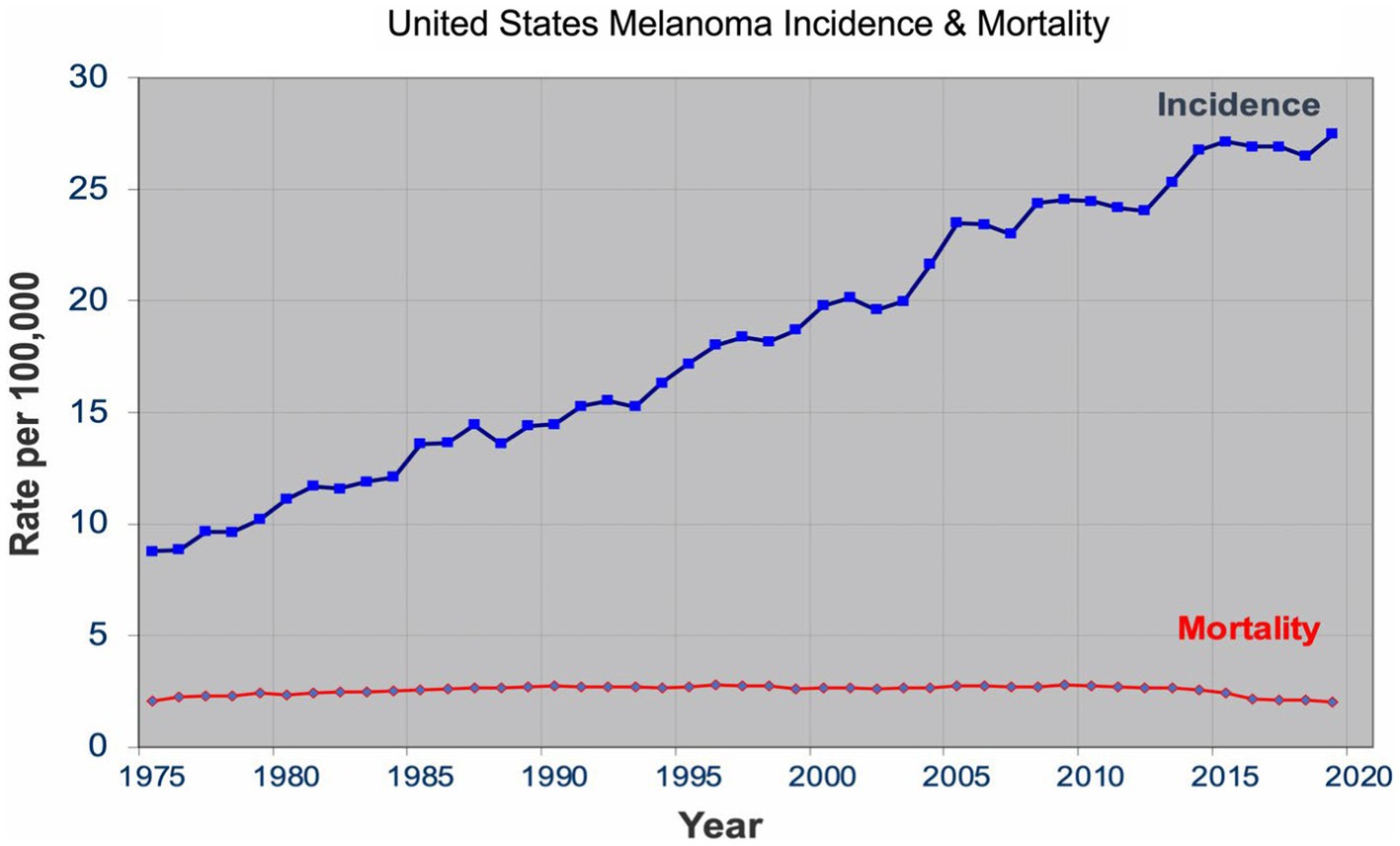
Figure 1 . United States melanoma incidence and mortality.
The sharp rise in the incidence of early-stage melanomas without the concomitant rise in mortality has brought the issue of overdiagnosis in the foreground ( 7 , 11 – 15 ). Overdiagnosis is defined as identification of a cancer, if left alone, that would not have caused death ( 16 , 17 ). It is an epidemiologic phenomenon that is easily discernable at the population level, but not at the patient or slide level. The mounting epidemiologic evidence indicates that most of the early melanomas diagnosed represent indolent or biologically benign forms, which are not the obligate precursors to the deadly forms of melanoma, and that the stable mortality reflects the stable incidence of the aggressive melanomas that screening do not capture ( 7 , 18 – 21 ). Furthermore, over the past two decades, the incidence of melanoma in situ has dramatically increased from 28,600 in 2000 to 101,280 in 2021 in the US ( 22 , 23 ). Despite the marked increase, no decrease in late disease have been observed. Moreover, they are diagnosed at a later age than the invasive melanomas, further bolstering the argument that they are not obligate precursors of invasive melanomas ( 24 – 26 ).
Effective cancer screening results in a decrease in late-stage disease and mortality. By this metric, cervical and colon cancer screenings qualify as effective cancer screening programs ( 27 ). For both cancers, there is a reliable precursor lesion that are screened and removed, human papillomavirus induced cervical intraepithelial neoplasia and colon polyps, respectively. For melanoma, a reliable precursor lesion has been elusive. First described by Wallace Clark in 1978, the dysplastic nevus was promulgated as a precursor lesion to melanoma ( 28 – 31 ), which began an era of close monitoring and their removal that still continues today. The countless biopsies and subsequent excisions of dysplastic nevi, over the past four decades, however, failed to make a difference in the late-stage disease and mortality rate.
The United States Preventive Service Task Force (USPSTF) has consistently given a grade of I for insufficient evidence for or against skin cancer screening, primarily because there are no population-level or randomized controlled trials that demonstrate the benefits of screening ( 32 ). There was an initial excitement about the preliminary population data that indicated a decreased melanoma-specific mortality in northern Germany, but the benefit was short-lived and longer follow-up, and the subsequent nationwide population screening had no impact on melanoma-specific mortality ( 33 – 35 ). Accordingly, no major medical societies and organizations in the US have a formal recommendation on skin cancer screening.
According to Welch and coworkers ( 12 ), the rapid rise in the incidence is the byproduct of “epidemic of inspection, surveillance, and biopsy of pigmented skin lesions.” The authors recommend curtailing self-referral of skin biopsy specimens, increasing the threshold to biopsy, particularly small, pigmented lesions, increasing the histopathological threshold in the diagnosis of melanomas, and ceasing all population-based skin cancer screenings. The dermatology community is unlikely to follow these recommendations as perceived benefits of screening and early detection are entrenched in the community and overdiagnosis cannot be perceived at the patient or slide level. Without any pivot in the detection strategy, however, epidemiologic evidence of overdiagnosis is expected to become more pronounced. Thyroid cancer has similar issues of overdiagnosis and has a nearly identical incidence and mortality rate pattern. In 2017, USPSTF gave a grade of D for thyroid cancer screening, which resulted in a decrease in the incidence with a mortality rate that remained unchanged in the subsequent years ( 36 , 37 ). Without formal evidence of benefit, population skin cancer screening is at risk of receiving a grade of D for discourage screening as did thyroid cancer screening. Opportunities awaits dermatology community to perform the necessary studies that show the benefits of screening, particularly in populations that are at high risk of developing melanoma ( 38 ).
Risk factors
Environmental.
Ultraviolet radiation (UVR) from sun exposure has been firmly established as the dominant environmental factor that increases the risk of developing melanoma ( 39 ). Intermittent sun exposure, particularly resulting in blistering sunburns, has been hypothesized to increase the risk of melanoma development ( 40 ). Meta-analyses have concluded that the relative risk is approximately 2 for sunburn history and 1.3 for tanning bed history ( 41 , 42 ). In comparison, smoking and lung cancer have a relative risk of 10–20. The relationship between UVR and melanoma risk is a complex one, a relationship that still needs to be further clarified.
Current epidemiologic data suggest the existence of three heterogeneous forms of melanomas: (1) slow-growing melanomas associated with intermittent sun exposure and melanocytic nevi, (2) slow-growing indolent melanomas associated with chronic sun exposure occurring on the head and neck ( Figures 2 , 3 ), (3) fast-growing aggressive melanomas minimally associated with sun exposure and melanocytic nevi ( Figure 4 ) ( 43 – 45 ). The fast-growing melanomas are not amenable to screening due to their rapid growth rate. They also elude detection because they do not harbor predictable clinical features, often simulating a benign and malignant non-melanocytic lesions and even inflammatory diseases ( 46 ). Nodular melanomas, particularly amelanotic ones, present as rapid growers ( 46 ). The current epidemic of melanoma consists of mostly slow growing thin melanomas because of screening efforts, which identify melanomas with early stages that are stretched out much longer in time. Lentigo maligna and superficial spreading type of melanoma fall into this category of melanoma.
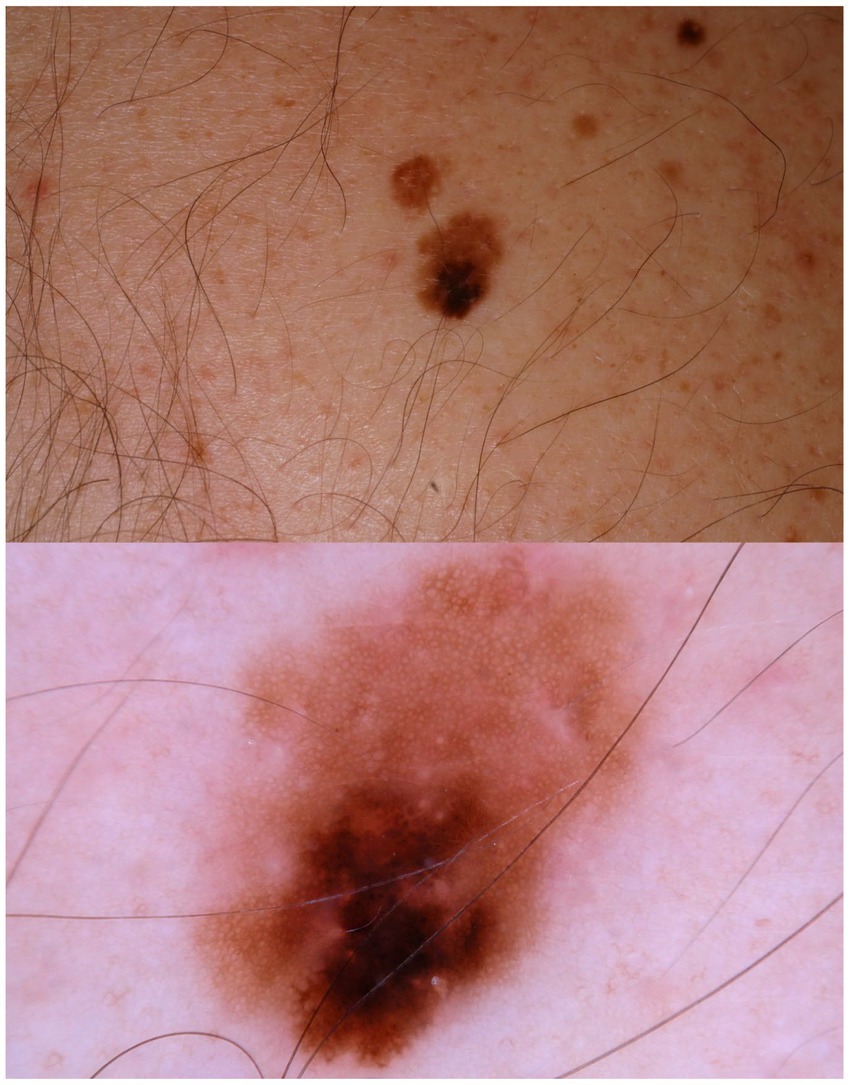
Figure 2 . A patient multiple nevi presents with a changing mole. Dermatoscopic image shows an asymmetric melanocytic lesion with regular network and black blotch.
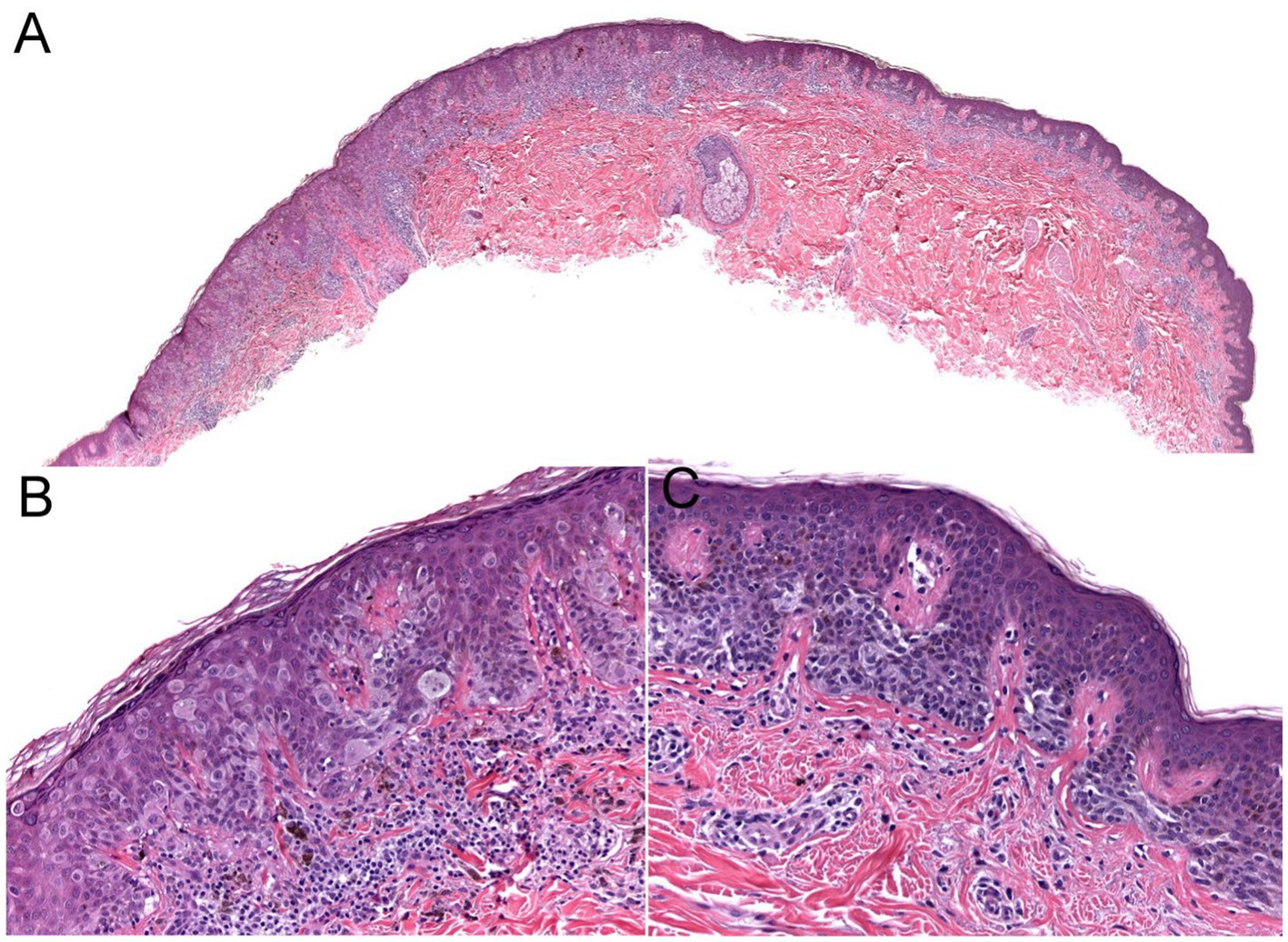
Figure 3 . The biopsy of the Figure 2 lesion showing a thin (Stage 1A) superficial spreading melanoma arising in a dysplastic nevus. (A) A shave biopsy showing a melanocytic lesion with an asymmetric architecture (20× magnification). (B) The left side of the lesion shows the melanoma in situ component: large atypical pagetoid melanocytes in pagetoid spread within the epidermis (200× magnification). (C) The right side of the lesion shows the nevus component: nested monomorphous melanocytes at the dermoepidermal junction (200× magnification).
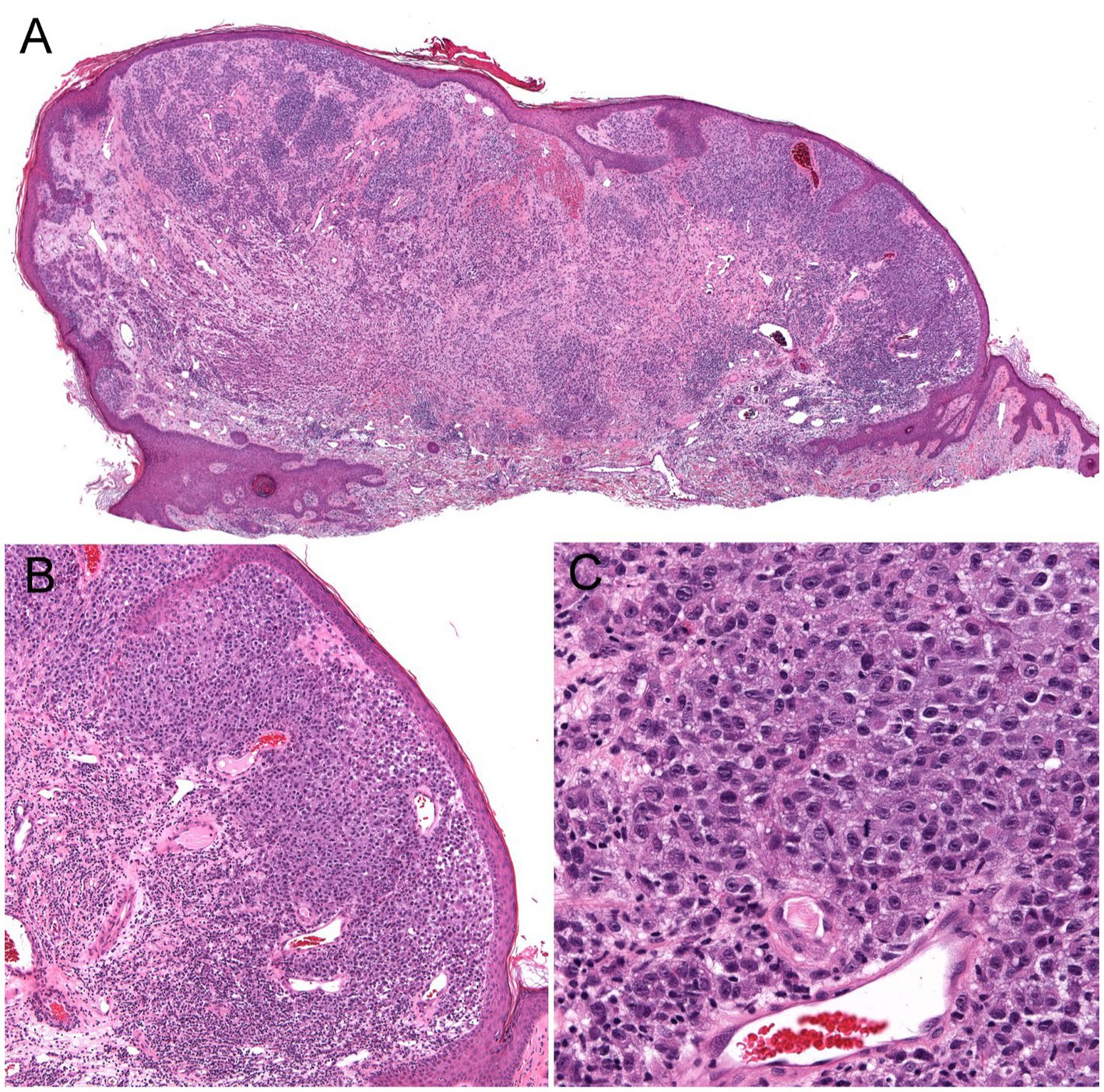
Figure 4 . Unsuspected nodular melanoma (Stage 2B) clinically diagnosed as an inflamed skin tag. (A) Polypoid asymmetrical melanocytic lesion with irregular distribution of melanocytes (40× magnification). (B) Melanocytes arranged in sheets in the superficial dermis (100× magnification). (C) Large atypical melanocytes that vary in size and shape with occasional mitotic figures (400× magnification).
Phenotype risk factors
Phenotype risk factors for developing melanoma includes Caucasian race with fair skin, high nevus count, giant congenital nevus, particularly garment or bathing trunk nevus, and Clark/dyplastic nevus ( 47 , 48 ). Caucasians have 20 times the risk of developing melanoma compared to Black people ( 3 ). Immunosuppression and prior history of melanoma also confer a higher risk.
Giant congenital nevus (>20 cm), particularly garment or bathing trunk nevi, has a significant life-time risk of developing melanoma, ranging from 2.3 to 14% ( 49 ). Though some giant congenital nevi may be amenable for prophylactic removal, the garment or bathing trunk nevi are usually too large to remove. Lifetime monitoring for development of melanoma and symptoms due to neurocutaneous melanocytosis is required for these patients.
Precursor lesion: the dysplastic nevus
In the paradigm of multistep progression of cancer, identification of a reliable precursor lesion is crucial for early detection and reducing the morbidity and mortality associated with the cancer.
In an attempt to follow the successful cervical and colon cancer model and assuming the linear multistep progression paradigm, identification of a precursor lesion for melanoma was sought by clinicians and researchers fighting the battle against melanoma. In 1978, Clark and colleagues described six melanoma prone families where they observed flat melanocytic nevi with irregular border and color variegation in majority of the family members who developed melanoma ( 28 ). The authors proposed that these nevi, referred to as B-K moles at the time, have a higher risk of transforming into melanoma. Shortly thereafter, without any formal evidence, these nevi, renamed as dysplastic nevi, received a stamp of approval in a NIH consensus conference of being a marker and precursor to melanoma ( 30 ). Over 40 years of practice of close scrutiny and their removal has not resulted in any convincing evidence of their association with melanoma ( 50 , 51 ). Many authors have concluded that the nevus may serve as a phenotype marker but not a precursor lesion to melanoma ( 50 – 54 ). Some have argued against their precursor status from the outset ( 55 ).
Genetics risk factors
Exciting advances have been made in the discoveries of the genetic underpinnings of cutaneous melanocytic neoplasms, benign and malignant. Germline mutations that significantly increases the life-time risk of developing melanoma include CDKN2A, CDK4, BAP1, TERT, MITF, MC1R, and POT1 ( Table 1 ) ( 56 ). These germline mutations underlie the familial or hereditary melanoma dominant syndromes, in which melanoma is the predominant cancer of the syndrome. Germline mutations that underlie melanoma subordinate or mixed cancer syndromes include PTEN, TP53, BRCA1, BRCA2, and XP A-G ( 57 ). In these syndromes, other cancers have a higher penetrance rate than melanoma.
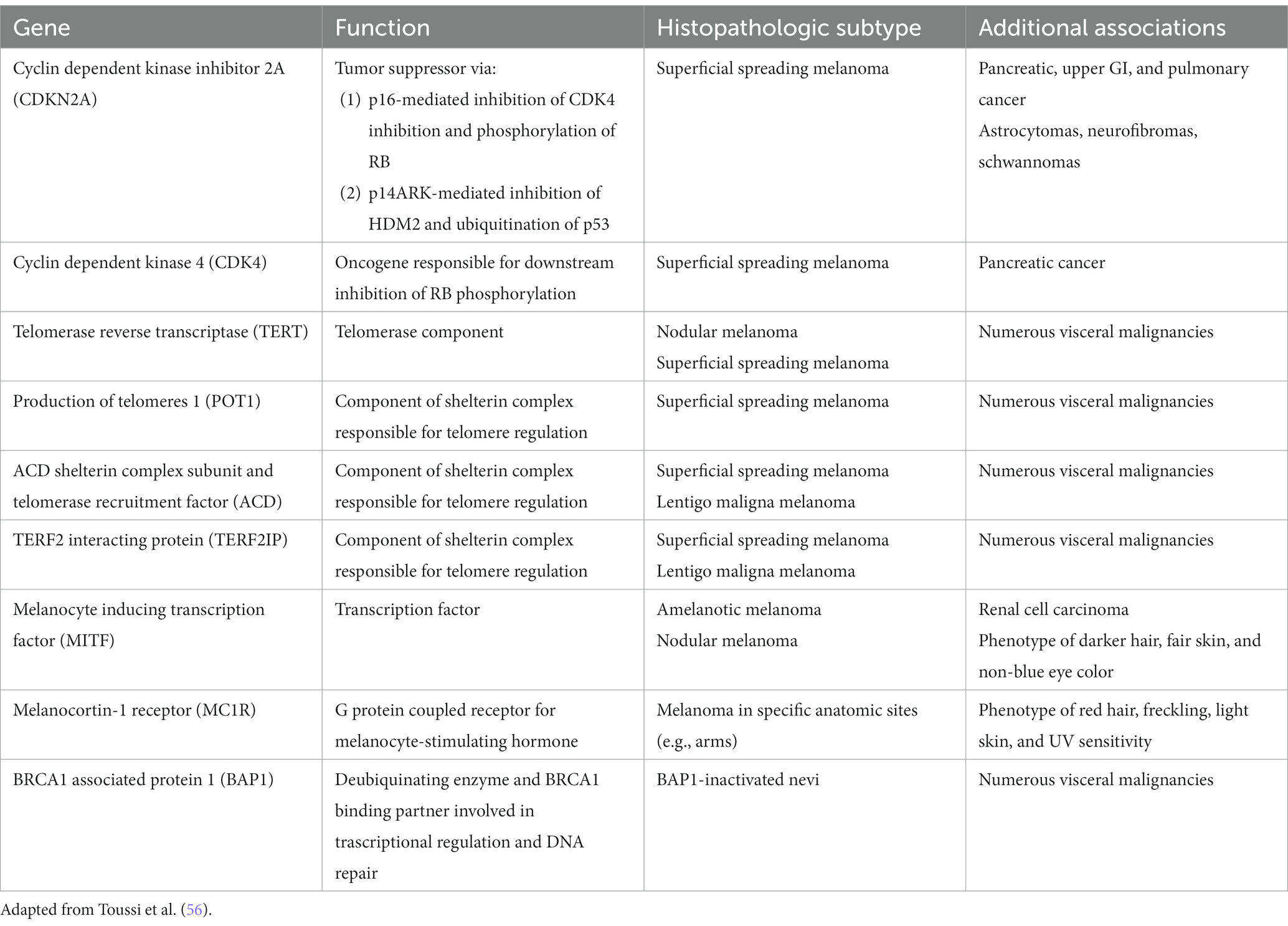
Table 1 . Germline mutations associated with increased melanoma risk.
While only 5–12% of melanomas are thought to be hereditary, approximately 40% of hereditary melanomas are attributable to CDKN2A mutations, making CDKN2A the most commonly mutated gene responsible for an autosomal dominant pattern of hereditary melanoma ( 58 ). Coding for tumor suppressors p16 and p14 (ARF) which regulate the cell cycle, patients with germline mutation in the CDKN2A gene have a high risk of developing melanoma, glioblastoma, and pancreatic carcinoma ( 57 ). Compared to the 2.6% lifetime risk of developing melanoma in the US White population, patients with germline CDKN2A mutation increases that risk to 28 to 76% depending on the presence of other factors. In one study, the risk of melanoma in CDKN2A mutation carriers was approximately 14% by age 50 years, 24% by age 70 years, and 28% by age 80 years ( 59 ). In comparison, as one of the mutations for the hereditary pancreatic syndromes, CDKN2A carriers confers a 17% lifetime risk of developing pancreatic cancer ( 60 ). Furthermore, while CDKN2A is common somatically mutated in sporadic melanoma, somatic biallelic inactivation of CDKN2A occurs exclusively within invasive melanoma ( 56 ). Therefore, the gene continues to represent an important mutational contributor to melanoma development both familial and sporadic.
First discovered in uveal melanoma, mutations in the BAP1 gene interfere with its function as a deubiquitinating enzyme and tumor suppressor ( 61 ). Malignancies associated with germline mutations in BAP1 include cutaneous melanoma, ocular melanoma, mesothelioma, renal cell carcinoma, and basal cell carcinoma ( 61 – 64 ). They also may develop small dome-shaped nevi with a spitzoid melanocytes that show loss of BAP1, referred to as BAP1 deficient nevus or Wiesner nevus ( 65 ). The vast majority of Wiesner nevus occurs sporadically, and, thus, genetic testing should be based on detailed patient’s history.
TERT, which encodes the telomerase reverse transcriptase subunit of the telomerase enzyme is another important predisposing mutation for the development of melanoma ( 66 ). Mutations in the TERT gene allow for the escape of premalignant cells from senesce and apoptosis, contributing to the development of malignancy. First identified in melanoma, TERT mutations have become increasingly identified as one of the most common noncoding mutations in all cancers. Importantly, somatic TERT promoter mutations portend poor prognostic factors, including a higher likelihood of increased tumor thickness and the presence of ulceration, high mitotic rate, and lymph node metastasis ( 56 , 66 ).
Gene testing for melanoma
Except for the CDKN2A gene, formal guidelines for genetic testing for mutations responsible for the hereditary melanomas do not exist ( 57 ). The “rules of two or three” apply to testing for CDKN2A mutation and not for others ( 67 ). Genetic test should be considered for history of three or more primary melanomas and/or pancreatic cancer in geographic areas of high melanoma prevalence and two or more primary melanomas or in situ melanomas in areas of low prevalence. History of invasive melanomas in multiple family members at ages earlier than 40 should raise the suspicion of hereditary melanoma syndrome. Leachman and coworkers have outlined more detailed suggestions of screening for germline mutations other than CDKN2A ( 67 ). Though technological advances in genomic analysis have enabled discovery of new mutations associated with melanoma and the ease of testing for gene mutations, the actual benefits of the testing and surveillance, in terms of outcomes, are not available.
One of the commonly tested genes is BRCA1 and BRCA2 mutations for evaluation of genetic basis of breast cancers. They both play a role in contributing to the repair of damaged DNA and the destruction of cells with irreparable DNA damage. Although BRCA1 mutations have failed to demonstrate an increased risk of melanoma, BRCA2 mutations have been linked to an increase incidence of melanoma in large breast and ovarian cancer families. An in-depth analysis of published data, however, showed insufficient evidence to warrant increased skin cancer surveillance in these patients without other risk factors ( 68 ).
Melanoma diagnosis
The current clinicopathologic classification of melanoma has been widely adopted and employed in clinical practice for its simplicity and ease of implementation. The classification consists of four major distinct clinicopathologic subtypes with its own corresponding in situ lesions: lentigo maligna, superficial spreading, acral lentiginous, and nodular ( 69 , 70 ). The classification relies heavily on the interpretation of the histopathologic findings, a highly subjective discipline with issues of interobserver reliability ( 71 – 74 ). Though there are melanomas that clearly match the clinical and pathological criteria of a given subtype, many have overlapping histopathologic patterns in the same lesion, making subtyping arbitrary ( 75 , 76 ). For example, acral lentiginous melanomas have a wide spectrum of histopathologic patterns that encompasses histopathologic patterns observed in the other three subtypes of melanomas. In addition, the classification does not intrinsically incorporate prognostic information. Instead, known extrinsic prognostic factors are added to the pathology report, primarily Breslow depth and ulceration that dictate management. The classification assumes a linear model of progression for all melanomas where melanoma in situ lesions are assumed to be the obligate early lesion that becomes invasive and subsequently metastasizes. Though progression of melanomas varies widely, all subtypes of melanomas are treated the same, driven primarily by the thickness of the melanoma.
Recently, the World Health Organization introduced a new classification of melanoma that includes epidemiologic and genomic information in addition to the clinicopathologic criteria. The classification has been expanded to 9 different subtypes that account for the very rare and mucosal melanomas ( Table 2 ) ( 77 ). This classification also assumes a linear model of progression in which the melanocytic nevus with the same driver mutation as the melanoma is proposed as the precursor lesion for each subtype. Except for the rare giant or garment congenital nevi where there is a known higher risk of developing melanoma within the nevus, evidence is lacking for the precursor model of progression. The rate at which the precursor nevus acquires the requisite mutations to transform into melanoma is unknown and the rare occurrences of these nevi make it hard to verify their precursor status. The long experience with Clark/dysplastic nevus has not supported the precursor model of melanoma progression ( 50 , 51 ).
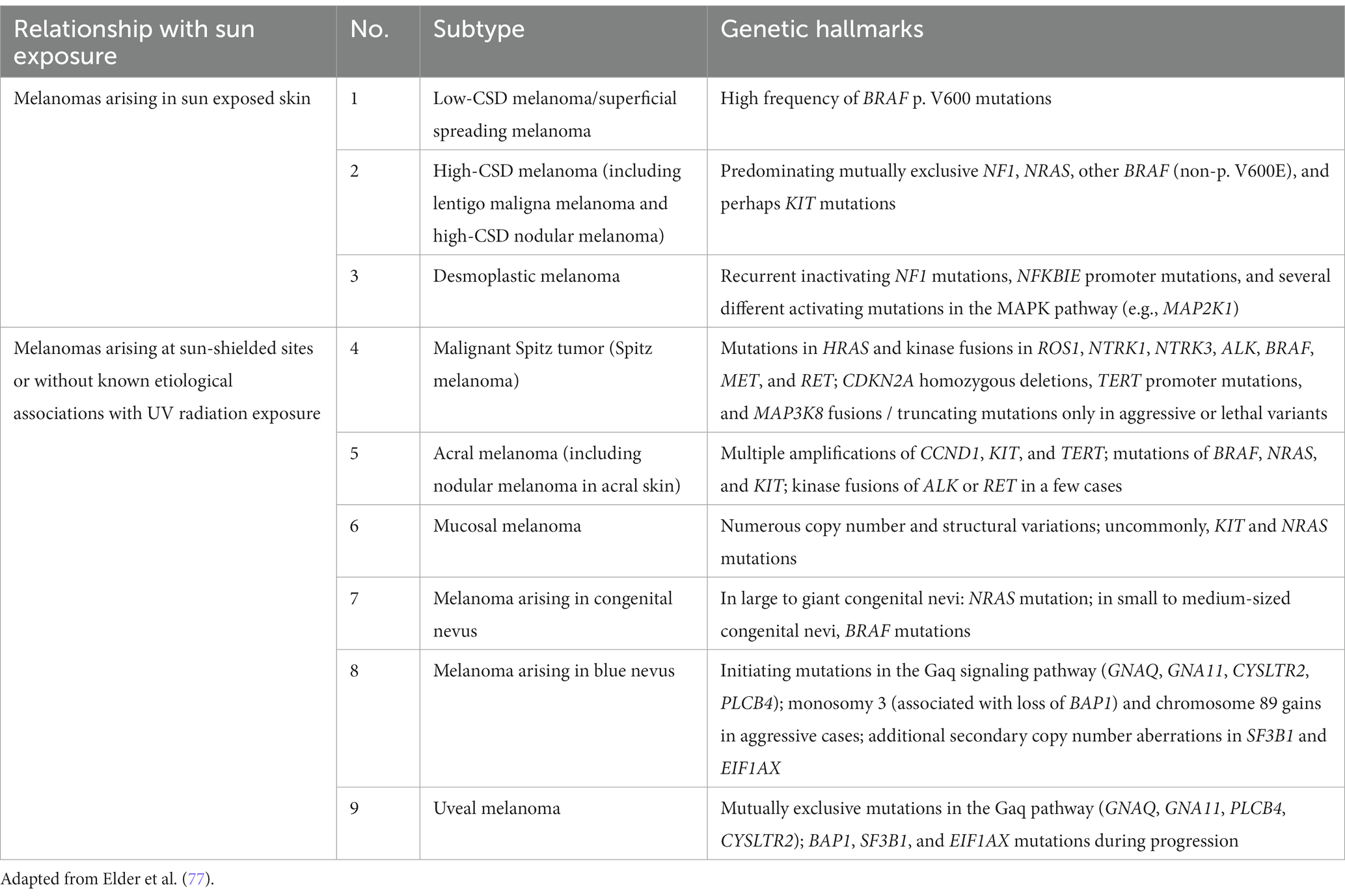
Table 2 . World Health Organization classification of melanoma.
Melanocytic pathology assessment tool and hierarchy for diagnosis (MPATH-Dx)
While guidelines have been established for melanomas, a standardized management guidelines have not been established for a large group of melanocytic neoplasms for reasons that have plagued the gold standard in diagnosing melanocytic neoplasms—interobserver reliability. In addition, the lack of standardized diagnostic terms for melanocytic neoplasms and disagreements about the fundamental nature of various melanocytic neoplasms have contributed to the confusion among clinicians and patients and the lack of standard management. The local and regional variation on the diagnostic term for the “B-K mole” first described by Clark and colleagues, which includes dysplastic nevus, atypical nevus, nevus with architectural disorder, and Clark nevus, exemplifies the issue of standardization of diagnostic terminology. In 2014, MPATH-Dx schema was introduced to simplify and standardize reporting of melanocytic neoplasms by bring about clarity to classification and management of melanocytic neoplasms, regardless of the different diagnostic terms used ( 78 ). The initial version MPATH-Dx consisted of 5 classes with benign and malignant diagnoses with minimal disagreement at the two ends of the classification hierarchy. In early 2023, a new version of MPATH-Dx was published after years of feedback that also accounts for the schema of 2018 WHO classification of melanocytic neoplasms ( Table 3 ) ( 79 ). The previous 5 class hierarchy has been simplified into four, essentially removing the original Class II by eliminating the moderately atypical nevus following the WHO classification and moving Spitz nevus into the new Class II group. The new Class I group, referred to as low grade, requires no further treatment while Class II group, referred to as high grade, requires further treatment, which includes a diverse spectrum of melanocytic lesions—high grade dysplastic or atypical nevus, cellular blue nevus, and melanoma in situ . As acknowledged by the authors, the MPATH-Dx schema does not escape the issues of inherent subjectivity of pathologic diagnosis of melanocytic neoplasms. While the concordance rates for the two ends of the diagnostic spectrum is good, the concordance rate is poor for thin melanomas, Spitz nevi, and grading of melanocytic lesions ( 74 ). In addition, many clinicians will find the margin recommendation of up to 1 cm for melanocytic lesions in Class II vague and arbitrary. Clinicians will look for more precise margin recommendations as 2-, 5-, and 10-millimeter margins make for significant differences in surgery and impact for patients depending on the site and patient’s age. Furthermore, there will be clinicians who will object to the recommendation of removal of routine Spitz, cellular blue, deep penetrating nevi that are included in Class II. Despite these limitations of the scheme, MPATH-Dx is working toward more precise classification, clarity in management, and standardization of reporting that are needed in the diagnostic pathway.
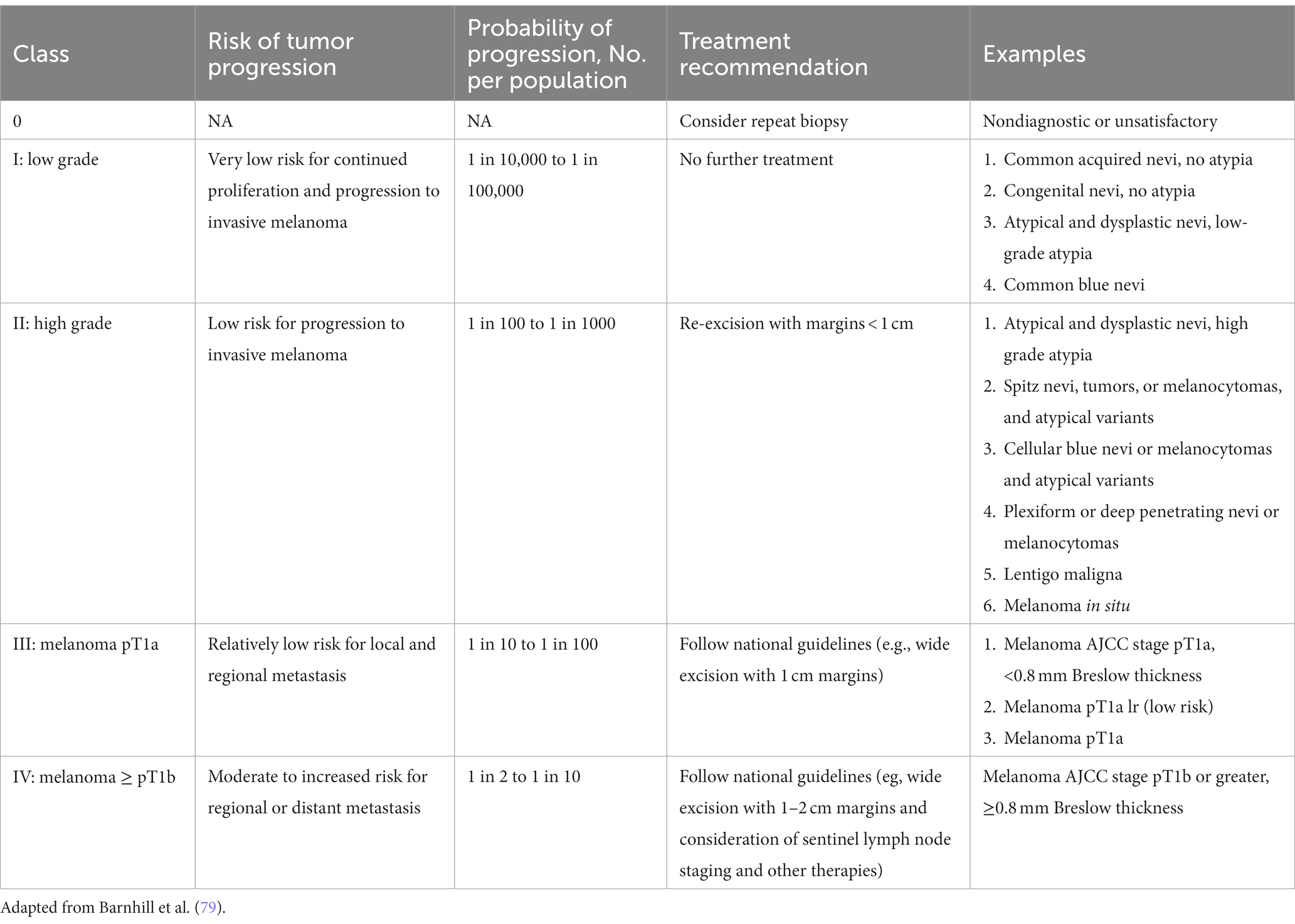
Table 3 . Melanocytic pathology assessment tool and hierarchy for diagnosis (MPATH-Dx).
Clinical diagnosis
The ABCD mnemonic has been a diagnostic aid in the early detection of melanoma since the 1980s, and the recent inclusion of “evolution” as an “E” criterion has been reported to increase its sensitivity. The ABCDE criteria refers to the presence of asymmetry, border irregularity, color variability, a diameter of 6 mm or greater, and evolution or recent change. With the incorporation of ABCDE criteria, diagnostic accuracy of naked-eye examination for melanoma has been estimated to be approximately 65% overall ( 80 – 82 ). The introduction of dermatoscopy has been a major change in the clinical diagnosis of melanocytic neoplasms at the bedside. While the diagnostic technique is not new, the availability of small handheld version of the device coincided with the widespread adoption of dermatoscopy in the modern era. The modern dermatoscope provides a light source, usually a 10× magnification and, more importantly, polarization, which renders the cornified layer translucent, allowing the visualization of subsurface structures ( 83 ). Whole new diagnostic criteria of subsurface structures have emerged in diagnosing a variety of inflammatory, infectious, and neoplastic disease of the skin, particularly early melanomas, one of the major objectives of dermatoscopy. Various dermatoscopic criteria have been developed for benign and malignant melanocytic neoplasms. The presence of an atypical pigment network, blue-white veil, atypical vascular patterns, and irregular streaks, pigmentation, globules, or regression structures on dermatoscopy have a higher association with melanoma ( 84 ). Diagnostic algorithms that have been developed include the 7-point checklist, Menzies method, and the CASH criteria ( 84 ). Each of these algorithms has been shown to increase sensitivity and specificity in melanoma identification. Meta-analyses of large studies have suggest up to 18 and 10% increase in the sensitivity and specificity in the diagnosis of melanoma, respectively ( 85 – 87 ). Major limitations of the studies were that most were retrospective in design evaluated by a group of experts in the field involving images of already managed melanocytic lesions with no impact on management. Accordingly, the more recent Cochrane review ( 88 ) concluded that the evidence base of dermatoscopy is limited and “when used by specialists , dermoscopy is better at diagnosing melanoma compared to inspection of a suspicious skin lesion using the naked eye alone.” Conclusive evidence was lacking to “explicitly estimate the sensitivity and specificity of dermoscopy, either with or without visual inspection.” Furthermore, despite the enthusiasm and widespread adoption of the diagnostic technique, evidence of desired impact in terms of decreased biopsy rates, cost savings, and improved outcomes of patients are not available. The words of late Carli from 2007 hold true today: “Dermoscopy not yet shown to increase sensitivity of melanoma diagnosis in real practice.” ( 89 ) Further studies are needed to validate the widespread use for the diagnosis of melanomas, particularly in the general dermatology and primary care settings.
Recently, a tape-strip test, Pigmented Lesion Assay (DermTech, La Jolla, CA), has been introduced that analyzes the RNA from the stratum corneum for expression levels of RNA Linc00518 (Linc) and PRAME, which are overexpressed in melanomas. The assay also provides the status of TERT, a frequent somatic driver mutation in melanoma. In limited number of studies, the test boasts a greater than 99% negative predictive value, greater than 91% sensitivity, and 70% specificity in the diagnosis of melanoma ( 90 – 92 ). The test has not been widely in use and its role has not been studied extensively. In theory the high negative predictive value should provide reassurance to monitor the pigmented lesion with a negative test. The test also has been promoted to be useful in cosmetically sensitive or difficult to biopsy areas of the skin. Further independent studies and experience are needed to determine whether the promising results are reproducible and the exact role of the test.
Artificial intelligence
Artificial intelligence (AI) is expected have a profound impact on practice of medicine in the coming years, particularly perceptual specialties such as radiology, pathology, and dermatology as significant advances have been made in image recognition AI algorithms. Jaffe et al. evaluated an AI algorithm that was able to sift through 1,550 images of suspicious and benign skin lesions and identify melanoma with a sensitivity of 100% ( 93 ). Importantly, the specificity of the algorithm was found to be 64.8% which was only slightly less than the 69.9% specificity of clinicians. Other studies have shown that artificial intelligence is able to perform similarly to dermatoscopic evaluation in the identification of melanoma ( 94 ).
Currently there are no Food and Drug Administration (FDA) approved or cleared AI products in the US for the diagnosis or for the triage purposes of pigmented lesions. The number of commercially available smartphone applications, however, is rapidly growing. Because the diagnostic accuracy of the applications has been inconsistent and unreliable, several reviews have recommended against their use ( 95 – 97 ).
DermAssist, developed by Google, has been CE-marked as a Class 1 Medical Device in Europe with potential for worldwide expansion. The deep learning system within DermAssist was found to be non-inferior compared to 6 dermatologists and superior to 12 primary care physicians and nurse practitioners in providing a diagnosis for a set of 26 common skin conditions ( 98 ). When allowed to provide a three-diagnosis differential, the deep learning system was able to achieve a sensitivity of 90% compared to 89% in the dermatologist group and 69 and 72% in the primary care physician and nurse practitioner groups, respectively ( 98 ).
As the databases of clinical, dermatoscopic, and histologic images for melanocytic lesions grow, validated training data sets that are more generalizable are expected to emerge, setting the stage for AI augmented practice of dermatology. It is important to note that 90% of the databases used to create the DermAssist software extracted images from patients with lighter skin types; therefore, concerns regarding bias and equal access to the benefits of AI remain to be addressed, particularly in regard to skin of color ( 99 ).
Histopathological diagnosis
Despite the emergence of sophisticated molecular based tests, histopathological diagnosis of melanoma still remains the gold standard. The inherent subjective nature of histologic diagnosis of melanocytic neoplasms has resulted in high rate of discordance among pathologists ( 71 – 74 ). While thick bulky melanomas usually pose no issues, biopsies of ever smaller and thin lesions have further highlighted the problem of interobserver reliability. In the largest iteration of concordance study of melanocytic neoplasms among pathologists, only 25% concordance rate was observed for Spitz and atypical nevi and 45% concordance rate was observed for atypical spitz tumor, severely atypical nevi, and melanoma in situ , rates that are unacceptably low to be a valid diagnostic test ( 74 ). The low concordance rate among pathologists indicates markedly different thresholds are being applied to a large group of melanocytic neoplasms that have a significant impact on management. With the advances in molecular diagnostics, pathologists are turning to more precise molecular based ancillary diagnostic tests.
Historically, immunohistochemical stains had minimal diagnostic role, having only the confirmatory role of classifying the neoplasm as melanocytic. With insights on molecular signatures of melanomas, several immunohistochemical stains with a more diagnostic role have become available that include PReferentially expressed Antigen in Melanoma (PRAME) and p16. PRAME is overexpressed in melanomas and other cancers. Reflective of its discriminator power, it is included in several gene expression profiling tests for the prognostication of uveal melanoma (Decision Dx-UM), diagnosis of melanoma (myPath Melanoma), and guidance on the decision to biopsy (DermTech). Sensitivity ranging from 67–94% has been reported using PRAME IHC for the diagnosis of melanoma ( 100 – 104 ). For spindle cell desmoplastic melanomas, S100 and SOX10 continues to play a key role in their diagnosis as lower sensitivity ranging from 20 to 35% was observed ( 104 , 105 ). Other useful IHC stains in the diagnosis of melanoma include p16. The loss of p16 expression, the product of CDKN2A gene, strongly correlates with the diagnosis of melanoma that can be demonstrated with the available IHC stain ( 106 ). Differentiating Spitz nevus from spitzoid melanoma, however, is not always helpful ( 107 ). Immunohistochemical stains have emerged for detecting the status of BRAF, BAP1, and cKit for the guidance role in targeted therapies.
Molecular diagnostic tests
Current molecular diagnostic tests for melanoma available to pathologists include comparative genomic hybridization (CGH), fluorescence in situ hybridization (FISH), gene expression profiling (GEP). CGH identifies chromosomal copy number variations, including the deletion or multiplications of chromosomal segments ( 108 ). The technique involves DNA labeling with fluorochromes that subsequently allow for comparison with reference DNA to highlight genomic areas with gains or losses of DNA material. Advent of single-nucleotide polymorphism arrays allows for targeting genetic loci to the resolution of specific point mutations within the genome of melanocytes, allowing for the identification of loss of heterozygosity, even in chromosomal copy-neutral mutations which are missed with traditional CGH ( 109 ). Early CGH studies demonstrated that over 95% of melanomas demonstrated chromosomal number abnormalities in contrast to only 13% of benign nevi ( 109 , 110 ). More recent studies have demonstrated the utility of CGH in differentiating melanoma from traditionally diagnostically challenging melanocytic entities, such as cellular blue nevi and Spitz nevi. Spitz nevi have been shown to demonstrate only isolated chromosomal number abnormalities at limited loci, while spitzoid melanomas demonstrate multiple copy number abnormalities in various segments ( 110 ).
In contrast to CGH that analyzes the whole genome, FISH allows for visualization of gains and losses of specific genomic segments. As normal somatic cells are expected to have two copies of any specific chromosome or chromosomal segment, the presence of more or less than two fluorescent signals indicates the presence of a chromosomal number abnormality. FISH has shown promising results in the differentiation of unequivocal lesions, including conjunctival nevi, epithelioid blue nevi, and, in particular Spitz nevi, and their respective melanoma counterparts with reported sensitivity of 83% and specificity of 94% ( 111 ).
While the original FISH assay utilized four probes targeting 6p25 (RREB1), 6q23 (MYB), 11q13 (cyclin D1), and Cep6, two additional probes targeting CDKN2A (9p21) and MYC (8q24) were added, which has increased sensitivity and specificity to 94 and 98%, respectively ( 112 ). Although FISH offers a greater ease-of-use and less tissue and labor requirements, CGH has been found to be more sensitive and specific given its ability to assay the entire genome. The high cost and false-positives are additional shortcomings of FISH assays, particularly in lesions that demonstrate polyploidy, such as Spitz nevi which can demonstrate tetraploidy, thus triggering a false positive result ( 110 ).
Leveraging real-time reverse transcription-polymerase chain reaction (qRT-PCR) technology, myPath (Castle Biosciences, Friendswood, Texas), a GEP test, has become available for pathologists to aid in the diagnosis of ambiguous melanocytic neoplasms. The assay analyzes the expression of 23 genes that includes PRAME and S100. The assay returns a numerical score that corresponds to likely benign , likely malignant or likely indeterminate . Retrospective validation studies have yielded sensitivity and specificity as high as 94 and 96%, respectively, in unambiguous melanocytic lesions ( 113 – 115 ). A significantly lower sensitivity in the 50% range was observed in studies in which ambiguous melanocytic lesions were evaluated ( 116 , 117 ). Larger prospective studies on ambiguous melanocytic neoplasms are needed to demonstrate the utility and reliability of the ambiguous lesions for which the test was intended.
Artificial intelligence augmented practice of dermatopathology is in its nascent stage. Several studies suggesting that performance AI is equal to or better than experienced pathologists have been published in the diagnosis of melanoma in artificial study settings ( 118 – 123 ). To harness the potential of AI in dermatopathology, some barriers need to be solved. Application of AI requires digitalization of slides, cost of which have prevented most laboratories adopting digitalization of the laboratory workflow. Generalizability of results requires large, validated data training sets. Most published studies use proprietary small data training sets that may not be generalizable. Lastly, AI cannot solve the issue of diagnostic discordance issue among dermatopathologists for melanocytic lesions, which will continue to be a barrier in the training and application of AI models ( 124 ).
Prognosis and staging
Clinician have long relied on American Joint Committee (AJCC) on Cancer staging guidelines. The 8th edition of the AJCC melanoma staging system was implemented in 2018. The primary determinant of the localized stage is the Breslow depth of the melanoma and ulceration. Breslow depth is measured from the granular layer of the epidermis down to the greatest depth of the melanoma. One of the biggest changes from the 7th edition is the change in the definition of T1a and T1b. While the cutoff for T1a and T1b stage was ≤ 1 mm in the 7th edition, it was lowered to < 0.8 mm in the 8th edition ( 125 ). The result of the change directed more patients to a sentinel biopsy. The full impact of directing more patients for a sentinel biopsy in not known, but the rate of sentinel node positivity appears unchanged in one population-based study ( 126 ). A more individualized approach was suggested that accounted for clinicopathologic and molecular features.
Based on qRT-PCR technology that can be performed on paraffin embedded sections, several prognostic gene expression profiling assay tests have become available ( 127 ). In the US, 31-gene profiling assay (DecisionDx-Melanoma by Castle Biosciences) has been developed to provide prognostic risk stratification independent of the AJCC staging system. The assay predicts the risk of recurrence or metastasis in stage I, II, and III melanoma. The risk stratification scores consist of 1A (low risk), 1B/2A (intermediate risk) and 2A (high risk) ( 128 ). Multiple studies have consistently reported that the assay results independently predict metastatic risk, highlighting the utility of the GEP test ( 128 – 131 ).
While clinicians believe that GEP testing may have clinical benefit for patients with stage II and IIIA disease, the controversy has been for testing patients with stage I disease, a group with a very low risk of recurrence and metastasis ( 128 ). As stage I disease make up over 70% of new cases melanoma each year in the US, the test has the potential for high utilization for this stage of the disease, which has raised concerns about the high cost of the test ($7,193 per test) with unknown clinical benefit at this time ( 132 ). Meta-analysis by Marchetti and coworkers have concluded the performance of GEP tests for stage I disease was poor and highlighted the potential harm for patients in this group ( 127 , 133 ). More recently, Kangas-Dick et al. reported that the GEP test did not perform better than traditional clinicopathologic prognostic features in predicting melanoma recurrence risk ( 134 ). Proponents and opponents of the GEP test all have criticized the methodologies of the studies that oppose their stance. Consensus statements for and against the test have been published ( 128 , 133 , 135 ). Currently, the American Academy of Dermatology (AAD) and National Comprehensive Cancer Network (NCCN) do not endorse the routine use of GEP. To settle the issue of validity and clinical applicability, authors have recommended prospective randomized clinical trial with predetermined end points free of industry sponsorship bias ( 128 , 132 , 133 , 136 ).
The remarkable advances in understanding the genomic underpinning of melanoma are paving the road for a paradigm shift in the approach to melanoma classification, diagnosis, staging, and therapy. The more precise, objective-based classification and diagnosis of melanoma are expected to replace the clinicopathologic one that is currently widely in use. Molecular diagnostics will play a greater role in the clinical and histologic diagnosis of melanoma as validated, convenient, and cost-effective molecular-based tests are expected to emerge. AI augmented clinical and histopathologic diagnosis of melanoma is expected to make the process more streamlined, precise, and efficient. The next iteration of AJCC staging will better reflect the rapid advances molecular basis of prognostication that is expected to be incorporated. The one issue that needs more immediate attention from the dermatology community is overdiagnosis. Though there is no debate on whether the overdiagnosis of melanoma exists, there is debate as to the degree. Epidemiologic evidence all but indicates a significant degree of overdiagnosis, providing a compelling reason for a shift in strategy from the current approach to melanoma detection. The immediate need is to identify the small fraction of aggressive melanomas within the sea of indolent early melanomas that are being detected today. The resolution of this knowledge gap requires appropriate attention both in terms of funding and research.
Author contributions
SW: Writing – original draft, Writing – review & editing, Data curation, Formal analysis. JL: Conceptualization, Supervision, Writing – original draft, Writing – review & editing.
The author(s) declare that no financial support was received for the research, authorship, and/or publication of this article.
Conflict of interest
The authors declare that the research was conducted in the absence of any commercial or financial relationships that could be construed as a potential conflict of interest.
Publisher’s note
All claims expressed in this article are solely those of the authors and do not necessarily represent those of their affiliated organizations, or those of the publisher, the editors and the reviewers. Any product that may be evaluated in this article, or claim that may be made by its manufacturer, is not guaranteed or endorsed by the publisher.
1. Arnold, M, Singh, D, Laversanne, M, Vignat, J, Vaccarella, S, Meheus, F, et al. Global burden of cutaneous melanoma in 2020 and projections to 2040. JAMA Dermatol . (2022) 158:495–503. doi: 10.1001/jamadermatol.2022.0160
PubMed Abstract | CrossRef Full Text | Google Scholar
2. Siegel, RL, Miller, KD, Fuchs, HE, and Jemal, A. Cancer statistics, 2022. CA Cancer J Clin . (2022) 72:7–33. doi: 10.3322/caac.21708
CrossRef Full Text | Google Scholar
3. SEER. (n.d.) Melanoma of the Skin – Cancer stat facts. SEER web site. Available at: https://seer.cancer.gov/statfacts/html/melan.html (Accessed July 9, 2023)
Google Scholar
4. Landow, SM, Gjelsvik, A, and Weinstock, MA. Mortality burden and prognosis of thin melanomas overall and by subcategory of thickness, SEER registry data, 1992-2013. J Am Acad Dermatol . (2017) 76:258–63. doi: 10.1016/j.jaad.2016.10.018
5. American Cancer Society. (2023) Melanoma skin Cancer statistics. Available at: https://www.cancer.org/cancer/types/melanoma-skin-cancer/about/key-statistics.html (Accessed July 9, 2023)
6. Olsen, CM, Thompson, JF, Pandeya, N, and Whiteman, DC. Evaluation of sex-specific incidence of melanoma. JAMA Dermatol . (2020) 156:553–60. doi: 10.1001/jamadermatol.2020.0470
7. Kurtansky, NR, Dusza, SW, Halpern, AC, Hartman, RI, Geller, AC, Marghoob, AA, et al. An epidemiologic analysis of melanoma Overdiagnosis in the United States, 1975-2017. J Invest Dermatol . (2022) 142:1804–1811.e6. doi: 10.1016/j.jid.2021.12.003
8. Ackerman, AB. Macular and patch lesions of malignant melanoma: malignant melanoma in situ . J Dermatol Surg Oncol . (1983) 9:615–8. doi: 10.1111/j.1524-4725.1983.tb00868.x
9. Menzies, SW, and Zalaudek, I. Why perform dermoscopy? The evidence for its role in the routine management of pigmented skin lesions. Arch Dermatol . (2006) 142:1211–2. doi: 10.1001/archderm.142.9.1211
10. Ring, C, Cox, N, and Lee, JB. Dermatoscopy. Clin Dermatol . (2021) 39:635–42. doi: 10.1016/j.clindermatol.2021.03.009
11. Burton, RC, and Armstrong, BK. Recent incidence trends imply a nonmetastasizing form of invasive melanoma. Melanoma Res . (1994) 4:107–13. doi: 10.1097/00008390-199404000-00005
12. Welch, HG, Mazer, BL, and Adamson, AS. The rapid rise in cutaneous melanoma diagnoses. N Engl J Med . (2021) 384:72–9. doi: 10.1056/NEJMsb2019760
13. Glasziou, PP, Jones, MA, Pathirana, T, Barratt, AL, and Bell, KJ. Estimating the magnitude of cancer overdiagnosis in Australia. Med J Aust . (2020) 212:163–8. doi: 10.5694/mja2.50455
14. Whiteman, DC, Olsen, CM, MacGregor, S, Law, MH, Thompson, B, Dusingize, JC, et al. The effect of screening on melanoma incidence and biopsy rates. Br J Dermatol . (2022) 187:515–22. doi: 10.1111/bjd.21649
15. Adamson, AS, Suarez, EA, and Welch, HG. Estimating Overdiagnosis of melanoma using trends among black and white patients in the US. JAMA Dermatol . (2022) 158:426–31. doi: 10.1001/jamadermatol.2022.0139
16. Carter, SM, Degeling, C, Doust, J, and Barratt, A. A definition and ethical evaluation of overdiagnosis. J Med Ethics . (2016) 42:705–14. doi: 10.1136/medethics-2015-102928
17. Brodersen, J, Schwartz, LM, Heneghan, C, O’Sullivan, JW, Aronson, JK, and Woloshin, S. Overdiagnosis: what it is and what it isn’t. BMJ Evid Based Med . (2018) 23:1–3. doi: 10.1136/ebmed-2017-110886
18. Lipsker, DM, Hedelin, G, Heid, E, Grosshans, EM, and Cribier, BJ. Striking increase of thin melanomas contrasts with stable incidence of thick melanomas. Arch Dermatol . (1999) 135:1451–6. doi: 10.1001/archderm.135.12.1451
19. Croswell, JM, Ransohoff, DF, and Kramer, BS. Principles of cancer screening: lessons from history and study design issues. Semin Oncol . (2010) 37:202–15. doi: 10.1053/j.seminoncol.2010.05.006
20. Vecchiato, A, Zonta, E, Campana, L, Dal Bello, G, Rastrelli, M, Rossi, CR, et al. Long-term survival of patients with invasive ultra-thin cutaneous melanoma: a single-center retrospective analysis. Medicine (Baltimore) . (2016) 95:e2452. doi: 10.1097/MD.0000000000002452
21. Eguchi, MM, Elder, DE, Barnhill, RL, Piepkorn, MW, Knezevich, SR, Elmore, JG, et al. Prognostic modeling of cutaneous melanoma stage I patients using cancer registry data identifies subsets with very-low melanoma mortality. Cancer . (2023) 129:89–97. doi: 10.1002/cncr.34490
22. Greenlee, RT, Murray, T, Bolden, S, and Wingo, PA. Cancer statistics, 2000. CA Cancer J Clin . (2000) 50:7–33. doi: 10.3322/canjclin.50.1.7
23. Siegel, RL, Miller, KD, Fuchs, HE, and Jemal, A. Cancer statistics, 2021. CA Cancer J Clin . (2021) 71:7–33. doi: 10.3322/caac.21654
24. Olsen, CM, Pandeya, N, Rosenberg, PS, and Whiteman, DC. Incidence of in situ vs invasive melanoma: testing the “obligate precursor” hypothesis. J Natl Cancer Inst . (2022) 114:1364–70. doi: 10.1093/jnci/djac138
25. Elder, DE. Obligate and potential precursors of melanoma. J Natl Cancer Inst . (2022) 114:1320–2. doi: 10.1093/jnci/djac139
26. Patel, VR, Roberson, ML, Pignone, MP, and Adamson, AS. Risk of mortality after a diagnosis of melanoma in situ. JAMA Dermatol . (2023) 159:e231494:703–10. doi: 10.1001/jamadermatol.2023.1494
27. Esserman, LJ, Thompson, IM, and Reid, B. Overdiagnosis and overtreatment in cancer: an opportunity for improvement. JAMA . (2013) 310:797–8. doi: 10.1001/jama.2013.108415
28. Clark, WH, Reimer, RR, Greene, M, Ainsworth, AM, and Mastrangelo, MJ. Origin of familial malignant melanomas from heritable melanocytic lesions. ‘The B-K mole syndrome’. Arch Dermatol . (1978) 114:732–8.
29. Consensus conference: precursors to malignant melanoma. JAMA . (1984) 251:1864–6. doi: 10.1001/jama.1984.03340380046022
30. NIH Consensus conference. Diagnosis and treatment of early melanoma. JAMA . (1992) 268:1314–9. doi: 10.1001/jama.1992.03490100112037
31. Kanzler, MH, and Mraz-Gernhard, S. Primary cutaneous malignant melanoma and its precursor lesions: diagnostic and therapeutic overview. J Am Acad Dermatol . (2001) 45:260–76. doi: 10.1067/mjd.2001.116239
32. Mangione, CM, Barry, MJ, Nicholson, WK, Chelmow, D, Coker, TR, Davis, EM, et al. Screening for skin Cancer: US preventive services task force recommendation statement. JAMA . (2023) 329:1290–5. doi: 10.1001/jama.2023.4342
33. Breitbart, EW, Waldmann, A, Nolte, S, Capellaro, M, Greinert, R, Volkmer, B, et al. Systematic skin cancer screening in northern Germany. J Am Acad Dermatol . (2012) 66:201–11. doi: 10.1016/j.jaad.2010.11.016
34. Boniol, M, Autier, P, and Gandini, S. Melanoma mortality following skin cancer screening in Germany. BMJ Open . (2015) 5:e008158. doi: 10.1136/bmjopen-2015-008158
35. Stang, A, and Jöckel, K. Does skin cancer screening save lives? A detailed analysis of mortality time trends in Schleswig-Holstein and Germany. Cancer . (2016) 122:432–7. doi: 10.1002/cncr.29755
36. Bibbins-Domingo, K, Grossman, DC, Curry, SJ, Barry, MJ, Davidson, KW, Doubeni, CA, et al. Screening for thyroid Cancer: US preventive services task force recommendation statement. JAMA . (2017) 317:1882–7. doi: 10.1001/jama.2017.4011
37. SEER. (n.d.) Cancer of the Thyroid – Cancer stat facts. SEER web site. Available at: https://seer.cancer.gov/statfacts/html/thyro.html (Accessed July 9, 2023).
38. Adamson, AS. The USPSTF I statement on skin Cancer screening-not a disappointment but an opportunity. JAMA Dermatol . (2023) 159:579–81. doi: 10.1001/jamadermatol.2023.0706
39. Fears, TR, Scotto, J, and Schneiderman, MA. Mathematical models of age and ultraviolet effects on the incidence of skin cancer among whites in the United States. Am J Epidemiol . (1977) 105:420–7. doi: 10.1093/oxfordjournals.aje.a112400
40. Holman, CD, Armstrong, BK, and Heenan, PJ. Relationship of cutaneous malignant melanoma to individual sunlight-exposure habits. J Natl Cancer Inst . (1986) 76:403–14.
PubMed Abstract | Google Scholar
41. An, S, Kim, K, Moon, S, Ko, K-P, Kim, I, Lee, JE, et al. Indoor tanning and the risk of overall and early-onset melanoma and non-melanoma skin Cancer: systematic review and Meta-analysis. Cancers (Basel) . (2021) 13:5940. doi: 10.3390/cancers13235940
42. Gandini, S, Sera, F, Cattaruzza, MS, Pasquini, P, Picconi, O, Boyle, P, et al. Meta-analysis of risk factors for cutaneous melanoma: II Sun exposure. Eur J Cancer . (2005) 41:45–60. doi: 10.1016/j.ejca.2004.10.016
43. Lipsker, D, Engel, F, Cribier, B, Velten, M, and Hedelin, G. Trends in melanoma epidemiology suggest three different types of melanoma. Br J Dermatol . (2007) 157:338–43. doi: 10.1111/j.1365-2133.2007.08029.x
44. Martorell-Calatayud, A, Nagore, E, Botella-Estrada, R, Scherer, D, Requena, C, Serra-Guillén, C, et al. Defining fast-growing melanomas: reappraisal of epidemiological, clinical, and histological features. Melanoma Res . (2011) 21:131–8. doi: 10.1097/CMR.0b013e328342f312
45. Argenziano, G, Kittler, H, Ferrara, G, Rubegni, P, Malvehy, J, Puig, S, et al. Slow-growing melanoma: a dermoscopy follow-up study. Br J Dermatol . (2010) 162:267–73. doi: 10.1111/j.1365-2133.2009.09416.x
46. Hermes, HM, Sahu, J, Schwartz, LR, and Lee, JB. Clinical and histologic characteristics of clinically unsuspected melanomas. Clin Dermatol . (2014) 32:324–30. doi: 10.1016/j.clindermatol.2013.10.003
47. Rhodes, AR, Weinstock, MA, Fitzpatrick, TB, Mihm, MC, and Sober, AJ. Risk factors for cutaneous melanoma. A practical method of recognizing predisposed individuals. JAMA . (1987) 258:3146–54. doi: 10.1001/jama.1987.03400210088032
48. Holly, EA, Kelly, JW, Shpall, SN, and Chiu, SH. Number of melanocytic nevi as a major risk factor for malignant melanoma. J Am Acad Dermatol . (1987) 17:459–68. doi: 10.1016/s0190-9622(87)70230-8
49. Krengel, S, Hauschild, A, and Schäfer, T. Melanoma risk in congenital melanocytic naevi: a systematic review. Br J Dermatol . (2006) 155:1–8. doi: 10.1111/j.1365-2133.2006.07218.x
50. Duffy, K, and Grossman, D. The dysplastic nevus: from historical perspective to management in the modern era: part II. Molecular aspects and clinical management. J Am Acad Dermatol . (2012) 67:19.e1–19.e32. doi: 10.1016/j.jaad.2012.03.013
51. Spaccarelli, N, Drozdowski, R, Peters, MS, and Grant-Kels, JM. Dysplastic nevus part II: dysplastic nevi: molecular/genetic profiles and management. J Am Acad Dermatol . (2023) 88:13–20. doi: 10.1016/j.jaad.2022.05.071
52. Kittler, H, and Tschandl, P. Dysplastic nevus: why this term should be abandoned in dermatoscopy. Dermatol Clin . (2013) 31:579–88, viii. doi: 10.1016/j.det.2013.06.009
53. Rosendahl, CO, Grant-Kels, JM, and Que, SKT. Dysplastic nevus: fact and fiction. J Am Acad Dermatol . (2015) 73:507–12. doi: 10.1016/j.jaad.2015.04.029
54. Lozeau, DF, Farber, MJ, and Lee, JB. A nongrading histologic approach to Clark (dysplastic) nevi: a potential to decrease the excision rate. J Am Acad Dermatol . (2016) 74:68–74. doi: 10.1016/j.jaad.2015.09.030
55. Ackerman, AB. What naevus is dysplastic, a syndrome and the commonest precursor of malignant melanoma? A riddle and an answer. Histopathology . (1988) 13:241–56. doi: 10.1111/j.1365-2559.1988.tb02036.x
56. Toussi, A, Mans, N, Welborn, J, and Kiuru, M. Germline mutations predisposing to melanoma. J Cutan Pathol . (2020) 47:606–16. doi: 10.1111/cup.13689
57. Leachman, SA, Lucero, OM, Sampson, JE, Cassidy, P, Bruno, W, Queirolo, P, et al. Identification, genetic testing, and management of hereditary melanoma. Cancer Metastasis Rev . (2017) 36:77–90. doi: 10.1007/s10555-017-9661-5
58. Ransohoff, KJ, Jaju, PD, Tang, JY, Carbone, M, Leachman, S, and Sarin, KY. Familial skin cancer syndromes: increased melanoma risk. J Am Acad Dermatol . (2016) 74:423–36. doi: 10.1016/j.jaad.2015.09.070
59. Begg, CB, Orlow, I, Hummer, AJ, Armstrong, BK, Kricker, A, Marrett, LD, et al. Lifetime risk of melanoma in CDKN2A mutation carriers in a population-based sample. J Natl Cancer Inst . (2005) 97:1507–15. doi: 10.1093/jnci/dji312
60. Vasen, HF, Gruis, NA, Frants, RR, van Der Velden, PA, Hille, ET, and Bergman, W. Risk of developing pancreatic cancer in families with familial atypical multiple mole melanoma associated with a specific 19 deletion of p16 (p16-Leiden). Int J Cancer . (2000) 87:809–11.
61. Wiesner, T, Obenauf, AC, Murali, R, Fried, I, Griewank, KG, Ulz, P, et al. Germline mutations in BAP1 predispose to melanocytic tumors. Nat Genet . (2011) 43:1018–21. doi: 10.1038/ng.910
62. Farley, MN, Schmidt, LS, Mester, JL, Peña-Llopis, S, Pavia-Jimenez, A, Christie, A, et al. A novel germline mutation in BAP1 predisposes to familial clear-cell renal cell carcinoma. Mol Cancer Res . (2013) 11:1061–71. doi: 10.1158/1541-7786.MCR-13-0111
63. Abdel-Rahman, MH, Pilarski, R, Cebulla, CM, Massengill, JB, Christopher, BN, Boru, G, et al. Germline BAP1 mutation predisposes to uveal melanoma, lung adenocarcinoma, meningioma, and other cancers. J Med Genet . (2011) 48:856–9. doi: 10.1136/jmedgenet-2011-100156
64. de la Fouchardière, A, Cabaret, O, Savin, L, Combemale, P, Schvartz, H, Penet, C, et al. Germline BAP1 mutations predispose also to multiple basal cell carcinomas. Clin Genet . (2015) 88:273–7. doi: 10.1111/cge.12472
65. Llamas-Velasco, M, Pérez-Gónzalez, YC, Requena, L, and Kutzner, H. Histopathologic clues for the diagnosis of Wiesner nevus. J Am Acad Dermatol . (2014) 70:549–54. doi: 10.1016/j.jaad.2013.10.032
66. Motaparthi, K, Kim, J, Andea, AA, Missall, TA, Novoa, RA, Vidal, CI, et al. TERT and TERT promoter in melanocytic neoplasms: current concepts in pathogenesis, diagnosis, and prognosis. J Cutan Pathol . (2020) 47:710–9. doi: 10.1111/cup.13691
67. Leachman, SA, Carucci, J, Kohlmann, W, Banks, KC, Asgari, MM, Bergman, W, et al. Selection criteria for genetic assessment of patients with familial melanoma. J Am Acad Dermatol . (2009) 61:677.e1–677.e14. doi: 10.1016/j.jaad.2009.03.016
68. Gumaste, PV, Penn, LA, Cymerman, RM, Kirchhoff, T, Polsky, D, and McLellan, B. Skin cancer risk in BRCA1/2 mutation carriers. Br J Dermatol . (2015) 172:1498–506. doi: 10.1111/bjd.13626
69. McGovern, VJ, Mihm, MC, Bailly, C, Booth, JC, Clark, WH Jr, Cochran, AJ, et al. The classification of malignant melanoma and its histologic reporting. Cancer . (1973) 32:1446–57. doi: 10.1002/1097-0142(197312)32:63.0.co;2-8
70. Human malignant melanoma/edited by Wallace H. Clark, Jr., Leonard I. Goldman, Michael J. Mastrangelo . New York: Grune & Stratton (1979).
71. Farmer, ER, Gonin, R, and Hanna, MP. Discordance in the histopathologic diagnosis of melanoma and melanocytic nevi between expert pathologists. Hum Pathol . (1996) 27:528–31. doi: 10.1016/s0046-8177(96)90157-4
72. Corona, R, Mele, A, Amini, M, de Rosa, G, Coppola, G, Piccardi, P, et al. Interobserver variability on the histopathologic diagnosis of cutaneous melanoma and other pigmented skin lesions. J Clin Oncol . (1996) 14:1218–23. doi: 10.1200/JCO.1996.14.4.1218
73. Shoo, BA, Sagebiel, RW, and Kashani-Sabet, M. Discordance in the histopathologic diagnosis of melanoma at a melanoma referral center. J Am Acad Dermatol . (2010) 62:751–6. doi: 10.1016/j.jaad.2009.09.043
74. Elmore, JG, Barnhill, RL, Elder, DE, Longton, GM, Pepe, MS, Reisch, LM, et al. Pathologists’ diagnosis of invasive melanoma and melanocytic proliferations: observer accuracy and reproducibility study. BMJ . (2017) 357:j2813. doi: 10.1136/bmj.j2813
75. Weyers, W, Euler, M, Diaz-Cascajo, C, Schill, WB, and Bonczkowitz, M. Classification of cutaneous malignant melanoma: a reassessment of histopathologic criteria for the distinction of different types. Cancer . (1999) 86:288–99. doi: 10.1002/(sici)1097-0142(19990715)86:23.0.co;2-s
76. Hurt, MA. Types of melanoma? J Am Acad Dermatol . (2008) 58:1059–60. doi: 10.1016/j.jaad.2007.12.009
77. Elder, DE, Bastian, BC, Cree, IA, Massi, D, and Scolyer, RA. The 2018 World Health Organization classification of cutaneous, mucosal, and uveal melanoma: detailed analysis of 9 distinct subtypes defined by their evolutionary pathway. Arch Pathol Lab Med . (2020) 144:500–22. doi: 10.5858/arpa.2019-0561-RA
78. Piepkorn, MW, Barnhill, RL, Elder, DE, Knezevich, SR, Carney, PA, Reisch, LM, et al. The MPATH-dx reporting schema for melanocytic proliferations and melanoma. J Am Acad Dermatol . (2014) 70:131–41. doi: 10.1016/j.jaad.2013.07.027
79. Barnhill, RL, Elder, DE, Piepkorn, MW, Knezevich, SR, Reisch, LM, Eguchi, MM, et al. Revision of the melanocytic pathology assessment tool and hierarchy for diagnosis classification Schema for melanocytic lesions: a consensus statement. JAMA Netw Open . (2023) 6:e2250613. doi: 10.1001/jamanetworkopen.2022.50613
80. Lindelöf, B, and Hedblad, MA. Accuracy in the clinical diagnosis and pattern of malignant melanoma at a dermatological clinic. J Dermatol . (1994) 21:461–4. doi: 10.1111/j.1346-8138.1994.tb01775.x
81. Grin, CM, Kopf, AW, Welkovich, B, Bart, RS, and Levenstein, MJ. Accuracy in the clinical diagnosis of malignant melanoma. Arch Dermatol . (1990) 126:763–6. doi: 10.1001/archderm.1990.01670300063008
82. Morton, CA, and Mackie, RM. Clinical accuracy of the diagnosis of cutaneous malignant melanoma. Br J Dermatol . (1998) 138:283–7. doi: 10.1046/j.1365-2133.1998.02075.x
83. Braun, RP, Rabinovitz, HS, Oliviero, M, Kopf, AW, and Saurat, J. Dermoscopy of pigmented skin lesions. J Am Acad Dermatol . (2005) 52:109–21. doi: 10.1016/j.jaad.2001.11.001
84. Argenziano, G, Soyer, HP, Chimenti, S, Talamini, R, Corona, R, Sera, F, et al. Dermoscopy of pigmented skin lesions: results of a consensus meeting via the internet. J Am Acad Dermatol . (2003) 48:679–93. doi: 10.1067/mjd.2003.281
85. Mayer, J. Systematic review of the diagnostic accuracy of dermatoscopy in detecting malignant melanoma. Med J Aust . (1997) 167:206–10. doi: 10.5694/j.1326-5377.1997.tb138847.x
86. Bafounta, ML, Beauchet, A, Aegerter, P, and Saiag, P. Is dermoscopy (epiluminescence microscopy) useful for the diagnosis of melanoma? Results of a meta-analysis using techniques adapted to the evaluation of diagnostic tests. Arch Dermatol . (2001) 137:1343–50. doi: 10.1001/archderm.137.10.1343
87. Vestergaard, ME, Macaskill, P, Holt, PE, and Menzies, SW. Dermoscopy compared with naked eye examination for the diagnosis of primary melanoma: a meta-analysis of studies performed in a clinical setting. Br J Dermatol . (2008) 159:669–76. doi: 10.1111/j.1365-2133.2008.08713.x
88. Dinnes, J, Deeks, JJ, Chuchu, N, Ferrante di Ruffano, L, Matin, RN, Thomson, DR, et al. Dermoscopy, with and without visual inspection, for diagnosing melanoma in adults. Cochrane Database Syst Rev . (2018) 2018:CD011902. doi: 10.1002/14651858.CD011902.pub2
89. Carli, P. Dermoscopy not yet shown to increase sensitivity of melanoma diagnosis in real practice. Arch Dermatol . (2007) 143:659–66. doi: 10.1001/archderm.143.5.664-b
90. Ferris, LK, Gerami, P, Skelsey, MK, Peck, G, Hren, C, Gorman, C, et al. Real-world performance and utility of a noninvasive gene expression assay to evaluate melanoma risk in pigmented lesions. Melanoma Res . (2018) 28:478–82. doi: 10.1097/CMR.0000000000000478
91. Gerami, P, Yao, Z, Polsky, D, Jansen, B, Busam, K, Ho, J, et al. Development and validation of a noninvasive 2-gene molecular assay for cutaneous melanoma. J Am Acad Dermatol . (2017) 76:114–120.e2. doi: 10.1016/j.jaad.2016.07.038
92. Ferris, LK, Jansen, B, Ho, J, Busam, KJ, Gross, K, Hansen, DD, et al. Utility of a noninvasive 2-gene molecular assay for cutaneous melanoma and effect on the decision to biopsy. JAMA Dermatol . (2017) 153:675–80. doi: 10.1001/jamadermatol.2017.0473
93. Phillips, M, Marsden, H, Jaffe, W, Matin, RN, Wali, GN, Greenhalgh, J, et al. Assessment of accuracy of an artificial intelligence algorithm to detect melanoma in images of skin lesions. JAMA Netw Open . (2019) 2:e1913436. doi: 10.1001/jamanetworkopen.2019.13436
94. Rajpara, SM, Botello, AP, Townend, J, and Ormerod, AD. Systematic review of dermoscopy and digital dermoscopy/ artificial intelligence for the diagnosis of melanoma. Br J Dermatol . (2009) 161:591–604. doi: 10.1111/j.1365-2133.2009.09093.x
95. Sun, MD, Kentley, J, Mehta, P, Dusza, S, Halpern, AC, and Rotemberg, V. Accuracy of commercially available smartphone applications for the detection of melanoma. Br J Dermatol . (2022) 186:744–6. doi: 10.1111/bjd.20903
96. Algorithm based smartphone apps to assess risk of skin cancer in adults: systematic review of diagnostic accuracy studies. BMJ . (2020) 368:m645. doi: 10.1136/bmj.m645
97. Chuchu, N, Takwoingi, Y, Dinnes, J, Matin, RN, Bassett, O, Moreau, JF, et al. Smartphone applications for triaging adults with skin lesions that are suspicious for melanoma. Cochrane Database Syst Rev . (2018) 12:CD013192. doi: 10.1002/14651858.CD013192
98. Liu, Y, Jain, A, Eng, C, Way, DH, Lee, K, Bui, P, et al. A deep learning system for differential diagnosis of skin diseases. Nat Med . (2020) 26:900–8. doi: 10.1038/s41591-020-0842-3
99. Dave, P, Nambudiri, V, and Grant-Kels, JM. The introduction of “Dr AI”: what dermatologists should consider. J Am Acad Dermatol . (2023) 88:1401–2. doi: 10.1016/j.jaad.2022.01.014
100. O’Connor, MK, Dai, H, and Fraga, GR. PRAME immunohistochemistry for melanoma diagnosis: a STARD-compliant diagnostic accuracy study. J Cutan Pathol . (2022) 49:780–6. doi: 10.1111/cup.14267
101. Raghavan, SS, Wang, JY, Kwok, S, Rieger, KE, Novoa, RA, and Brown, RA. PRAME expression in melanocytic proliferations with intermediate histopathologic or spitzoid features. J Cutan Pathol . (2020) 47:1123–31. doi: 10.1111/cup.13818
102. Olds, H, Utz, S, Abrams, J, Terrano, D, and Mehregan, D. Use of PRAME immunostaining to distinguish early melanoma in situ from benign pigmented conditions. J Cutan Pathol . (2022) 49:510–4. doi: 10.1111/cup.14212
103. Alomari, AK, Tharp, AW, Umphress, B, and Kowal, RP. The utility of PRAME immunohistochemistry in the evaluation of challenging melanocytic tumors. J Cutan Pathol . (2021) 48:1115–23. doi: 10.1111/cup.14000
104. Lezcano, C, Jungbluth, AA, Nehal, KS, Hollmann, TJ, and Busam, KJ. PRAME expression in melanocytic tumors. Am J Surg Pathol . (2018) 42:1456–65. doi: 10.1097/PAS.0000000000001134
105. Plotzke, JM, Zoumberos, NA, Hrycaj, SM, Harms, PW, Bresler, SC, and Chan, MP. PRAME expression is similar in scar and desmoplastic melanoma. J Cutan Pathol . (2022) 49:829–32. doi: 10.1111/cup.14286
106. Pavey, SJ, Cummings, MC, Whiteman, DC, Castellano, M, Walsh, MD, Gabrielli, BG, et al. Loss of p16 expression is associated with histological features of melanoma invasion. Melanoma Res . (2002) 12:539–47. doi: 10.1097/00008390-200212000-00003
107. Mason, A, Wititsuwannakul, J, Klump, VR, Lott, J, and Lazova, R. Expression of p16 alone does not differentiate between Spitz nevi and Spitzoid melanoma. J Cutan Pathol . (2012) 39:1062–74. doi: 10.1111/cup.12014
108. Bauer, J, and Bastian, BC. Distinguishing melanocytic nevi from melanoma by DNA copy number changes: comparative genomic hybridization as a research and diagnostic tool. Dermatol Ther . (2006) 19:40–9. doi: 10.1111/j.1529-8019.2005.00055.x
109. Figl, A, Scherer, D, Nagore, E, Bermejo, JL, Botella-Estrada, R, Gast, A, et al. Single-nucleotide polymorphisms in DNA-repair genes and cutaneous melanoma. Mutat Res . (2010) 702:8–16. doi: 10.1016/j.mrgentox.2010.06.011
110. Miedema, J, and Andea, AA. Through the looking glass and what you find there: making sense of comparative genomic hybridization and fluorescence in situ hybridization for melanoma diagnosis. Mod Pathol . (2020) 33:1318–30. doi: 10.1038/s41379-020-0490-7
111. Gerami, P, Mafee, M, Lurtsbarapa, T, Guitart, J, Haghighat, Z, and Newman, M. Sensitivity of fluorescence in situ hybridization for melanoma diagnosis using RREB1, MYB, Cep6, and 11q13 probes in melanoma subtypes. Arch Dermatol . (2010) 146:273–8. doi: 10.1001/archdermatol.2009.386
112. Gerami, P, Li, G, Pouryazdanparast, P, Blondin, B, Beilfuss, B, Slenk, C, et al. A highly specific and discriminatory FISH assay for distinguishing between benign and malignant melanocytic neoplasms. Am J Surg Pathol . (2012) 36:808–17. doi: 10.1097/PAS.0b013e31824b1efd
113. Ko, JS, Matharoo-Ball, B, Billings, SD, Thomson, BJ, Tang, JY, Sarin, KY, et al. Diagnostic distinction of malignant melanoma and benign nevi by a gene expression signature and correlation to clinical outcomes. Cancer Epidemiol Biomark Prev . (2017) 26:1107–13. doi: 10.1158/1055-9965.EPI-16-0958
114. Clarke, LE, Flake, DD, Busam, K, Cockerell, C, Helm, K, McNiff, J, et al. An independent validation of a gene expression signature to differentiate malignant melanoma from benign melanocytic nevi. Cancer . (2017) 123:617–28. doi: 10.1002/cncr.30385
115. Clarke, LE, Warf, MB, Flake, DD, Hartman, A‐R, Tahan, S, Shea, CR, et al. Clinical validation of a gene expression signature that differentiates benign nevi from malignant melanoma. J Cutan Pathol . (2015) 42:244–52. doi: 10.1111/cup.12475
116. Reimann, JDR, Salim, S, Velazquez, EF, Wang, L, Williams, KM, Flejter, WL, et al. Comparison of melanoma gene expression score with histopathology, fluorescence in situ hybridization, and SNP array for the classification of melanocytic neoplasms. Mod Pathol . (2018) 31:1733–43. doi: 10.1038/s41379-018-0087-6
117. Minca, EC, Al-Rohil, RN, Wang, M, Harms, PW, Ko, JS, Collie, AM, et al. Comparison between melanoma gene expression score and fluorescence in situ hybridization for the classification of melanocytic lesions. Mod Pathol . (2016) 29:832–43. doi: 10.1038/modpathol.2016.84
118. Hart, SN, Flotte, W, Norgan, AP, Shah, KK, Buchan, ZR, Mounajjed, T, et al. Classification of melanocytic lesions in selected and whole-slide images via convolutional neural networks. J Pathol Inform . (2019) 10:5. doi: 10.4103/jpi.jpi_32_18
119. Hekler, A, Utikal, JS, Enk, AH, Berking, C, Klode, J, Schadendorf, D, et al. Pathologist-level classification of histopathological melanoma images with deep neural networks. Eur J Cancer . (2019) 115:79–83. doi: 10.1016/j.ejca.2019.04.021
120. Hekler, A, Utikal, JS, Enk, AH, Solass, W, Schmitt, M, Klode, J, et al. Deep learning outperformed 11 pathologists in the classification of histopathological melanoma images. Eur J Cancer . (2019) 118:91–6. doi: 10.1016/j.ejca.2019.06.012
121. Stiff, KM, Franklin, MJ, Zhou, Y, Madabhushi, A, and Knackstedt, TJ. Artificial intelligence and melanoma: a comprehensive review of clinical, dermoscopic, and histologic applications. Pigment Cell Melanoma Res . (2022) 35:203–11. doi: 10.1111/pcmr.13027
122. Brinker, TJ, Schmitt, M, Krieghoff-Henning, EI, Barnhill, R, Beltraminelli, H, Braun, SA, et al. Diagnostic performance of artificial intelligence for histologic melanoma recognition compared to 18 international expert pathologists. J Am Acad Dermatol . (2022) 86:640–2. doi: 10.1016/j.jaad.2021.02.009
123. Ianni, JD, Soans, RE, Sankarapandian, S, Chamarthi, RV, Ayyagari, D, Olsen, TG, et al. Tailored for real-world: a whole slide image classification system validated on Uncurated multi-site data emulating the prospective pathology workload. Sci Rep . (2020) 10:3217. doi: 10.1038/s41598-020-59985-2
124. Wells, A, Patel, S, Lee, JB, and Motaparthi, K. Artificial intelligence in dermatopathology: diagnosis, education, and research. J Cutan Pathol . (2021) 48:1061–8. doi: 10.1111/cup.13954
125. Keung, EZ, and Gershenwald, JE. The eighth edition American joint committee on Cancer (AJCC) melanoma staging system: implications for melanoma treatment and care. Expert Rev Anticancer Ther . (2018) 18:775–84. doi: 10.1080/14737140.2018.1489246
126. Weitemeyer, MB, Helvind, NM, Brinck, AM, Hölmich, LR, and Chakera, AH. More sentinel lymph node biopsies for thin melanomas after transition to AJCC 8th edition do not increase positivity rate: a Danish population-based study of 7148 patients. J Surg Oncol . (2022) 125:498–508. doi: 10.1002/jso.26723
127. Marchetti, MA, Coit, DG, Dusza, SW, Yu, A, McLean, LT, Hu, Y, et al. Performance of gene expression profile tests for prognosis in patients with localized cutaneous melanoma: a systematic review and Meta-analysis. JAMA Dermatol . (2020) 156:953–62. doi: 10.1001/jamadermatol.2020.1731
128. Grossman, D, Okwundu, N, Bartlett, EK, Marchetti, MA, Othus, M, Coit, DG, et al. Prognostic gene expression profiling in cutaneous melanoma: identifying the knowledge gaps and assessing the clinical benefit. JAMA Dermatol . (2020) 156:1004–11. doi: 10.1001/jamadermatol.2020.1729
129. Hsueh, EC, DeBloom, JR, Lee, J, Sussman, JJ, Covington, KR, Middlebrook, B, et al. Interim analysis of survival in a prospective, multi-center registry cohort of cutaneous melanoma tested with a prognostic 31-gene expression profile test. J Hematol Oncol . (2017) 10:152. doi: 10.1186/s13045-017-0520-1
130. Keller, J, Schwartz, TL, Lizalek, JM, Chang, E-S, Patel, AD, Hurley, MY, et al. Prospective validation of the prognostic 31-gene expression profiling test in primary cutaneous melanoma. Cancer Med . (2019) 8:2205–12. doi: 10.1002/cam4.2128
131. Greenhaw, BN, Covington, KR, Kurley, SJ, Yeniay, Y, Cao, NA, Plasseraud, KM, et al. Molecular risk prediction in cutaneous melanoma: a meta-analysis of the 31-gene expression profile prognostic test in 1,479 patients. J Am Acad Dermatol . (2020) 83:745–53. doi: 10.1016/j.jaad.2020.03.053
132. Kovarik, CL, Chu, EY, and Adamson, AS. Gene expression profile testing for thin melanoma: evidence to support clinical use remains thin. JAMA Dermatol . (2020) 156:837–8. doi: 10.1001/jamadermatol.2020.0894
133. Marchetti, MA, Bartlett, EK, Dusza, SW, and Bichakjian, CK. Use of a prognostic gene expression profile test for T1 cutaneous melanoma: will it help or harm patients? J Am Acad Dermatol . (2019) 80:e161–2. doi: 10.1016/j.jaad.2018.11.063
134. Kangas-Dick, AW, Greenbaum, A, Gall, V, Groisberg, R, Mehnert, J, Chen, C, et al. Evaluation of a gene expression profiling assay in primary cutaneous melanoma. Ann Surg Oncol . (2021) 28:4582–9. doi: 10.1245/s10434-020-09563-7
135. Farberg, AS, Marson, JW, Glazer, A, Litchman, GH, Svoboda, R, Winkelmann, RR, et al. Expert consensus on the use of prognostic gene expression profiling tests for the Management of Cutaneous Melanoma: consensus from the skin Cancer prevention working group. Dermatol Ther (Heidelb) . (2022) 12:807–23. doi: 10.1007/s13555-022-00709-x
136. Sabel, MS. Genomic expression profiling in melanoma and the road to clinical practice. Ann Surg Oncol . (2022) 29:764–6. doi: 10.1245/s10434-021-110993
Keywords: melanoma, melanoma epidemiology, melanoma genomics, melanoma diagnosis, melanoma classification
Citation: Waseh S and Lee JB (2023) Advances in melanoma: epidemiology, diagnosis, and prognosis. Front. Med . 10:1268479. doi: 10.3389/fmed.2023.1268479
Received: 28 July 2023; Accepted: 13 October 2023; Published: 22 November 2023.
Reviewed by:
Copyright © 2023 Waseh and Lee. This is an open-access article distributed under the terms of the Creative Commons Attribution License (CC BY) . The use, distribution or reproduction in other forums is permitted, provided the original author(s) and the copyright owner(s) are credited and that the original publication in this journal is cited, in accordance with accepted academic practice. No use, distribution or reproduction is permitted which does not comply with these terms.
*Correspondence: Jason B. Lee, [email protected]
This article is part of the Research Topic
Cutaneous Oncology and Skin Cancer Genomics
mRNA vaccine for melanoma reduces relapse after therapy, trial finds
In 2019, Gary Keblish got a diagnosis from his surgeon that left him stunned. The flat, dark-brown mole he’d had on his back for as long as he could remember had turned into a melanoma that was already advanced, putting him at risk of dying.
“I felt numb,” the Brooklyn teacher, 61, said in an interview.
Fortunately, Keblish was able sign on for a small clinical trial testing a preventive vaccine that might possibly keep the disease from coming back.
The trial focused on a personalized vaccine using mRNA technology that used mutations to target mutations unique to a patient’s cancer but not the healthy cells in the body. All participants in the trial would receive the immunotherapy drug Keytruda (pembrolizumab), the standard of care for high-risk melanoma patients like Keblish. Two-thirds of the participants would also receive the vaccine.
Keblish was one of those who received the vaccine — which teaches the body’s immune system to recognize cancer cells as different from normal cells so that, working along with the immunotheraoy drug, it can attack them.
After two years, Keblish's cancer hasn't returned.
Personalized cancer vaccine shows benefit
On Sunday, the results of the phase 2 trial, which showed that the combination of the vaccine and immunotherapy reduced the risk of recurrence by nearly half, were presented at the annual meeting of the American Association for Cancer Research.
It's the first randomized, controlled trial to show a benefit from this type of cancer vaccine, said senior investigator Dr. Jeffrey Weber, a deputy director of NYU Langone’s Perlmutter Cancer Center and a professor of medicine at the NYU Grossman School of Medicine.
To test the effectiveness of the vaccine, the international team of researchers recruited 157 melanoma patients whose tumors had been surgically removed and who were at high risk of experiencing a recurrence of their cancers. Fifty patients received only the immunotherapy medication and 107 also got the personalized vaccination.
One of the ways cancer evades the immune system is to fool the body into thinking the threat is over, at which point the natural braking system that prevents the immune system from staying constantly on kicks in. Weber compares the way pembrolizumab works to cutting a stuck brake cable on a car so it can go forward.
Once the system’s braking system has been partially disabled, “the immune system works really well,” Weber said, adding that the downside of “cutting brake cable” is that the immune system stays turned up and some people end up with inflammation and something that resembles an autoimmune disease.
One other way cancer can avoid being destroyed is through mutations, so the immune system’s soldiers cease to recognize it as a threat.
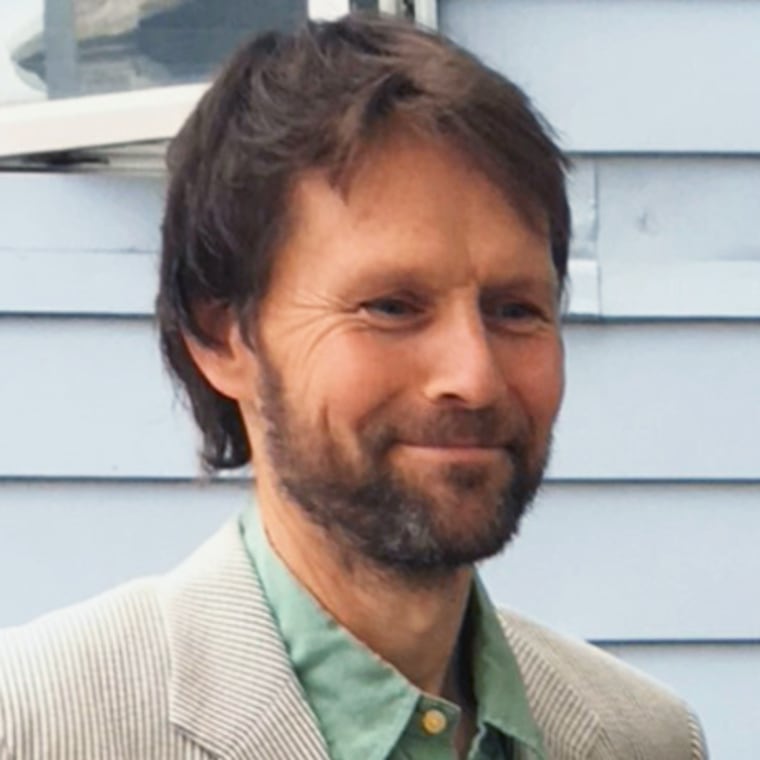
That’s where the personalized mRNA vaccine comes in. After a patient’s tumor is removed, doctors identify proteins that are specific to that person’s tumor and no other cells in the body. As many as 34 proteins from a patient’s tumor were then the target of the vaccine.
In the trial, 40% of the patients who received only the immunotherapy drug had a recurrence of their cancer during the two-year follow-up. In comparison, 22.4% of patients who got the drug plus the vaccine had a recurrence, leading to a difference of 44% between the two groups.
The new findings are significant, said Dr. Antoni Ribas, a professor of medicine at the University of California, Los Angeles, and director of Tumor Immunology Program at UCLA’s Jonsson Comprehensive Cancer Center.
It’s the first time a cancer vaccine has been shown to have this level of benefit, close to a 50% decrease in the risk of relapse,” said Ribas. “It tells us these vaccines actually work and can turn on an immune response against the patient’s own cancer."
The trial’s results are “very exciting,” said Dr. Thomas Marron, director of the Early Phase Trials Unit at the Tisch Cancer Institute and an associate professor of medicine at the Icahn School of Medicine at Mount Sinai in New York.
“Once the tumor is removed, we know it can come back because tiny microscopic bits have traveled elsewhere in the body and set up shop there,” Marron said. The recurrence often appears quickly, between six months and two years, he said.
The beauty of the vaccine in this study is that it targets up to 34 mutations, Marron said. “That’s like taking 34 shots on goal," he said. You’re teaching the immune system to recognize 34 different things that are unique to that cancer.”
The researchers anticipate the phase 3 trial, scheduled to launch this summer, to show similar results. With follow-up and monitoring, it could be at least two years before data is registered with the Food and Drug Administration and up to three years before the vaccine combination would be approved for use in patients, Weber said.
Still, it's an exciting advance in the field of cancer vaccines, particularly for stopping melanoma, the deadliest form of skin cancer, experts said.
The study is “important because it’s the first randomized study of a cancer vaccine with a clinically meaningful endpoint: stopping tumors from coming back,” said Dr. Margaret Callahan, research director of the Memorial Sloan Kettering Immunotherapeutics Program, who expressed cautious optimism about the findings.
“This is an exciting advance in the field of cancer vaccines, an area notoriously tough to make progress in,” Callahan said.
Linda Carroll is a regular health contributor to NBC News. She is coauthor of "The Concussion Crisis: Anatomy of a Silent Epidemic" and "Out of the Clouds: The Unlikely Horseman and the Unwanted Colt Who Conquered the Sport of Kings."
Reynolds Lewis is a producer with the NBC News Health & Medical Unit.
Immunotherapy in Melanoma: Recent Advances and Future Directions
Affiliations.
- 1 Department of Medicine, Division of General Internal Medicine, University of Pittsburgh Medical Center, Pittsburgh, PA 15213, USA.
- 2 Department of Cutaneous Oncology, H. Lee Moffitt Cancer Center and Research Institute, Tampa, FL 33612, USA.
- 3 Department of Medicine, Division of Hematology/Oncology, University of Pittsburgh Medical Center, Hillman Cancer Center, Pittsburgh, PA 15213, USA.
- 4 Tumor Microenvironment Center, UPMC Hillman Cancer Center, Pittsburgh, PA 15232, USA.
- PMID: 36831449
- PMCID: PMC9954703
- DOI: 10.3390/cancers15041106
The use of immunotherapy in the treatment of advanced and high-risk melanoma has led to a striking improvement in outcomes. Although the incidence of melanoma has continued to rise, median survival has improved from approximately 6 months to nearly 6 years for patients with advanced inoperable stage IV disease. Recent understanding of the tumor microenvironment and its interplay with the immune system has led to the explosive development of novel immunotherapy treatments. Since the approval of the therapeutic cytokines interleukin-2 and interferon alfa-2 in the 1990s, the development of novel immune checkpoint inhibitors (ICIs), oncolytic virus therapy, and modulators of the tumor microenvironment have given way to a new era in melanoma treatment. Monoclonal antibodies directed at programmed cell death protein 1 receptor (PD-1) and its ligand (PDL-1), cytotoxic T-lymphocyte-associated protein 4 (CTLA-4), and lymphocyte-activation gene 3 (LAG-3) have provided robust activation of the adaptive immune system, restoring immune surveillance leading to host tumor recognition and destruction. Multiple other immunomodulatory therapeutics are under investigation to overcome resistance to ICI therapy, including the toll-like receptor-9 (TLR-9) and 7/8 (TLR-7/8) agonists, stimulator of interferon genes (STING) agonists, and fecal microbiota transplantation. In this review, we focus on the recent advances in immunotherapy for the treatment of melanoma and provide an update on novel therapies currently under investigation.
Keywords: LAG-3; STING; T-VEC; TLR-9; adoptive cell therapy; fecal microbiota transplant; immune checkpoint blockade; immunotherapy; melanoma.
Publication types
Grants and funding.
- See us on facebook
- See us on twitter
- See us on youtube
- See us on linkedin
- See us on instagram
AI improves accuracy of skin cancer diagnoses in Stanford Medicine-led study
Artificial intelligence algorithms powered by deep learning improve skin cancer diagnostic accuracy for doctors, nurse practitioners and medical students in a study led by the Stanford Center for Digital Health.
April 11, 2024 - By Krista Conger
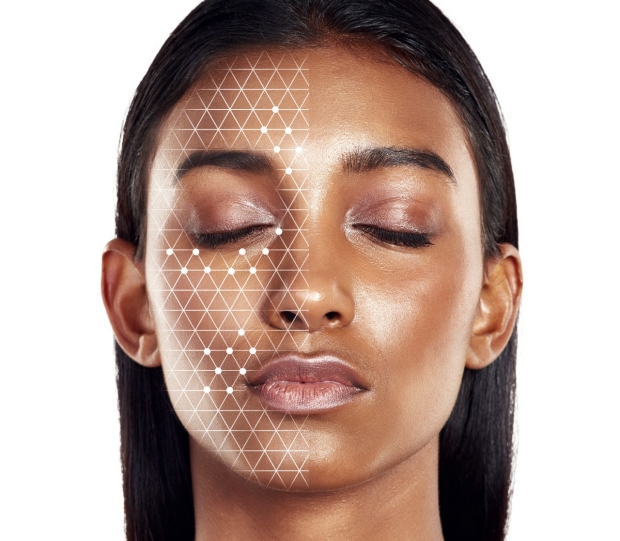
Artificial intelligence helped clinicians diagnose skin cancer more accurately, a Stanford Medicine-led study found. Chanelle Malambo/peopleimages.com - stock.adobe.com
A new study led by researchers at Stanford Medicine finds that computer algorithms powered by artificial intelligence based on deep learning can help health care practitioners to diagnose skin cancers more accurately. Even dermatologists benefit from AI guidance, although their improvement is less than that seen for non-dermatologists.
“This is a clear demonstration of how AI can be used in collaboration with a physician to improve patient care,” said professor of dermatology and of epidemiology Eleni Linos , MD. Linos leads the Stanford Center for Digital Health , which was launched to tackle some of the most pressing research questions at the intersection of technology and health by promoting collaboration between engineering, computer science, medicine and the humanities.
Linos, associate dean of research and the Ben Davenport and Lucy Zhang Professor in Medicine, is the senior author of the study , which was published on April 9 in npj Digital Medicine . Postdoctoral scholar Jiyeong Kim , PhD, and visiting researcher Isabelle Krakowski, MD, are the lead authors of the research.
“Previous studies have focused on how AI performs when compared with physicians,” Kim said. “Our study compared physicians working without AI assistance with physicians using AI when diagnosing skin cancers.”
AI algorithms are increasingly used in clinical settings, including dermatology. They are created by feeding a computer hundreds of thousands or even millions of images of skin conditions labeled with information such as diagnosis and patient outcome. Through a process called deep learning, the computer eventually learns to recognize telltale patterns in the images that correlate with specific skin diseases including cancers. Once trained, an algorithm written by the computer can be used to suggest possible diagnoses based on an image of a patient’s skin that it has not been exposed to.
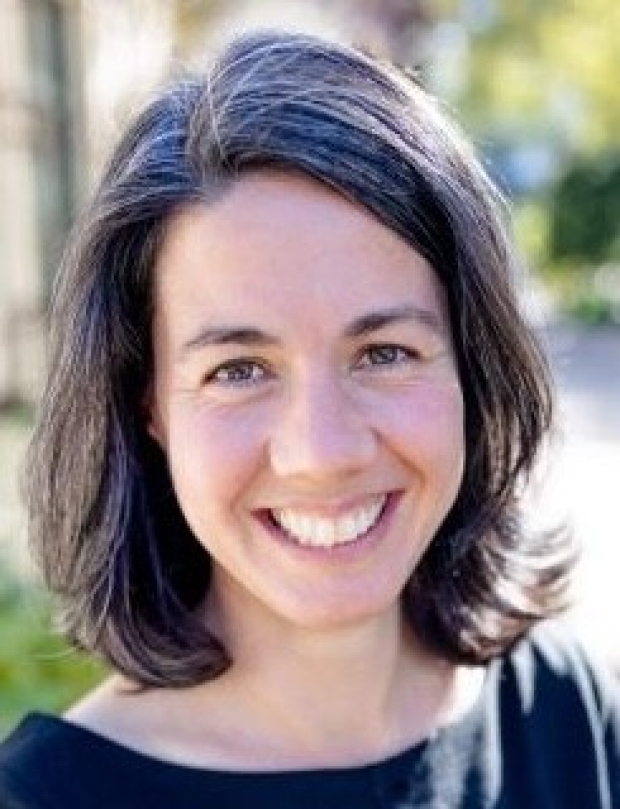
Eleni Linos
These diagnostic algorithms aren’t used alone, however. They are overseen by clinicians who also assess the patient, come to their own conclusions about a patient’s diagnosis and choose whether to accept the algorithm’s suggestion.
An accuracy boost
Kim and Linos’ team reviewed 12 studies detailing more than 67,000 evaluations of potential skin cancers by a variety of practitioners with and without AI assistance. They found that, overall, health care practitioners working without aid from artificial intelligence were able to accurately diagnose about 75% of people with skin cancer — a statistical measurement known as sensitivity. Conversely, the workers correctly diagnosed about 81.5% of people with cancer-like skin conditions but who did not have cancer — a companion measurement known as specificity.
Health care practitiones who used AI to guide their diagnoses did better. Their diagnoses were about 81.1% sensitive and 86.1% specific. The improvement may seem small, but the differences are critical for people told they don’t have cancer, but do, or for those who do have cancer but are told they are healthy.
When the researchers split the health care practitioners by specialty or level of training, they saw that medical students, nurse practitioners and primary care doctors benefited the most from AI guidance — improving on average about 13 points in sensitivity and 11 points in specificity. Dermatologists and dermatology residents performed better overall, but the sensitivity and specificity of their diagnoses also improved with AI.
“I was surprised to see everyone’s accuracy improve with AI assistance, regardless of their level of training,” Linos said. “This makes me very optimistic about the use of AI in clinical care. Soon our patients will not just be accepting, but expecting, that we use AI assistance to provide them with the best possible care.”
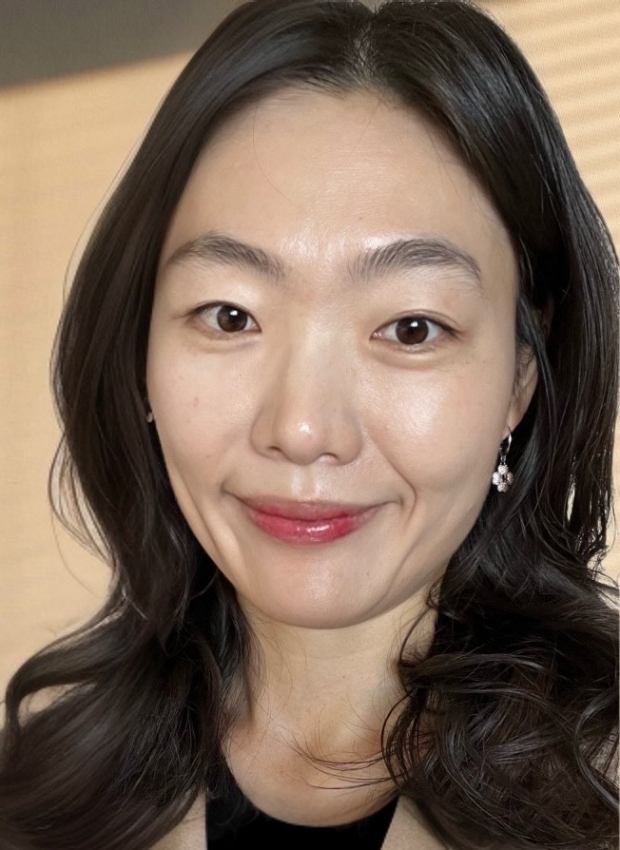
Jiyeong Kim
Researchers at the Stanford Center for Digital Health, including Kim, are interested in learning more about the promise of and barriers to integrating AI-based tools into health care. In particular, they are planning to investigate how the perceptions and attitudes of physicians and patients to AI will influence its implementation.
“We want to better understand how humans interact with and use AI to make clinical decisions,” Kim said.
Previous studies have indicated that a clinician’s degree of confidence in their own clinical decision, the degree of confidence of the AI, and whether the clinician and the AI agree on the diagnosis all influence whether the clinician incorporates the algorithm’s advice when making clinical decisions for a patient.
Medical specialties like dermatology and radiology, which rely heavily on images — visual inspection, pictures, X-rays, MRIs and CT scans, among others — for diagnoses are low-hanging fruit for computers that can pick out levels of detail beyond what a human eye (or brain) can reasonably process. But even other more symptom-based specialties, or prediction modeling, are likely to benefit from AI intervention, Linos and Kim feel. And it’s not just patients who stand to benefit.
“If this technology can simultaneously improve a doctor’s diagnostic accuracy and save them time, it’s really a win-win. In addition to helping patients, it could help reduce physician burnout and improve the human interpersonal relationships between doctors and their patients,” Linos said. “I have no doubt that AI assistance will eventually be used in all medical specialties. The key question is how we make sure it is used in a way that helps all patients regardless of their background and simultaneously supports physician well-being.”
Researchers from the Karolinska Institute, the Karolinska University Hospital and the University of Nicosia contributed to the research.
The study was funded by the National Institutes of Health (grants K24AR075060 and R01AR082109), Radiumhemmet Research, the Swedish Cancer Society and the Swedish Research Council.
For more news about responsible AI in health and medicine, sign up for the RAISE Health newsletter.
Register for the RAISE Health Symposium on May 14.
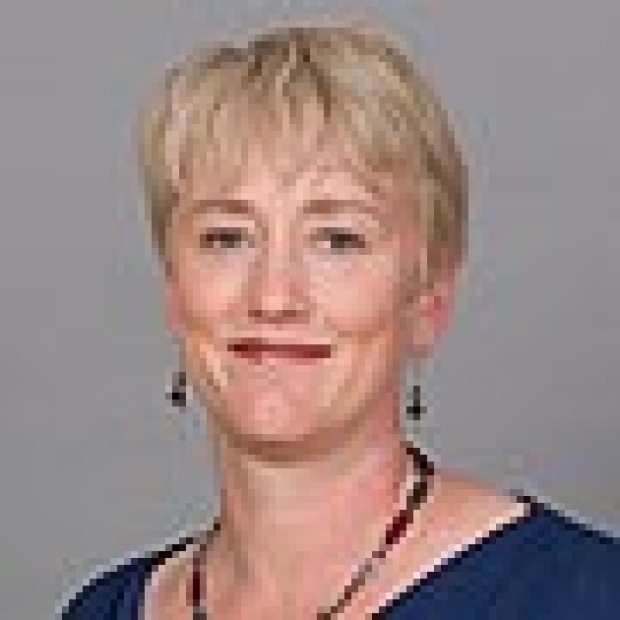
About Stanford Medicine
Stanford Medicine is an integrated academic health system comprising the Stanford School of Medicine and adult and pediatric health care delivery systems. Together, they harness the full potential of biomedicine through collaborative research, education and clinical care for patients. For more information, please visit med.stanford.edu .
Artificial intelligence
Exploring ways AI is applied to health care

Advertisement
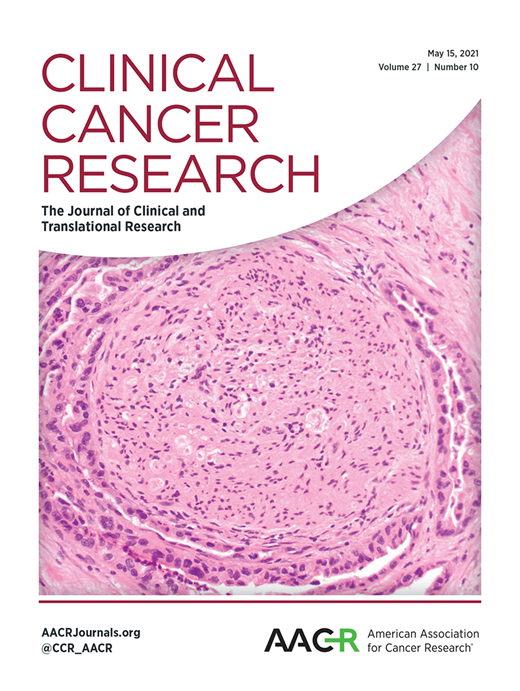
- Previous Article
- Next Article
Introduction
Tumor cell dormancy and metastasis, targeted therapy, immunotherapy, melanoma and covid-19, conclusions/the path forward, authors' disclosures, acknowledgments, the state of melanoma: emergent challenges and opportunities.

Clin Cancer Res 2021;27:2678–97
- Funder(s): NIH
- Award Id(s): 1P50CA221703
- Principal Award Recipient(s): J.E. Gershenwald
- Award Id(s): P50CA225450
- Principal Award Recipient(s): E. Hernando
- Funder(s): DoD
- Award Id(s): CA170374
- Award Id(s): ZIABC008756
- Principal Award Recipient(s): G. Merlino
- Funder(s): InterMEL
- Award Id(s): P01CA206980
- Funder(s): Melanoma Research Foundation
- Split-Screen
- Article contents
- Figures & tables
- Supplementary Data
- Peer Review
- Open the PDF for in another window
- Get Permissions
- Cite Icon Cite
- Search Site
- Version of Record May 15 2021
- Proof April 22 2021
- Accepted Manuscript January 7 2021
Michael B. Atkins , Clara Curiel-Lewandrowski , David E. Fisher , Susan M. Swetter , Hensin Tsao , Julio A. Aguirre-Ghiso , Maria S. Soengas , Ashani T. Weeraratna , Keith T. Flaherty , Meenhard Herlyn , Jeffrey A. Sosman , Hussein A. Tawbi , Anna C. Pavlick , Pamela B. Cassidy , Sunandana Chandra , Paul B. Chapman , Adil Daud , Zeynep Eroglu , Laura K. Ferris , Bernard A. Fox , Jeffrey E. Gershenwald , Geoffrey T. Gibney , Douglas Grossman , Brent A. Hanks , Douglas Hanniford , Eva Hernando , Joanne M. Jeter , Douglas B. Johnson , Samir N. Khleif , John M. Kirkwood , Sancy A. Leachman , Darren Mays , Kelly C. Nelson , Vernon K. Sondak , Ryan J. Sullivan , Glenn Merlino; on behalf of the Melanoma Research Foundation, The State of Melanoma: Emergent Challenges and Opportunities. Clin Cancer Res 15 May 2021; 27 (10): 2678–2697. https://doi.org/10.1158/1078-0432.CCR-20-4092
Download citation file:
- Ris (Zotero)
- Reference Manager
Five years ago, the Melanoma Research Foundation (MRF) conducted an assessment of the challenges and opportunities facing the melanoma research community and patients with melanoma. Since then, remarkable progress has been made on both the basic and clinical research fronts. However, the incidence, recurrence, and death rates for melanoma remain unacceptably high and significant challenges remain. Hence, the MRF Scientific Advisory Council and Breakthrough Consortium, a group that includes clinicians and scientists, reconvened to facilitate intensive discussions on thematic areas essential to melanoma researchers and patients alike, prevention, detection, diagnosis, metastatic dormancy and progression, response and resistance to targeted and immune-based therapy, and the clinical consequences of COVID-19 for patients with melanoma and providers. These extensive discussions helped to crystalize our understanding of the challenges and opportunities facing the broader melanoma community today. In this report, we discuss the progress made since the last MRF assessment, comment on what remains to be overcome, and offer recommendations for the best path forward.
The incidence of melanoma continues to grow worldwide. Interest in melanoma and the care of patients who suffer from it have also grown. The advent of the clinical use of the BRAF V600E inhibitors, vemurafenib and dabrafenib, tremendously boosted the prospects and hopes of patients with melanoma, but it was the use of ipilimumab, nivolumab, and pembrolizumab that made melanoma the poster child for immune checkpoint inhibitor treatment. Much has been accomplished over the last half decade in both the basic and clinical research arenas, and advances in our understanding and treatment of melanoma are now being applied to other forms of cancer. However, incidence, recurrence, and death rates remain unacceptably high, and much remains to be done to address fundamental questions about melanoma prevention, detection, diagnosis, metastatic dormancy and progression, and response and resistance to targeted and immune-based therapy. In this report, leading melanoma investigators consider the challenges that the field must overcome to make progress in all of these key areas. We also discuss issues that clinicians, investigators, and patients with melanoma face with respect to severe acute respiratory syndrome coronavirus 2 (SARS CoV-2) infection. Finally, biomedical research is advancing at a breakneck pace, and we discuss the best ways to exploit new scientific and technical discoveries to facilitate the care and recovery of patients with melanoma.
Primary prevention
Biological addiction in melanoma risk and prevention.
Since ultraviolet (UV) radiation is established as a primary carcinogen for both melanoma and nonmelanoma skin cancers, prevention strategies that seek to minimize UV mutagenesis are of clear significance. These prominently include UV avoidance (shade and protective clothing) and sunscreen usage, coupled with educational initiatives. Increased research on UV signaling responses in skin has shed light on potential mechanisms that may underlie sun-seeking behaviors. The UV-tanning pathway triggers p53-mediated induction of proopiomelanocortin expression, followed by cleavage into multiple bioactive peptides, which include melanocyte-stimulating hormone (MSH), as well as β-endorphin. Production of both MSH and β-endorphin has been shown to produce behavioral changes of addictive-like dependency. Given the “paradoxical” linkage of UV addiction to environmental mutagenesis, these findings suggest the existence of a powerful evolutionary driver, which may well be vitamin D. Studies are investigating potential linkage of vitamin D to opiate-like UV responses. However, it is critical to correct the misconception, sometimes suggested by the tanning industry, that UV is a “healthier” source of vitamin D than supplements.
Research is needed to better understand the role of vitamin D deficiency, as well as various behavioral diagnoses (addictions, affective disorders, etc.) that may be associated with sun-seeking behavior. While some people might become addicted to tanning, there are no established clinical screening or diagnostic criteria for this behavior, and research in this area is ongoing. Another primary prevention strategy that has been suggested, although not proven, involves approaches to alter skin pigmentation from fair-skin (high pheomelanin-to-eumelanin ratio) toward darker skin pigmentation. While safety of such approaches is paramount, the principle of mimicking epidemiologically low-risk human pigmentation phototypes represents a potentially valuable research approach. For example, the ability to potentially darken “amelanotic” melanocyte neoplasms might lead to earlier diagnoses and possible clinical benefit.
Recommended readings, refs. 1, 2 .
State of sunscreen safety and recommendations
The foundations of melanoma prevention include sun avoidance and routine sunscreen use. However, little is known about systemic absorption and toxicity of common sunscreens. In a pilot study, each of four sunscreen agents (avobenzone, oxybenzone, octocrylene, and ecamsule) was applied to volunteers four times per day for 4 days to 75% body surface, and the sunscreen levels in their blood samples were quantified. In each case, blood concentrations of the applied sunscreen exceeded the Food and Drug Administration (FDA) threshold for waiving nonclinical toxicology studies. A follow-up expanded randomized clinical trial of six active ingredients led to essentially the same conclusions. While there is no evidence that the measured levels actually cause toxicity in humans, these studies clearly demonstrate that common commercial sunscreens can be absorbed through the skin. Considering the importance of sunscreens in sun-safety practices and skin cancer prevention, long-term safety studies should be carried out, and will likely require participation from investigators from dermatology, pharmacology, toxicology, public health, and behavioral science. It is also critical that the FDA accelerates investigation and incorporation of additional UV filters that may have improved efficacy and favorable safety profiles.
Concerns about sunscreen toxicity also extend to aquatic life. A study by the State of Florida Office of Program Policy Analysis and Government Accountability found that oxybenzone and octinoxate in chemical sunscreens can have a negative effect on corals and other marine life, albeit following exposure to concentration levels generally not observed in nature. Another study assessed the concentrations of various UV filters and hormones in the surface seawater, sediment, and coral tissue in Hawaii, and found trace concentrations of the UV-B filter, oxybenzone, in all of them. Although the actual biologic significance of findings such as these remains unknown, Hawaii became the first state to ban the use of sunscreens containing oxybenzone and octinoxate, effective January 1, 2021. Significant coral depletion over the last few decades has been linked to other factors, including ocean warming and acidification driven by climate change, overfishing, and pollution. Nevertheless, the use of “environmentally safe” compounds, such mineral-based sunscreens (i.e., zinc oxide or titanium dioxide), and sun-protective clothing can perhaps mitigate against further damaging this threatened ecosystem. Future studies should be aimed at determining the actual biological effects of chemical sunscreens in humans and on our environment.
The FDA regulates sunscreen as an over-the-counter drug; an unintended consequence of this policy is restrictions on the ability of students to carry or use sunscreens on school premises and/or at school activities in many school districts around the country. As of July 2019, 22 states have enacted legislation to allow students to possess and self-apply sunscreen at school, which improves opportunities for routine use ( https://www.cdc.gov/cancer/skin/pdf/SkinCancerPreventionProgressReport-2019-508.pdf ). Sun-protective fabrics in clothing and hats provide an UV protection factor (UPF) that may be more effective, acceptable, and/or serve as an adjunct to sunscreen use. An advantage of the UPF standard is that both UVB and UVA are measured. A UPF of 50 blocks 98% of UVR, and activewear with this rating is readily available. Ongoing efforts to promote sunscreen as part of other sun-protective behaviors, including sun avoidance at peak UV times and use of sun-protective clothing, hats, and eyewear, are needed in public health messaging.
Recommended readings, refs. 3, 4 .
Implementing behavior change to enhance primary and secondary melanoma prevention through legislation and education
Empowering behavioral change for primary and secondary melanoma prevention remains challenging. Tanning via natural sunlight and/or indoor tanning bed use remains prevalent in our society, including among young people. To date in the United States, legislation prohibiting indoor tanning by minors (under 18 years old) has been enacted in 21 states and the District of Columbia, an increase from only two states in 2012. On the basis of Centers for Disease Control (CDC) Youth Risk Behavior Survey data, indoor tanning bed use among high school students has decreased significantly over the past several years, now exceeding reduction targets set forth in the Healthy People 2020 initiative. Indeed, the observed 64% decrease in tanning bed use among high school students between 2009 and 2017 is encouraging, and is likely related to the above-noted statewide restriction of indoor tanning by minors, as well as other initiatives. Use of indoor tanning facilities by adults has also been decreasing. According to National Health Interview Survey data, overall prevalence of indoor tanning dropped from 5.5% in 2010 to 3.5% in 2015; during the same interval, indoor tanning prevalence decreased for non-Hispanic white females ages 18–21 (31.8%–20.4%), 22–25 (29.6%–13.9%), and 26–29 years (22.1%–13.8%).
Internationally, Australia, Brazil, and Iran have restricted access to indoor tanning devices by all individuals; multiple other countries, most recently Spain and Poland, have enacted national laws that restrict use of indoor tanning devices by minors. A FDA regulation was proposed in 2015 that would restrict indoor tanning among all minors nationwide; however, as of Fall 2020, this regulation has not yet been finalized. Overall, changing social norms related to tanning increasingly involves novel approaches to reach the at-risk population, including social media messaging through “influencers” and ongoing legislation to ban tanning beds outright, as has been done in Australia and other countries.
Early detection strategies should ideally target the populations at highest risk for fatal melanoma: namely, middle-aged and older white men, and individuals of lower socioeconomic status (SES, for both sexes and across all racial-ethnic groups). The increasing burden of advanced melanoma in lower SES individuals highlights the need for improved community outreach, education, and awareness campaigns focused on melanoma risk and clinical warning signs, in tandem with novel care approaches (i.e., teledermatology/teledermoscopy) that can improve access to specialists for prompt diagnosis and treatment. Barriers to specialist access that impede early detection of melanoma are highlighted by health disparities in melanoma outcomes in rural areas in the United States and abroad. Limited melanoma-specific knowledge and perceived general health competence are associated with thicker melanoma, highlighting the potential value of broader public health campaigns intended to raise awareness.
Population-based data from the CDC (United States) and Queensland (Australia) demonstrate a persistent increasing incidence of melanoma in older adults, highlighting an ongoing unmet clinical need to enhance prevention efforts in this demographic. Remarkably, these data also demonstrate a decreasing incidence in younger adults, demonstrating the clinical impact of primary prevention efforts. Overall, there is significant opportunity to build on recent successes to continue to coordinate approaches to educate stakeholders on the dangers of UV radiation overexposure in support of legislative initiatives to restrict indoor tanning by minors at the U.S. state, national, and international levels, as well as a strong unmet clinical need to improve early detection strategies for those at highest risk.
Community-based efforts to improve primary and secondary melanoma prevention efforts are ongoing. For example, as part of a community-wide effort led by The University of Texas MD Anderson Cancer Center (Houston, TX) known as BeWellBaytown, the town of Baytown, TX, is being mobilized to promote wellness and stop cancer before it starts ( https://www.mdanderson.org/research/research-areas/prevention-personalized-risk-assessment/be-well-communities/be-well-baytown.html ). As a part of this initiative, evidence-based risk reduction strategies and programs are being employed to reduce UV radiation overexposure through: sun-safe education and a district-wide sun-safety policy, multifaceted education sessions on sun-protective behaviors, training on sun-protective behaviors for city staff who work outdoors, community infrastructure improvements to reduce excessive exposure to UV radiation in preschools, elementary schools, the local college, and at parks, and a city-wide health communication campaign to promote sun-safe behaviors and increase sunscreen use.
Recommended readings, refs. 5–12 .
Therapeutic prevention/chemoprevention
Sunscreens remain the only agents proven to reduce melanoma risk, as demonstrated by a randomized controlled trial in Australia. Given the limitations of applying topical products and other concerns related to sunscreens discussed above, if a safe and effective oral drug was available, it would likely be widely adopted as an alternative or adjunct to sunscreen. However, there are many obstacles to demonstrating efficacy of a new melanoma chemoprevention agent, including long latency of melanoma development, potential hazards of long-term drug administration, and large numbers of patients and high cost needed for a randomized controlled clinical trial.
Consequently, most of the work on melanoma chemoprevention has focused on repurposing drugs and natural products that may protect against one or more of the deleterious effects of acute UV exposure. For example, antioxidants have the potential to reduce UV-induced oxidative stress and consequent DNA damage, although positive results in human studies are lacking. Oral N-acetylcysteine (NAC) delayed development of UV-induced melanoma in a mouse model, but NAC did not protect nevi against UV-induced oxidative stress in human subjects. Broccoli sprout extract, which contains the antioxidant sulforaphane, protects against UV-induced skin damage and development of UV-induced squamous cell carcinoma in mice, and is currently being evaluated in patients with clinically atypical nevi. There is also some concern that antioxidants may promote melanoma metastasis based on studies in mice, but studies are lacking in patients with melanoma. Nicotinamide, the amide form of vitamin B3, was shown to reduce incidence of new keratinocyte carcinomas by 23% in patients with prior history of skin cancer. Although equal numbers of melanomas developed in the treated and control groups, the study was not powered to detect an effect on melanoma incidence. Given the capacity of nicotinamide to promote DNA repair in UV-treated melanocytes, and the association with T-lymphocyte infiltration in melanomas in humans treated with nicotinamide, there is some enthusiasm for pursuing nicotinamide as a melanoma prevention agent. Finally, anti-inflammatory agents, such as aspirin and other nonsteroidal anti-inflammatory drugs (NSAIDs), can target UV-induced inflammation and thereby, potentially reduce oxidative stress and procarcinogenic signaling. However, studies on the effects of aspirin and NSAID use on melanoma risk are conflicting, and there has never been a prospective trial in high-risk subjects. In addition to their potential use as oral agents, there is also the possibility of combining these agents with sunscreen to enhance efficacy.
Members of the Melanoma Prevention Working Group published a comprehensive review in 2019 on candidate prevention agents and requirements for moving forward into phase III clinical trials, which is included in the recommended reading below.
Recommended readings, refs. 13, 14 .
Melanoma risk prediction
Genetic testing.
There are increasing complexities and opportunities with multi-gene panel testing for germline mutations implicated in melanoma risk. Genetic risk counselors are now able to select 80 or more bona fide cancer susceptibility genes for evaluation in patients with a family history of melanoma. This reduces the likelihood of missing a pathogenic mutation in a known melanoma gene (e.g., CDKN2A, CDK4, BAP1 , and MITF-E318K ). However, multi-gene panel testing also identifies a number of variants of unknown significance (VUS) and secondary findings (e.g., BRCA2 mutation in a patient with melanoma). The American College of Medical Genetics and Genomics has published recommendations for reporting of secondary findings in clinical exome and genome sequencing, and by extrapolation, those for multi-gene panel testing. In the latest (2019) American Academy of Dermatology Guidelines, genetic risk evaluation by a trained counselor is recommended for individuals with features of hereditary melanoma, including melanoma tumor syndromes with germline mutations, that confer risk for visceral malignancies (e.g., CDKN2A and pancreatic cancer, BAP1 and mesothelioma, and MITF-E318K and translocation kidney cancer). Thus, testing should be performed by a broadly trained cancer risk genetic counselor, medical geneticist, or other trained medical provider with access to care for other at-risk malignancies beyond melanoma (e.g., gastroenterologist for CDKN2A mutation carriers and breast cancer surgeon for BRCA2 mutation carrier).
Because familial melanoma may result from shared environmental risk factors, as well as heritable mutations, all patients with familial melanoma, regardless of genetic status, should continue to engage in strict sun protection and routine skin cancer surveillance. The lack of a detectable variant, even with panel testing, does not exclude the possibility of a high-risk mutation somewhere in the untested genome. Thus, individuals without identified germline pathogenic variants are not completely risk free. If a variant is detected, there are multiple possible scenarios. First, a pathogenic mutation is identified in an “actionable” gene, such as CDKN2A . The National Comprehensive Cancer Network recently endorsed annual pancreatic cancer screening for individuals with germline CDKN2A pathogenic variants regardless of family history of pancreatic cancer, although this practice differs by institution. Individuals with deleterious germline CDKN2A/p16 mutations should generally be referred to a gastroenterologist for a discussion regarding the implications of their carrier status on pancreatic cancer risk. Second, a pathogenic mutation may be detected in a secondary actionable gene, such as BRCA2 . In this situation, genetic risk counselors and/or providers should be able to help refer the individual to the proper management team. Third, a pathogenic mutation may be found in a “nonactionable” gene, such as POT1 . There are currently no specific guidelines regarding the management of individuals with germline POT1 mutations, and, therefore, the default surveillance for patients with familial melanoma should suffice. Fourth, a VUS may be detected. In the absence of functional information, there is little that can be gleaned from the mutation alone. Strict sun protection and skin surveillance should be reinforced in patients with a VUS; however, additional screening for visceral malignancies is usually not warranted. Probands should be encouraged to inform other family members, especially if clinically actionable results are found later, because similar risks are shared by all carriers.
Direct-to-consumer or direct-to-physician marketing of genetic tests of melanoma susceptibility in the absence of training to understand the complexities of testing, such as VUS, may lead to harms, such as unnecessary fear and concern, as well as overuse of cancer screening diagnostic tests that may have limited impact on actual cancer outcomes. The American Academy of Dermatology melanoma clinical practice guidelines specifically recommend genetic counseling with a trained provider, to guard against misinformation and misguided clinical activities.
Recommended readings, refs. 15–18 .
Melanoma underreporting to state cancer registries: generating a nationwide initiative to overcome this challenge
It is estimated that cutaneous melanoma underreporting to U.S. state (and therefore national) cancer registries ranges from 30%–70%. Lack of understanding the true melanoma incidence in the United States adversely impacts resources needed to more effectively prevent, diagnose, and treat the condition. Nationwide initiatives, driven by organizations such as the American Association of Dermatology and state medical boards, could work to enhance practitioner awareness of state-based melanoma reporting requirements and to improve efforts by state cancer registries to facilitate reporting in community settings. For instance, diagnostic pathology/dermatopathology reports could highlight the responsibility of the practitioner establishing the diagnosis to report the melanoma, and offer guidance on the relevant state cancer registry information to aid the provider in doing so. This concept has already been successfully enacted in Arizona. Effective approaches to overcome this challenge in the United States may be useful to improve this issue worldwide.
Recommended reading, ref. 19 .
Secondary prevention
Melanoma screening efforts.
The success of early detection as a secondary prevention modality is dependent on identification and treatment of early, prelethal melanoma. This requires an approach that: (i) reaches individuals at risk and educates them about skin self-exams and melanoma clinical warning signs, (ii) assures access to healthcare providers who can identify melanoma at its early stages, and (iii) results in appropriate curative treatment. An ideal early-detection program must also assure that the benefits of early detection exceed the harms, including minimizing biopsies of benign lesions and overtreatment of relatively indolent skin cancers that can drive up costs of screening with little added benefit. Early-detection programs have been attempted in Germany, Australia, Belgium, France, and the United States. A recent evidence-based review has concluded that collectively, the data suggest that screening programs are beneficial, but that the evidence is not strong. We await additional data from these screening programs, especially Germany's nationwide effort, to determine whether the detection of thinner primaries translates to meaningful reductions in overall melanoma mortality.
The Oregon statewide War On Melanoma early-detection effort has developed a three-pronged approach to reach the lay public, skin service professionals (e.g., massage therapists, hairdressers, aestheticians, etc.), and primary care providers ( www.WarOnMelanoma.org ). Outreach is through an unbranded public health campaign and includes in-person and online educational resources at www.StartSeeingMelanoma.com . Specific strategies in Oregon to improve access to dermatologic care includes e-visits, e-consults, teledermoscopy, and mobile app mole-tracking with MoleMapper. The program is testing the hypotheses that implementation of this campaign will increase awareness and intention to self-screen, improve prognosis at the time of diagnosis, reduce mortality due to melanoma, and reduce the cost of care for melanoma. Early efforts for expansion to other states within the United States have begun in California (entitled Wipe Out Melanoma – California), Arizona, Missouri, Utah, Texas, Georgia, and Massachusetts.
The University of Pittsburgh Medical Center (Pittsburgh, PA) implemented a program that educated primary care physicians about skin cancer detection and encouraged an annual skin cancer screening examination of any patient age 35 years and older presenting for a routine visit. This initiative resulted in an increased rate of melanoma detection, as well as diagnosis of thinner melanomas among screened versus unscreened individuals. Although concerns about causing patient anxiety have been raised as a possible harm of skin cancer screening, a survey of patients found that in comparison with patients who did not have a full skin examination, those who did undergo a full skin examination did not differ on negative psychosocial measures and actually scored higher on measures of positive psychosocial wellbeing. Screening was also not associated with an increase in dermatology visits or skin surgeries.
Establishing a successful early-detection program requires a broad range of expertise and technology. Funding for dissemination and implementation science is needed to assure that progress is made through cooperation across the entire spectrum of stakeholders, including patients and patient advocates, primary care and melanoma specialists, laboratory scientists, public health and population scientists, and engineers.
Recommended readings, refs. 7, 20–25 .
Role of digital imaging, noninvasive technologies, and artificial intelligence in melanoma diagnosis
Digital imaging has become ubiquitous in society following the rapid development of high-resolution mobile devices (up to 108 megapixel sensors). Two-dimensional and three-dimensional (3-D) total body photography (TBP) using semiautomated devices continue to be developed with the goal of increasing image quality and speed of acquisition. Digital follow-up with serial imaging has also proven to be useful in the surveillance of high-risk populations by providing the added benefit of not overlooking cutaneous melanomas that have few dermoscopic criteria, while minimizing the excision of benign lesions. The benefits of TBP and serial dermoscopic imaging have been endorsed by recent consensus recommendations from pigmented lesion experts in the United States. Efforts are underway to execute the first randomized clinical study to compare routine clinical care with an intensive skin surveillance program consisting of 3-D TBP, sequential digital dermoscopy, and melanoma-risk stratification in a high-risk melanoma cohort in Australia. While digital images accurately document the appearance of skin lesions, they can also represent a threat to patient privacy. Therefore, proper measures need to be in place to safeguard this sensitive data. A lack of standardization in image acquisition and storage limits the current utility of this technology.
With the continued expansion of imaging databases, and recent developments in computer science, the implementation of artificial intelligence (AI) in the melanoma diagnostic field is gaining interest. Access to large data pipelines is the primary barrier to developing clinical level AI, as the development and training of such algorithms require large datasets of standardized images tagged with a “ground truth” diagnosis. AI implies that computers can simulate intelligent behavior, while machine learning is the application of AI to allow the system to learn on its own and improve over time. Augmented intelligence is the intersection of machine learning and other advanced applications to work with and enhance human tasks. In the AI arena, machine learning and computer vision are particularly applicable to cutaneous melanoma diagnosis, aiming to augment clinician decision-making, enhance human intelligence, and optimize the physician–patient relationship.
Multiple publications have demonstrated that various convolutional neural networks, a type of deep learning algorithm, can do well in binary clinical or dermoscopic image classification (benign vs. malignant) of skin neoplasms, including melanoma, and keratinocyte cancers, with the convolutional neural networks consistently performing as well if not better than selected groups of expert clinicians. However, studies to date have been done in an experimental setting (“ in silico ”) and have not yet been applied to the real-world clinical setting.
The creation of standardized and safe imaging documentation represents a powerful opportunity to enhance serial skin self-examination and facilitate objective and accurate skin cancer screening by medical providers. It also creates a critical tool for following patients with atypical nevus phenotype, and establishes robust databases for the development of reliable machine learning algorithms for early melanoma identification. All AI platforms require prospective clinical testing and randomized controlled trials to assess their diagnostic capabilities versus that of the healthcare provider, and must demonstrate improved sensitivity, specificity, and cost effectiveness before becoming part of routine clinical practice.
In addition to the implementation of standardized digital imaging (with and without AI support), there has been an explosion of other noninvasive imaging and molecular diagnostic technologies designed to provide a “bedside” diagnosis of cutaneous melanoma. These include existing FDA-approved technologies (e.g., reflectance confocal microscopy and electrical impedance spectroscopy) and emerging technologies (e.g., optical coherence tomography, polarized light, photoacoustic imaging, Raman spectroscopy, and elastic scattering spectroscopy). Current molecular diagnostic techniques include adhesive patch gene expression profiling, utilizing PRAME and LINC . These technological advances have the potential to positively impact the field by providing better access, improved triage, and more accurate diagnosis for patients; however, they require vigilance to ensure that they are clinically implemented following appropriate validation. Specifically, these modalities must be tested against “real-world” clinical interpretation (i.e., dermatologist visual inspection with dermoscopy, utilization of TPB/mole mapping, and/or serial digital dermoscopy in patients with high-risk nevus phenotypes). As yet, they do not replace the gold standard of histopathologic examination.
It is anticipated that effective integration of prospectively validated noninvasive diagnostic devices, including AI and augmented AI platforms, will be paradigm changing for automated diagnosis of skin cancer, including melanoma, and may greatly enhance primary care–based diagnoses and prioritize patient referrals to dermatologists in rural areas with specialist shortages.
Recommended readings, refs. 26–28 .
Need for increased specificity in melanoma diagnosis: healthcare cost implications
Provider sensitivity for diagnosis of cutaneous melanoma is not perfect; low provider sensitivity results in melanomas diagnosed at more advanced stages, which necessitates more intensive therapeutic courses, incurs additional personal and financial morbidity for the patient, and has a higher mortality risk. Appropriately validated quality metrics are important to guide continuous improvements in early-melanoma detection as they quantify performance with melanoma screening examinations, identify opportunities for additional educational intervention, and measure changes in provider diagnostic sensitivity associated with introduction of novel diagnostic support technologies. Despite these potential benefits, quality metrics for cutaneous melanoma diagnosis have remained elusive. The most commonly cited metric is the “number needed to biopsy” (i.e., how many skin biopsies are necessary for a melanoma diagnosis). This gives an approximation of the provider's positive predictive value, but is of limited practical application when the provider's sensitivity, specificity, and melanoma prevalence are unknown.
Development of clinically appropriate, feasible, and validated quality metrics for melanoma detection, including sensitivity, is best guided by the clinician end-users, yet the absence of structured clinical and pathology data limits practical metric development. Advances in native language processing offer opportunity for evaluation of structured pathology diagnosis fields, but interpretation of more heterogeneous clinical impression data fields remains challenging for this technology. Measurement of overlooked melanomas (false negatives) remains particularly challenging, as the “diagnostic moment” of the full skin examination is not currently captured. Similarly, the absence of an International Classification of Diseases, Tenth Revision code structure for “skin cancer screening” examinations hinders efforts to retrospectively identify patients who received screening examinations, thus complicating efforts to quantify “number needed to screen” or similar outcomes measures.
Performance metrics, when paired with opportunities for clinical practice improvement, have the potential to optimize patient comfort and satisfaction, improve clinicians' self-efficacy regarding noninvasive diagnosis, improve busy clinicians' allocations of time and effort (for both dermatologists and pathologists), and reduce healthcare costs. The cost of adjuvant systemic therapy (e.g., 1 year of adjuvant pembrolizumab estimated at more than $160,000 for drug cost alone) versus the cost of one diagnostic biopsy (∼$259), suggests that increasing allocation of resources to comprehensive early-detection initiatives may be worth considering to reduce total healthcare costs.
Recommended readings, refs. 29, 30 .
Tertiary prevention as it relates to melanoma survivorship
Tertiary prevention focuses on avoiding or reducing the sequelae of a disease process once established in an individual. It may be integrated into treatment considerations and has considerable overlap with “survivorship.” Recent statistics from the American Cancer Society indicate that there are more than 1.3 million melanoma survivors living in the United States; nearly half of those survivors are under the age of 65 years. Advances in treatment of systemic disease with immunotherapy and targeted therapies have caused dramatic decreases in mortality from melanoma: in the years 2013–2017, the American Cancer Society reported a 7% decrease in melanoma-related deaths annually for patients ages 20–64 years and a 5%–6% decrease in melanoma-related deaths annually for those more than 65 years old. However, survivorship can pose multiple issues, including those of second primary melanomas, increased risk of other skin cancers, and long-term consequences from treatment of melanoma. Immunotherapy with programmed cell death protein 1 receptor (PD-1) inhibitors, for example, can cause permanent autoimmune adverse effects, including hypothyroidism, adrenal insufficiency, and diabetes. Current work in this area focuses on guidelines for management of these and other side effects of therapy, while maintaining the efficacy of treatment. The development, documentation, and implementation of formal survivorship plans for individuals treated with curative intent is another approach to decrease associated morbidity and improve patient quality of life.
Recommended readings, refs. 31, 32 .
Recommendations for future prevention research are summarized in Table 1 .
Prevention and early-detection recommendations.
The clinical importance of defining dormancy and its underlying mechanisms
Clinical cancer dormancy is the period of time a patient who ultimately recurs was clinically free of detectable disease, defined in melanoma from the time of treatment of the primary lesion until the appearance of metastasis or other recurrences. But dormancy is more than simply subclinical growth, the concept implies a period of time where clinically undetectable residual tumor persists, but does not actively proliferate. In melanoma, there are many cases where patients recur after a disease-free interval spanning a decade or more after initial removal of the primary lesion, but whether the underlying mechanism(s) for this prolonged disease-free interval truly reflects dormant cells (as opposed to slow, but steady, growth of a very small inoculum of residual tumor cells) has never been determined. Traditionally, studies of dormancy were largely based on cultured cells, mouse models, or patients who were untreated or subjected to rather ineffective treatments. As staging and prognostication become more precise and treatments become more effective, a large fraction of patients with resected stage III, and someday even stage II, disease is expected to be subjected to adjuvant therapy, typically for up to a year. Adjuvant therapy may alter the kinetics of growth of any residual cells, and cells entering dormancy might retain a degree of sensitivity to the treatment administered, but survive long enough to recur after treatment discontinuation. Understanding this phenomenon is, therefore, critical to the rational designing of both adjuvant therapy regimens and salvage treatments in patients recurring after completion of adjuvant therapy.
Here, we highlight pending questions in the context of melanoma dormancy that provide exciting opportunities for research and innovation at basic and clinical levels. In addition to understanding what drives the onset of dormancy, we need to identify signatures that maintain the dormant state(s), and conversely, those that allow for “awakening” of dormant cells. The extent to which persister cells may evolve with time (genetically and epigenetically) is an active area of research. It is also unclear how the fate of dormant tumor cells is modulated in an organ-specific manner. For example, are mechanisms governing asymptomatic, or “silent” metastasis in the brain similar to those in lung, liver, or other distal sites?
Another unresolved question is the role of the immune system with respect to dormant versus active metastasis. Below we offer some ideas as to how to leverage models and human sample analysis to help address these unmet needs. In particular, we discuss opportunities for developing strategies to detect disseminated melanoma cells and to monitor their presence as dormant or active cells in patients.
Animal models to visualize and characterize melanocytes and melanoma progression
One of the challenges with studying dormancy in vivo is the paucity of imaging tools and physiologically relevant animal models. These limitations are being addressed by using highly sophisticated models in zebrafish, as well as in genetically engineered mouse (GEM) models. In both settings, it is now possible to trace tumor cells in living animals and assess the contribution of various risk factors, such as UV radiation or aging. Other mouse models are being generated to monitor specific roles of the vasculature and distinct components of the tumor microenvironment. The development of GEM models for depletion of selective immune cells or modification of stromal compartments is also expanding our ability to analyze cell–cell interactions. Similarly, new tools for intravital imaging in zebrafish and the possibility of cost-effective genetic and pharmacologic screens in these animals represent great platforms for in-depth studies of melanocytic cell populations and their differential potential to self-renew and enter into programs of cellular quiescence (dormancy). They also offer the opportunity to monitor proliferation of such cells in basal conditions or in response to intrinsic and/or extrinsic prooncogenic signals.
Detection, isolation, and monitoring of dormant melanoma: human to mice and vice versa
Animal models may be very useful in defining mechanistic aspects of melanocyte biology and tumor cell dormancy/awakening as mentioned above, but a main challenge is to translate this knowledge into reliable prognostic biomarkers and response indicators. Progress in this field will necessarily involve a comprehensive and multidisciplinary characterization of human specimens (primary lesions, liquid biopsies, and recurrent/metastatic tumors).
Tissue collection
The question of what/when tissues should be collected in retrospective versus prospective analyses is always an important one. This has become critical as larger cohorts of patients are proceeding to neoadjuvant intervention, where agents are administered before local treatment and before detection of clinical visceral metastases. Consensus is building that sampling should go beyond the primary lesion and sentinel lymph nodes. Bone marrow may represent a screening platform if dormancy cues and regulators identified in model systems can be validated in comprehensive studies in human patients.
Regarding markers for monitoring disseminated melanoma cells, pigmentation-associated markers could be used to identify tumor cells in sentinel lymph nodes and bone marrow. This could also be the case to detect circulating tumor cells in blood. A consideration, however, is that pigmentation markers may be lost as melanoma cells switch into invasive phenotypes. Consequently, methods to detect specific genetic alterations, such as gains, losses, or translocations characteristic of melanoma, may allow the pinpointing of amelanotic cancer cells. Technologies for single-cell analyses in situ or in dissociated biopsies are likely to represent a tractable platform for gene discovery, but there is a need for systematization/homogenization of protocols and bioinformatics analyses. Access to biobanks or repositories of specimens linked to clinical trials are also key. In particular, probing the cellular state of residual tumor cells may help understand long-term effects of immunotherapy agents that may drive melanoma cells into dormancy.
Liquid biopsies
An area of further research in melanoma and other cancers is the use of blood or serum to monitor for active and dormant residual disease. The mutational status of cell-free DNA (cfDNA) can be explored as a strategy to predict or anticipate a soon-to-occur relapse. This may also be the case for DNA or RNA contained in exosomes. Analysis of samples obtained at the time of surgery will be important as a baseline control, but also at multiple timepoints during follow-up, to determine whether patients carry dormant or proliferative disseminated cells after surgery or after adjuvant treatment. Limitations with respect to sensitivity should be addressed, and consideration should be taken into account that dormant lesions may not necessarily shed circulating cells to the same extent as actively proliferating tumors. Therefore, from a clinical perspective, it may be more practical to pursue circulating tumor cells in the context of drug response in advanced stage IV patients, rather than in stage II–III tumors.
Given the considerations posed above for the detection of circulating cells, an alternative possibility to gauge the potential for tumor cell relapse is to test for proteins in serum that have been linked to dormancy induction/maintenance (i.e., WNT5A, SOX9, N2RF1, or TFGB2) or that have been linked to dormancy break (i.e., uPAR, FBXW7, FAK, or DKK1). Conceptually, it should be possible not only to probe tumor-associated mutations or gene signatures, but also to assess secreted factors from the host. Such an approach has not yet been tested.
Rapid autopsy programs
Rapid autopsy programs have been set up in some academic medical centers to sample many organ sites immediately postmortem to collect metastatic tumors and clinically uninvolved tissues, where subclinical disseminated tumor cells may reside. These efforts could improve our understanding of the complexity of end-stage disease and the systemic nature of melanoma spread. In studies of prostate cancer, rapid autopsy programs have provided information regarding the extent of metastatic disease. However, studies of dormancy with postmortem biopsies have not been performed in melanoma. As for other sampling procedures, the question remains of whether characterizing dormant cells that never reactivated in deceased patients will be clinically informative for other prospective studies. Similarly, it will be important to determine whether disseminated tumor cells identified at a given timepoint reflect the actual (epi)genetic changes that happened at the site of origin years earlier (i.e., to study tumor cell evolution). In this context, it will be useful to compare disseminated tumor cells in patients with detectable metastases with those identified only postmortem, looking at cases who had complete resection versus those who had responded previously to a systemic therapy, but then relapsed. This would perhaps distinguish different stages of dormancy from irreversible arrest programs, such as senescence.
In summary, the field of tumor dormancy is nascent, but burgeoning. Outstanding needs include more physiologic animal models for in vivo studies and a better knowledge of the plasticity of human melanomas in naïve versus treated patients. Intriguing data are emerging regarding aspects of tumor cell tropism to different organs, but it will be important to dissect in more detail the impact of the microenvironment at large. From a clinical perspective, the field needs more sensitive imaging tools for the detection of micrometastases, as well as for the processing of liquid biopsies. What and when to collect specimens for analyses in patients who have remained asymptomatic for years or after long-term treatment is also a challenge. Moreover, biopsies should be considered beyond the primary tumor that may inform about (pre)metastatic niches. As we design therapies to address tumor dormancy, we need to be aware of long-term effects of sustained treatment. Overall, addressing the potential of dormant tumor cells to grow and colonize distal sites will be critical to overcoming the burden of recurrent disease.
Recommended readings, refs. 33–37 .
Recommendations for future tumor cell dormancy and metastasis research are summarized in Table 2 .
Dormancy/metastasis recommendations.
With the publication of large-scale genomic data in cutaneous melanoma and uveal melanoma as part of The Cancer Genome Atlas (TCGA) and other large studies that included cutaneous, as well as mucosal and acral melanoma subtypes, the landscape of targetable mutations in melanoma is coming into focus. While translation has only led to therapeutic breakthroughs in patients whose tumors harbor BRAF V600 mutations, ongoing research is underway to determine how to optimally target these alternative genetic aberrations. Additional targetable mutations could be addressed over the coming years to maximize the benefit of targeted therapy for patients with melanoma.
Clinical experience for BRAF-targeted therapies
The past decade has been an unquestioned success with the development of effective BRAF-targeted therapy for patients with BRAF V600 -mutant melanoma. The safety and efficacy data of combined BRAF-MEK inhibition have led to regulatory approval of three regimens (dabrafenib + trametinib, vemurafenib + cobimetinib, and encorafenib + binimetinib) in the metastatic setting and one (dabrafenib + trametinib) in the adjuvant setting for stage III melanoma. Furthermore, recent long-term data from three phase III trials (co-BRIM, pooled data from COMBI-V and COMBI-D) of BRAF-MEK combinations show 5-year progression-free survival (PFS) in 14%–19% of patients, with 5-year overall survival (OS) of 31% and 34%, respectively, for vemurafenib + cobimetinib and dabrafenib + trametinib. These numbers are even better for patients with a normal versus elevated lactate dehydrogenase (5-year PFS, 18%–25% vs. 8%–10%), those with a performance status of 0, and those with fewer than three organs involved with melanoma. Furthermore, 5-year follow-up results from the adjuvant study of dabrafenib + trametinib versus placebo (COMBI-AD) showed a 16% absolute difference in the percentage of patients who were relapse free after the application of combination BRAF-MEK inhibition in the adjuvant setting compared with patients undergoing observation. And yet, despite this success, there are several issues that must be addressed to optimally use these agents.
First, there are no data that indicate whether any of the three different BRAF-targeted therapy regimens are superior in efficacy. Data suggest that efficacy is similar across regimens, with each regimen showing similar relative efficacy compared with single-agent vemurafenib in randomized phase III trials. Interestingly, the COLUMBUS trial included an arm of single-agent encorafenib, which demonstrated superiority to vemurafenib. Thus, single-agent encorafenib may be more efficacious than single-agent vemurafenib; however, it is not clear from the data across trials that encorafenib + binimetinib is more effective than vemurafenib + cobimetinib. Thus, the key distinguishing features of the regimens are number of pills per day, timing of administration vis-à-vis food intake, toxicity, and pharmacokinetics/half-life.
Second, the great success of BRAF-targeted therapy has been overshadowed by immune checkpoint inhibitor data, either single-agent PD-1 inhibition or combined anti-PD-1 and anti-CTLA-4 (cytotoxic T-lymphocyte-associated protein 4) inhibition. With 5-year OS of 44% and 52%, respectively, and an enhanced ability to maintain disease control after stopping therapy, immunotherapy has become favored as initial therapy for patients with BRAF V600E melanoma. However, results from ongoing randomized trials comparing the sequence of combined immune checkpoint inhibition with BRAF-targeted therapy at disease progression or the reverse sequence (DREAM-seq and NCT02224781, and SECOMBIT and NCT02631447) are needed to provide level 1 evidence to support optimal therapy choices in the first-line setting. Unfortunately, there are no widely adopted pretreatment biomarkers that can be used to help select patients with BRAF V600 melanoma most likely to benefit from immunotherapy or BRAF-targeted therapy. It is hopeful that the two clinical trials launched to address the question of “which type of therapy first?” will also help answer the more nuanced question of “which therapy for which patient?”
Third, while as many as 15%–25% of patients, depending on risk factors, may remain progression free 5 years after starting treatment with BRAF-MEK inhibitors, the majority of these patients remain continuously on treatment. Data have suggested that 40%–50% of patients who discontinue treatment either voluntarily or due to toxicity while still in response will experience disease progression within 6–9 months. While many of such patients can respond to resumption of therapy, data are limited and anecdotal. More information is needed to determine which and when patients can safely stop BRAF/MEK-targeted therapy and what the impact of stopping is on patient outcome and quality of life.
Fourth, translational research studies have consistently shown that BRAF/MEK inhibition is associated with increased CD8 + T cells, T-cell cytotoxicity, and melanoma differentiation antigen expression, as well as decreased immunosuppressive cytokines within metastatic melanoma deposits. These data have been used to support the development and testing of triplet combination regimens involving BRAF/MEK and PD-1 pathway inhibition. Recent results from the IMSPIRE 150 and Keynote 022 trials show that these triple combinations can produce improved median PFS and duration of response relative to targeted therapy alone. However, despite these encouraging correlative studies and clinical results, it remains unclear whether the tumor biopsy findings are a consequence of enhanced antitumor immunity or merely tumor cell death and depletion and whether the early clinical findings result from synergistic, additive, or even subadditive effects of the combination. More research is needed on the impact of BRAF/MEK inhibition on antitumor immunity and determining how the triplet combinations compare with ipilimumab + nivolumab immunotherapy with subsequent targeted therapy upon progression in terms of critical endpoints, such as OS, cost, toxicity, and the ability to allow treatment-free survival (time without treatment or toxicity).
Finally, there are also no data to help determine which patients with resected stage III BRAF-mutant melanoma should preferentially be offered adjuvant BRAF-targeted therapy or anti-PD-1 therapy. The COMBI-AD trial comparing adjuvant dabrafenib + trametinib with placebo showed a 5-year recurrence-free advantage of 52% versus 36% compared with placebo, suggesting that a significant proportion of stage III patients with BRAF-mutant melanoma harboring minimal residual disease may be cured with an year of adjuvant-targeted therapy. Identifying who these patients are and whether they differ from those getting long-term benefit from adjuvant immunotherapy are critical unmet needs. In addition, we need information regarding the optimal duration of therapy in the adjuvant setting, a risk–benefit analysis of BRAF-targeted versus anti-PD-1 therapy, and more data regarding whether these regimens may be as or more effective in the neoadjuvant setting. Notably, several small neoadjuvant trials have demonstrated that major response or pathologic complete response (CR) is associated with objective clinical endpoints, such as recurrence-free survival. This suggests that the neoadjuvant setting may be a place to vet promising targeted therapy regimens using pathologic CR as a key metric of potential efficacy, along with a number of correlative endpoints to better understand the effects of therapy on the tumor and its microenvironment.
Non-BRAF–targeted therapies
The second most common oncogenic driver mutation in melanoma occurs in NRAS , a target that currently lacks a specific inhibitor. Preclinically, inhibitors of the MAPK pathway, particularly pan-RAF, MEK, and ERK inhibitors, have shown promising efficacy. Unfortunately, despite modest benefits of MEK and ERK inhibitors in small trials in patients with NRAS-mutant melanoma, a randomized phase III trial of the MEK inhibitor, binimetinib, compared with dacarbazine demonstrated only a modest benefit in PFS and no difference in OS. On the basis of these data, the clinical testing of single-agent MAPK inhibitors in this setting was discontinued. Large-scale national basic research efforts (see below) are focused on identifying novel inhibitors of activated KRAS pathway, raising the possibility that such efforts may ultimately benefit patients with NRAS melanoma.
Targeted therapy resistance
The great majority of patients with BRAF -mutant melanoma treated with targeted therapy ultimately will require additional therapy due to primary (intrinsic) or secondary (acquired) resistance. A deeper understanding is needed to better address resistance in all melanoma subtypes and to inform future therapy development. It is key that patients treated with standard-of-care BRAF-targeted therapy are enrolled into correlative protocols that allow, when feasible, serial biopsies and blood analyses, and that clinical trials of targeted therapies build in mandated biopsies and blood analyses. Such collection of patient samples in a dedicated fashion is necessary to more fully understand the therapeutic resistance landscape. Preclinical studies informed by clinical samples, followed by translation of preclinical breakthroughs are expected to lead to next generation, transformative therapies.
Molecular landscapes
In recent years, sequencing studies have revealed that cutaneous melanomas, which commonly arise from sun-exposed surfaces of the body, harbor a high mutational burden and a UV mutation signature, but infrequent structural rearrangements. In contrast, mucosal and acral melanomas have a low mutational burden and rarely display a UV mutation signature. The most common genetic alterations present in cutaneous melanoma provide a rationale for genomic subclassification of these patients. Specifically, BRAF, NRAS , and NF1 mutations, which are largely mutually exclusive drivers of MAPK activation, comprise three genomic subtypes established by TCGA in cutaneous melanoma. A fourth, so-called triple wild-type (WT) tumors, comprise cases that lack these mutations. Nevertheless, even triple WT tumors show an activated MAPK pathway due to autocrine and paracrine growth factor stimulation.
BRAF mutation classifications
BRAF V600 (class I) mutations are the most prevalent in melanoma, and in light of the availability of specific inhibitors, represent the only clinically actionable genetic subtype to date. Class II BRAF mutations are RAS independent, with intermediate to high kinase activity. In contrast, class III BRAF mutations are RAS dependent, with low kinase activity. Class II and III mutations are generally not sensitive to current BRAF-targeted agents.
NRAS mutations
NRAS mutations are typically alterations of the Q61 residue, which hyperactivates its ability to phosphorylate downstream targets. Advances in the understanding of RAS structures, interactors, posttranslational modifications, and activity have led to a variety of promising preclinical strategies to develop RAS-targeting agents, but no RAS-targeted agents are yet approved for clinical use.
NF1 mutations
NF1 loss-of-function mutations are also core melanoma drivers. NF1 enhances hydrolysis of RAS-bound GTP to restrain downstream MAPK activity. NF1 mutations frequently cooccur with mutations in RASopathy genes, such as RASA2 and PTPN11 , encoding SHP2 and MAP2K1/2 . These cooccurring mutations are thought to further amplify MAPK signaling to drive tumorigenesis, where NF1 loss of function alone may be insufficient. Despite MAPK activation as a critical driver of NF1 -mutant melanoma, there is scant evidence that MEK inhibitors or other targeted agents are efficacious in NF1 -mutant melanoma. SHP2 inhibitors represent a promising class of targeted therapies for RAS-GTP–dependent cancers, including those with NF1 mutation or class 3 BRAF mutations. SHP2 functions downstream to integrate growth factor signaling and RAS activity. Allosteric SHP2 inhibitors designed to subvert RAS activity are currently in early clinical development.
Triple WT melanomas
Triple WT tumors can harbor KIT amplification, mutation, or structural rearrangement, or mutations in HRAS, KRAS, GNAQ , or GNA11 . KIT alterations are found most frequently in acral or mucosal melanomas. Tumors with activating KIT mutations in exons 9 or 11, although not amplification, have responded to tyrosine kinase inhibitors that target KIT, such as imatinib, which are largely ineffective against other melanomas. In addition to the main core driver mutations described above, other common alterations have been documented, which may be actionable with existing drugs in clinical use or development. Alterations in cell-cycle regulators (i.e., CDKN2A loss, CDK4 or CCND1 amplification, and ALK translocations) provide a rationale to combine CDK4/6 inhibitors, such as palbociclib and ribociclib. Palbociclib monotherapy has shown efficacy in patients with acral melanomas with CDK alterations, as well as synergy with BRAF and MEK inhibitors in cell line models. Similarly, genetic alterations in the PI3K/AKT/PTEN pathway have supported preclinical studies with PI3K inhibitors. Unfortunately, none of these small molecules have shown promise as either single agents or combinations against metastatic melanoma. Alterations in the MDM2/p53 axis are relatively less common in melanoma (<20% of patients), but could be targeted with second-generation MDM2 or MDMX inhibitors that restore p53 levels and/or function, such as ALRN-6924, currently in phase I clinical trials.
Various analytic approaches have nominated a number of additional potential therapeutic targets. Recurrent point mutations in IDH1 at R132 are observed in several cancer types, including 3%–5% of melanomas. Ivosidenib is an inhibitor of mutant IDH1 that was recently approved for use in acute myeloid leukemia. Efficacy in IDH1-mutant melanoma has not been established. A hotspot mutation in RAC1 (P29S/L), a rho GTPase, confers hyperactivity, and is nearly melanoma specific. Numerous strategies to develop rho GTPase inhibitors have been pursued, but have not yet achieved clinical success. Neurotrophic tyrosine receptor kinase (NTRK) fusions have also been noted in melanoma, in particular spitzoid lesions, and may be targetable with newly approved NTRK inhibitors, such as entrectinib.
Uveal melanomas (along with very rare melanomas arising from blue nevi) are characterized by mutually exclusive activating mutations in GNAQ or GNA11 , encoding heterotrimeric Gαq family members. Preclinical studies have found that GNA11-mutant uveal melanomas are initially sensitive to BET inhibitors, and that combined FGFR inhibition overcomes the emergence of resistance to BET inhibitors. Recent studies have found that Gαq stimulates YAP via FAK, identifying these as suitable therapeutic targets in uveal melanoma. A new generation of orally bioavailable FAK inhibitors is currently being tested in phase I trials, offering new promise for patients with metastatic uveal melanoma.
Molecular heterogeneity and phenotypic plasticity
In addition to the diversity of oncogenic driver mutations, there is abundant heterogeneity of melanoma cells between patients, between lesions from the same individual, and within individual tumors. Controversial cancer stem cell models initially reported for melanoma have ceded to models in which phenotypic plasticity of melanoma cells is thought to be a consequence of different transcriptional or cell states. Highly plastic melanoma cells can switch between these different cell states. This cell state switching likely serves to engage specific tumorigenic properties in response to microenvironmental cues or when otherwise needed for tumor growth or survival. Through bulk transcriptional analyses of both patient tumors and cell culture models, transcriptional states characterized by high expression of either AXL or MITF , among other genes, were established. These cell states have been described as “invasive” and “proliferative” classes, respectively. The MITF/AXL cell states have also been characterized by properties related to drug sensitivity. The AXL-high cell state, compared with the MITF-high state, is reported to possess relative resistance to MAPK pathway inhibitors, but sensitivity to ferroptosis-inducing agents, such as GPX4 inhibitors. These cell states were recently confirmed to coexist intratumorally by single-cell RNA sequencing of patient tumors. It is expected that additional single-cell RNA sequencing, spatial profiling, and other advanced phenotypic and functional characterization will further clarify different classes of cells in patient tumors. Specific subpopulations representing a given cell state may exist prior to therapy, for example, NGFR/EGFR + cells, or develop during therapy leading to resistance. Thus, for complete eradication of a tumor, we may need to develop dual strategies that target both the bulk of the tumor and more resistant subpopulations. Because of the apparent heterogeneity and plasticity of melanomas, the aim should be to focus on common pathways, many yet to be discovered. Potential candidate pathways could relate to pigment cell differentiation and to neural crest-like dedifferentiation.
Models for therapy resistance
Melanoma cells have inherent or develop resistance to small-molecule inhibitors that are in clinical use. This intrinsic and/or acquired resistance can be seen and modeled both in vitro in melanoma cell lines and in vivo in human cell line xenografts, patient-derived xenografts (PDX), and GEM models of melanoma. To maintain continuous drug pressure on tumors, mice are fed a diet containing the appropriate inhibitors, achieving drug concentrations in the circulation similar to those used in patients. Xenografts and GEM models have confirmed the correlative studies from patient tissues. In vivo models are ideal to assess mechanisms of resistance and point to main dependencies and vulnerabilities of melanoma cells to better develop new strategies for second-line or rescue therapy. Each type of in vivo model has advantages and weaknesses. The weakness of GEM models is their relative paucity of genetic aberrations, while human xenografts lack a typical tumor microenvironment because their immunocompromised murine hosts lack most immune cells. To overcome the latter, investigators have been working on humanizing the mice by reconstituting them with human hematopoietic stem cells that could differentiate into all blood cell lineages. Ideally, the immune cells and the tumor cells should come from the same patient. However, collecting hematopoietic stem cells from patients with melanoma in sufficient numbers needed for experimental studies in mice is not feasible. A potential alternative is the generation of immune cells from patients through a series of dedifferentiation steps to obtain induced pluripotent stem cells that can then be differentiated to hematopoietic stem cells and thymic progenitor cells. Those technologies are still underdeveloped, but urgently needed to better understand the role of immune cells in the tumor microenvironment for resistance against signaling inhibitors.
Preclinical → clinical development
Ultimately, any potentially transformative preclinical development needs to make it into the clinic. In the past decade, one example of this is the development of combined BRAF-targeted therapy with anti-PD-1/anti-PD-L1 (programmed death-ligand 1) therapy. The initial discovery that BRAF and/or MEK inhibition led to increased melanocytic antigen expression in vitro and in vivo , as well as increased CD8 + T cells and other proinflammatory changes in patient samples, led to several clinical trials of triplet BRAF/MEK/anti-PD-L1 drugs. Moving forward, a number of key factors for clinical development of promising preclinical findings need to be considered. First, each new trial needs a strong rationale from experimental models, not only cell lines but xenograft/PDX and/or GEM models and, if possible, patient samples. Those data need to convincingly show at least tumor stasis or optimal regression. Second, biomarkers should be developed to optimally select patients for trials based on the relative likelihood of benefit or toxicity. Third, with combination therapies gaining favor, it is becoming increasingly critical to reassess timing, sequence, and dose of treatments. To achieve these ambitious objectives, it will become important for clinical and research centers to collaborate, as large-scale trials on highly selected patients are only possible when conducted in multiple centers. Optimally, those multicenter trials would be supported by both pharmaceutical companies and federal agencies, facilitating the ability to acquire requisite pretreatment, multiple on-treatment, and posttreatment samples. Particularly, complex combination trials need extensive evaluation of both the host immune and tumor responses. New findings should be evaluated and validated in experimental models to support future planning for clinical trials. The more information we can gather from each trial, the better we can plan for subsequent trials.
Recommended readings, refs. 38–44 .
Recommendations for future targeted therapy research are summarized in Table 3 .
Targeted therapy recommendations.
Resistance to immune checkpoint inhibitors
Immunotherapy with checkpoint inhibitors has markedly improved and extended the lives of many patients with advanced or metastatic melanoma. While in the early 2000s median OS was 6–9 months, recent clinical trials show 3- to 5-year survival rates exceeding 50%. However, approximately 70% of patients still experience disease progression within 5 years. This includes both de novo (primary) resistance in 30%–50% of patients and an additional 20%–30% with progression after initial benefit (secondary resistance). Further improvements in outcomes from immunotherapy of patients with melanoma will require additional scientific, clinical, and societal advances.
Clinical definitions
Immunotherapy approaches and drug development are evolving rapidly. New agents are commonly evaluated for activity in patients with melanoma who have shown primary or secondary resistance to an immune checkpoint inhibitor. However, because of unique patterns of response and the lack of consistency in the clinical definitions of what constitutes a treatment-refractory patient, the design of clinical trials of new agents and interpretation of results remain a significant challenge. To address this unmet need, the Society for Immunotherapy of Cancer convened a taskforce to generate consensus clinical definitions for resistance to immune checkpoint inhibitors in three distinct scenarios: primary (or de novo ) resistance, secondary (or acquired) resistance, and resistance after therapy has been discontinued. According to the taskforce, the definition of primary resistance to a single agent requires that the patient had received adequate exposure, defined as at least 6 weeks of therapy, and developed either confirmed radiographic progression with two imaging tests at least 4 weeks apart or unequivocal clinical evidence of progression within 6 months of starting treatment and while on therapy. Secondary resistance assumes that the patient had exposure to therapy for at least 6 months, obtained initial clinical benefit [CR, partial response (PR), or stable disease (SD) for at least 6 months], and had either confirmed radiographic progression with two imaging tests at least 4 weeks apart or unequivocal clinical progression. Resistance after stopping therapy, whether after completion of adjuvant/neoadjuvant therapy, following attainment of maximal benefit, or due to severe toxicity, is considered to behave similarly to either primary or secondary resistance, depending on the initial response and the length of time since the last treatment, with 12 weeks being the consensus cutoff between primary and secondary resistance. The goal of this effort was to provide guidance for clinical trial design and to support analyses of data related to mechanisms of resistance.
Recommended reading, ref. 45 .
Mechanistic underpinnings
While the mechanisms for primary and secondary resistance may overlap, they could also be quite different. Understanding the mechanism of the immune response can provide a context for the development of resistance. Cytotoxic T-cell responses are critical to initial tumor destruction and play a central role in preventing recurrence; however, there is increasing evidence for a role of other lymphocyte populations, including B and natural killer (NK) cells, in either enhancing or sustaining the initial immune response. Humoral immune responses to tumor antigens have improved patient outcomes coinciding with the presence of tertiary lymphoid structures within the tumor; these are examples of B-cell contributions. Antibodies targeting membrane antigens can mediate antibody-dependent cellular cytotoxicity or complement-dependent cytotoxicity, which may sustain tumor cell destruction. It is still unknown whether B cells are simply surrogate markers of a productive antitumor T-cell response or are contributing in a meaningful way to the elimination of residual tumor. In addition, NK cells can be activated in response to cytokines released by reinvigorated T cells following checkpoint inhibitor therapy and serve to eliminate residual tumor cells devoid of surface HLA molecule expression.
Mechanisms of primary resistance
Primary resistance can occur even in cases where T cells are present in the tumor microenvironment (“warm” tumors). The immune destruction of tumor cells may be inhibited directly by expression of non-PD-1 pathway inhibitory checkpoints (e.g., TIM-3, VISTA, and LAG-3), the presence of immunosuppressive cytokines (e.g., TGFβ and IL6), or through the recruitment of immunosuppressive cells [e.g., regulatory T cells (Treg), myeloid-derived suppressor cells, and tumor-associated macrophages (type 2)]. Finally, the absence of immune cells in the tumor microenvironment (“cold” tumors) either through loss of antigen expression, lack of T-cell chemokines to prevent migration to tumor sites, or active exclusion triggered by activation of WNT–β-catenin expression or loss of PTEN are all well-established causes of primary resistance.
Mechanisms of secondary resistance
Tumor cells can lose expression of target antigens (shared or neoantigens) or downregulate expression of components of the antigen-processing machinery, including HLA restriction elements. Alternatively, tumor cells may lose expression of PD-L1 via abnormal signaling through the IFNγ pathway. While these resistance mechanisms are well accepted, the resistance mechanisms in the majority of cases remain unknown.
Recommended readings, refs. 3, 46 .
Rational combination strategies
Ideally, combination immunotherapy should involve complementary or synergistic mechanisms that increase the effectiveness of therapy without escalating adverse events. With more consistent definitions, both clinical and mechanistic, combinatorial approaches can more rationally target an appropriate mechanism of resistance.
The presence of a high frequency of tumor-infiltrating “exhausted” CD8 + T cells expressing PD-1 and CTLA-4 is highly predictive of anti-PD-1 response in patients with metastatic melanoma. However, recent research has shown that even PD-1 blockade produces only a limited burst of T-cell proliferation in many animal models. Similarly, circulating peripheral exhausted T cells were found to be only transiently reinvigorated during anti-PD-1 therapy of melanoma. Prolonging this activation through combination of an anti-PD-1 antibody with an anti-CTLA-4 antibody, a 41BB or GITR agonist antibody or cytokine stimulation to produce longer lasting metabolically competent effector cells could result in enhanced T-cell survival and tumor clearance. Ipilimumab, a clinically available anti-CTLA-4 antibody, is thought to act via enhanced T-cell priming, although there are data that Treg depletion or stimulation of exhausted T cells may also play a role.
Given that many melanomas lack tumor-infiltrating lymphocytes, an obvious possibility for combination immunotherapy is an inflammatory signal that attracts cDC1 or cDC2, T cells, or NK cells to the tumor site and sets up an IFNγ/IL12 cascade. This may be achieved by approaches that include intratumoral injections of oncolytic viruses, STING, TLR7/9, as well as cytokines, such as IL12, which can polarize the tumor microenvironment in a Th1 direction.
Finally, another direction for combination immunotherapy is to generate tumor-selective T cells with peptide, RNA, or DNA vaccines. While cancer vaccine research has shown limited progress to date, promising new directions, such as personalized neoantigen or shared antigen vaccines using existing or novel adjuvants, are being actively pursued.
Recommended readings, refs. 47–50 .
Current issues in the management of advanced melanomas
In the treatment-naïve setting, there are currently two main systemic immunotherapy options for patients with advanced unresectable melanoma: anti-PD-1 monotherapy (nivolumab and pembrolizumab) and anti-PD-1 plus anti-CTLA-4 combination therapy (nivolumab + ipilimumab). Other FDA-approved immunotherapies may be reasonable in specific populations, such as talimogene laherparepvec (TVEC) or TVEC coadministered with an immune checkpoint inhibitor, although the latter remains under study.
Brain metastases
Melanoma has the highest propensity of all common malignancies to metastasize to the brain, with 40% of patients expected to develop brain metastases, following the diagnosis of metastatic disease and up to 70% by the time of death from the disease. Therapeutic strategies for melanoma brain metastases have been largely restricted to stereotactic radiosurgery or neurosurgical resection. Whole-brain radiotherapy has shown little therapeutic benefit compared with its benefit in other malignancies.
Combination anti-CTLA-4/anti-PD-1 immunotherapy has demonstrated impressive clinical activity with objective response rates of 50%–60% and median OS that has not yet been reached in up to 5 years in phase II/III studies. This combination in asymptomatic patients, with silent melanoma brain metastases not requiring corticosteroids, produced an intracranial response rate of 55% with 29% CRs. Furthermore, the intracranial PFS rate at 9 months was 67%, far exceeding what has been observed with other therapies. However, the combination still has substantial systemic toxicities with serious adverse events in as many as 55% of patients, encouraging the pursuit of equally effective but less toxic combinations. While anti-CTLA-4/anti-PD-1 combinations involving different doses and schedules have shown improved toxicity profiles with comparable efficacy in extracranial melanoma metastases, these less toxic regimens have not been studied in patients with melanoma brain metastases. Similarly, promising novel anti-PD-1–based combinations have not yet been investigated in patients with melanoma brain metastases.
In addition, nivolumab + ipilimumab has shown much less activity in patients with symptomatic melanoma brain metastases, possibly related to the immunosuppressive effects of corticosteroids necessary to control the edema around the brain lesions. The development of alternatives to corticosteroids for controlling central nervous system edema, such as the VEGF antibody, bevacizumab, is urgently needed. Also, stereotactic radiosurgery has historically improved the outcomes of patients with melanoma brain metastases and may be synergistic with immunotherapy. Efforts to determine how best to combine these two effective treatment approaches remain a high priority.
Finally, leptomeningeal disease (LMD) remains a frequent site of treatment failure and source of death in patients with melanoma. Understanding whether LMD results from altered tumor biology or is an immune sanctuary is critical to developing effective treatment. Opportunities exist for intrathecal immunotherapy with checkpoint inhibitors alone and in combination, but such studies are complicated by the requirements for multidisciplinary involvement in the treatment, the relative rarity of the entity, patient comorbidities, and often rapid clinical decline. Lacking an effective treatment, studies to determine whether immunotherapy applied in earlier stages of melanoma can prevent the development of this devastating entity will be critical.
Recommended reading, ref. 51 .
Adjuvant therapy
Recent studies in adjuvant immunotherapy have changed the treatment landscape for patients with resected stage III or stage IV melanoma. Phase III studies have shown that adjuvant ipilimumab is superior to placebo in terms of OS, and that pembrolizumab is superior to placebo and nivolumab is superior to ipilimumab in terms of recurrence-free survival. More recently, a phase II trial found that adjuvant nivolumab + ipilimumab was superior to nivolumab alone in patients with stage IV resected melanoma in terms of recurrence-free survival. Results of a phase III trial of adjuvant nivolumab + ipilimumab versus nivolumab alone are awaited, but the initial release of information from an analysis of low PD-L1–expressing tumors showed no significant difference between the two arms in recurrence-free survival. Ongoing phase III trials are determining whether the benefits of nivolumab or pembrolizumab can be extended to patients with high-risk stage II disease. Also, the impact of these adjuvant immunotherapies on OS (essentially, the survival benefit of initiating treatment postoperatively vs. waiting and treating only those who relapse) is eagerly awaited.
Despite this progress, adjuvant treatment continues to present several unique challenges, including the unmet need for biomarkers to guide who require treatment, treatment duration, and intensity. Also, the management of recurrence after adjuvant immunotherapy is an issue of increasing importance since the numbers of relapsing patients will grow because of the expanded use of adjuvant therapy. Finally, there is a significant financial toxicity associated with these treatments that society will need to address to optimize access to such therapies.
Predictive biomarkers for improved patient selection
While several studies have highlighted candidate markers for selecting patients in need of further systemic therapy, more extensive prospective studies are necessary for rigorous validation using standard clinicopathologic prognostic factors as a comparator. In general, blood-based soluble biomarkers offer several advantages, including their amenability to longitudinal monitoring and their ability to sample heterogeneous tumors without the sampling bias intrinsic to tumor tissue–based assays. However, reported blood-based soluble markers, cfDNA, and plasma-derived tumor exosomes still require additional prospective validation in larger multicenter clinical trials. Ultimately, it appears likely that a combination of approaches will be required to identify patients most likely to benefit from adjuvant therapy. An improved understanding of underlying mechanisms of melanoma dormancy and reactivation could help guide further development of these markers (see above).
Prediction of immune-related adverse events
An area that is particularly relevant to adjuvant immunotherapy is the prediction of immune-related adverse events (irAE), because the acceptable risk to benefit ratio is much lower in this clinical setting. There is a pressing need for the development of biomarkers capable of identifying those patients who are at higher risk for severe life-threatening toxicities, such as myocarditis. There are emerging data implicating auto-antibodies, T-cell–derived cytokines, and neutrophil-related markers as predictive of specific irAEs in the metastatic disease setting; however, additional studies are needed to validate these data and verify that these are relevant to the adjuvant treatment setting. This process may be complicated by the diverse underlying pathogenesis of the various irAEs.
Duration of adjuvant treatment
While the current recommended adjuvant treatment duration is 1 year, additional studies are needed to more clearly define the most appropriate duration of adjuvant therapy for the individual patient to minimize both financial and clinical toxicity. It may be that much shorter durations will be equally effective with reduced costs and toxicity, especially for those with early stage III disease where the risk of melanoma relapse is greatly reduced.
Recommended readings, refs. 52–54 .
Neoadjuvant therapy
Traditionally, surgery has been a preferred option for patients with locoregionally advanced melanoma. However, the development of effective systemic therapies has led to clinical trials of immunotherapy or targeted therapy preceding surgery in resectable or borderline resectable patients. Recent studies showed that a single dose of anti-PD-1 produced a pathologic CR in 30% of treated patients, further supporting the idea that less than 1 year of adjuvant therapy might be sufficient. In other studies, two doses of combination anti-PD-1 + anti-CTLA-4 produced pathologic CRs in up to 60% of subjects, and pathologic CR was associated with freedom from subsequent relapse without need for postoperative adjuvant therapy. Recent studies examining whether this approach could be used to limit surgery to the resection of a marked “index” node rather than a complete node dissection show encouraging early results. Studies are also exploring whether information obtained from examination of that index lymph node could be used to guide subsequent treatment choices, such as additional surgery, continued adjuvant therapy, or switching to a different systemic therapy. On the basis of this work, one can foresee the role of surgery in patients with stage III and oligometastatic stage IV melanoma shifting from an attempt at definitive treatment to obtaining information about the interplay between tumor and the immune system that can guide upfront therapy, along with salvage resection of residual, nonresponding disease when necessary.
In addition, neoadjuvant therapy offers an unparalleled opportunity for interrogating tumor tissue to better define mechanisms of an effective immune response and the means of tumor escape for various therapies. As such, it is a great tool for the rational development of combination immunotherapy regimens. As early data suggest that a pathologic CR in a regional lymph node portends long-term disease-free survival, an opportunity exists for using this endpoint to identify promising combination regimens, potentially accelerating drug development by limiting the need for expensive large-scale randomized phase III trials. These advantages may be recognized by regulatory agencies and parallel the drug development pathways in other tumor types, such as breast cancer.
Recommended readings, refs. 55–58 .
Current challenges for melanoma clinical trials
Beyond basic scientific and clinical questions, there are other important clinical issues that have emerged from the success of therapy.
Financial toxicity
The financial toxicity (i.e., the personal impact of the cost of treatment borne directly by the patient) of modern melanoma therapy, especially immunotherapy, is related in large part to the duration of therapy and number of infusions administered. Currently, there is insufficient information on which to base the decision of when to stop therapy in the absence of toxicity or disease progression. Many questions exist: (i) can treatment be stopped after a radiologic CR? (ii) Is a radiologic CR a reliable measure of disease disappearance? (iii) Is it too restrictive or too insensitive? Clinical trials provide minimal data to shed light on these questions. Randomized trials assessing the optimal duration of therapy are difficult to perform as they require large numbers of patients to confirm equivalent treatment outcomes and have garnered little support from the pharmaceutical industry. In the absence of trial data, the incidence of late toxicity may influence the balance favoring more or less treatment. Furthermore, is there a better way to assess the presence of residual disease after treatment in those who display a PR or SD with standard imaging? The answer may provide further evidence for stopping treatment earlier. The potential approaches are numerous and include PET-CT scans with/without directed biopsies, blood markers (e.g., cfDNA and exosomes), and assays for circulating tumor cells. The development of various noninvasive techniques should be prioritized and potential funding sources should be identified and engaged.
Clinical trial accrual and completion
Critically important to our capacity to further improve outcomes for patients with melanoma is the need to limit the focus of clinical trials to the major hypotheses of the day, expedite the development of these trials in multicenter studies and cooperative groups and assess treatments in the neoadjuvant setting where pathologic response may provide more rapid evidence of clinical activity of a new agent or a new combination for early FDA approval. With the current broad spectrum of approved treatments for patients with melanoma, clinical research is often viewed as less of a necessity. However, it remains essential if we are to make further advances and better select patients for specific treatments. Therefore, it is even more critical than ever to identify means to incentivize referrals of patients for such clinical trials.
Raising the OS bar further
Biomarkers in melanoma are needed to select best regimens for specific cohorts of patients, based on a single or multiple biologic markers. For those unlikely to respond, we must define alternate or combination regimens ideally based on a better understanding of the underlying mechanism of immune resistance. These efforts require broad collaborations, harmonized definitions, and coordinated clinical trial portfolios that utilize all existing resources of cooperative groups, pharma and academic collaborations to accelerate the pace of progress for patients with melanoma.
Recommended readings, refs. 59, 60 .
Recommendations for future immunotherapy research are summarized in Table 4 .
Immunotherapy recommendations.
The COVID-19 pandemic created unprecedented obstacles and challenges in providing medical care to our patients with melanoma. We have been forced into making decisions about surveillance visits, surgery, and treatment that we have not considered previously, sometimes delaying patient care to protect patients, their families, and our staff from contracting COVID-19. This pandemic has forced us to adapt quickly and develop alternative strategies and solutions for patient management. This experience has raised many questions and provided us with few answers. We continue to learn and evolve our care practices every day so as to provide safe, seamless, and comprehensive care, despite the unknown duration of this new environment. This section details some of the changes that have been made and questions that merit future inquiry.
Screening for early melanoma
During the initial peak in the COVID-19 pandemic, nonessential workers were told to shelter in place. Most private dermatology offices were closed, so routine total body skin exams on established patients and evaluations of patients presenting with new suspicious lesions were delayed. A number of questions have now arisen as a result of these unanticipated delays: (i) what will be the impact of delayed diagnosis of a primary melanoma? (ii) Now that precautions are in place and dermatologists are back in the office, have we seen an increase in the stage at presentation of newly diagnosed patients? (iii) Will a 3- to 6-month delay in diagnosis or surveillance visits have a significant impact on disease stage at presentation or ultimate outcomes?
Telemedicine was thrust into the forefront due to this outbreak. It provided a platform for safely assessing and managing a patient's most acute issues. Patients could engage their care team via the internet and often providers could remotely visualize any skin lesions of concern to the patient. In some instances, a remote assessment could allay a patient's fears until an in-person visit could be scheduled. This technology does not, however, allow for routine screening to be performed and so potentially suspicious lesions not recognized by the patient are not routinely assessed. Furthermore, it remains unclear to what degree atypical skin lesions can be accurately assessed via telemedicine so as to prioritize in-person visits. The potential for AI or machine learning to assist in the virtual evaluation of skin lesions also remains unclear at this time. Other key questions regarding the value of virtual screening efforts include: (i) do we always need to see patients in person for follow-up visits when we are now proficient in telemedicine? (ii) Is telemedicine an option for our most at-risk populations as many elderly and underserved patients do not have smartphones, tablets, or computers in their home? (iii) How do we reach these patients remotely? (iv) Can we utilize mole image analysis applications to better evaluate pigmented skin lesions via telemedicine? (v) How do we validate, improve, and prioritize the various applications that are available or in development?
Surgery and neoadjuvant therapy
In many hospitals around the country, as COVID-19 numbers spiked, elective surgeries were delayed or cancelled, including cancer surgeries. Concerns about the availability of adequate personal protective equipment and the risks of transmission to hospital personnel, especially anesthesia providers, led to moratoria on surgeries even in areas where coronavirus infection rates were relatively low. Surgical committees were formed to help prioritize cases as operating rooms slowly reopened, with some patients waiting weeks or months for surgery on the basis of perceived relative urgencies.
The risks of surgical delays must be weighed against the risk of a patient acquiring COVID-19 infection in the hospital or early postoperative period, and against the impact of operating on a patient with occult or active COVID-19 infection. Available data suggest a substantial risk of mortality associated with general anesthesia during active COVID-19 infection and anecdotal evidence points to an increased risk of thrombotic events in the postoperative period as well. Thus, risks and benefits of delaying surgery must consider the prevalence of COVID-19 in a specific region, the availability of nonoperative or preoperative therapy, and the urgency of surgical intervention. Other factors impacting decision-making regarding cancer surgery are the nature of the procedure (general anesthesia vs. local/conscious sedation), the likelihood of requiring intensive care unit care, and the type of facility (general acute care hospital, free-standing cancer center, and ambulatory surgery facility not attached to a hospital).
The widespread delays in scheduling surgery for patients with melanoma forced many oncologists to consider neoadjuvant therapy for patients with advanced stage tumors (resectable clinical stage III and IV disease). However, this in turn led to the question of which neoadjuvant therapies could be safely administered without putting patients at increased risk of infections or other complications that would require them to seek urgent medical evaluation. Many patients did not want to leave their homes and most oncologists were concerned about administering immunotherapy without regular follow-up. A major concern surrounding neoadjuvant immunotherapy was the unknown and possible severe consequences of concomitant pneumonitis and COVID-19. Fortunately, additional studies have suggested that immunotherapy does not dramatically increase the risk for severe complications of COVID-19; thus, neoadjuvant immunotherapy may be considered as a valid option for patients, even in the COVID-19 era.
As a therapeutic modality without clear immunosuppressive or stimulatory properties, BRAF-targeted therapy was viewed as an acceptable alternative therapeutic approach even in the context of a concurrent COVID-19 infection (albeit in the absence of data). However, BRAF/MEK inhibitor therapy is known to induce pyrexia and chills, which would complicate triaging of patients when evaluating for possible symptomatic SARS CoV-2 infection. Specific BRAF/MEK inhibitor combinations have different rates of pyrexia and chills, so choosing a regimen with a low incidence of pyrexia, such as encorafenib plus binimetinib, made sense despite the absence of data for that combination in the neoadjuvant setting.
Another concern with neoadjuvant therapy is uncertainty about the duration of preoperative treatment required. Virtually all melanoma neoadjuvant therapy clinical trials reported to date have utilized a relatively short duration of preoperative systemic therapy with surgery scheduled soon after the last dose of drug. While some single-institution experience had suggested that 6 months or more of preoperative treatment was feasible and safe, there was substantial concern that such prolonged preoperative treatment might compromise resectability. Broader experience has now shown that this fear was overblown, and prolonged preoperative treatment can allow patients the flexibility to wait until surgery could be scheduled in a safe environment.
So, what have we learned and what questions remain? Although neoadjuvant therapy research is ongoing to assess its true value, this approach is likely here to stay. In the current era, we must carefully integrate the risks and benefits of surgery and neoadjuvant therapy against the risks of COVID-19 when making decisions about deferring surgery. Questions still remain about the best preoperative therapy for patients with BRAF-mutant melanoma, and what postoperative treatment should be delivered to patients who have a pathologic CR. But surgeons and oncologists should feel reassured that neoadjuvant therapy is a safe option and that preoperative treatment can continue for several months or longer if necessary.
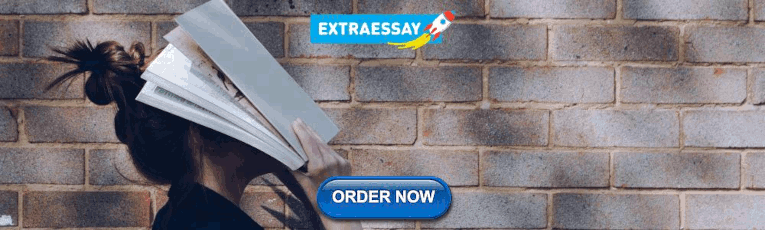
Adjuvant therapy and treatment for unresectable metastatic melanoma
Most oncologists advocate for starting adjuvant therapy for high-risk melanoma within 90 days of surgery based on the pivotal trial designs. However, during times of high COVID-19 prevalence, adjuvant therapy may be deferred beyond 90 days postsurgery to minimize the risk to the patient and to resource-challenged healthcare systems. The availability of longer interval regimens for nivolumab (4 weeks) and pembrolizumab (6 weeks) has eased some of these concerns for both patients contemplating and in the midst of adjuvant therapy. Also, arguments for use of BRAF-targeted therapy versus immunotherapy in patients with BRAF-mutant disease have been further accentuated by pandemic-related considerations of their distinct toxicity profiles and risks of hospital exposure. It is unknown how these delays in the initiation of therapy, changes in preferred treatment approaches, skipping doses of immunotherapy, or skipping adjuvant therapy altogether will impact patient outcomes.
Metastatic disease therapy
It is also unclear how the pandemic has altered the therapeutic management of patients with metastatic melanoma. Here, we list some pointed examples: (i) are patients being treated more frequently with anti-PD-1 monotherapy rather than in combination with anti-CTLA-4 due to concerns about the increased toxicity? (ii) Have oncologists migrated to longer intervals between anti-PD-1 treatments, such as the pembrolizumab every 6 weeks regimen? (iii) Are patients with BRAF-mutant melanoma treated more frequently with oral BRAF-targeted therapy to minimize visits to the cancer clinic or hospital infusion center? (iv) How has telemedicine been used to assess treatment-related toxicities and minimize visits to the clinic? (v) What other avenues are being used to minimize clinic visits, such as using local laboratories for blood draws instead of the hospital or clinic? (vi) Are patients being tested for COVID-19 before initiating therapy, and should asymptomatic patients be retested while on therapy? (vii) If patients test positive for COVID-19, how is a decision made to resume therapy? (viii) How long are oncologists and patients willing to wait to resume treatment? (ix) When offices are reopened, to what extent are patient volumes restricted compared with prepandemic, and how have waiting rooms been reconfigured to support social/physical distancing?
Clinical trials
COVID-19 has had a dramatic impact on clinical trial conduct. Given patients' decreased willingness to come to clinics for scheduled visits and risk exposure, some trials have granted waivers for certain requirements, such as biopsies and other procedures. Many trials have also permitted use of telemedicine visits or obtaining certain tests at facilities close to home instead of at the study site. For example, the NCI released a memorandum on guidance for their clinical trials affected by COVID-19, such as detailing what may constitute a minor deviation with regards to missed visits or biopsies ( https://ctep.cancer.gov/investigatorResources/corona_virus_guidance.htm ).
Increased allowance of telemedicine visits and off-site testing, if they remain an option in clinical trials in the post-COVID-19 era, may actually improve access and accrual to clinical trials. Many patients need to travel long distances to participate in clinical trials, which inevitably creates barriers to enrollment for patients who would otherwise be eligible and willing to participate. This can also lead to disparities in trial access, with patients who have the financial means and resources to take time off work and afford long travel are better able to enroll in trials. The pandemic experience may support the paring down or making optional tests that are not critical for the study conduct and other measures, such as enabling an electronic and remote consent processes, mailing oral study medications or study kits to patients, and utilizing closer off-site facilities for certain trial tests, exams, or treatments that may provide long-lasting benefit in clinical trial access for our patients.
Unanswered questions remain, including: (i) what will be the long-term impact of the pandemic on collection of research-related tumor biopsies and blood samples on clinical research? (ii) Will there be any long-term impact for patients infected previously with COVID-19 on their immune system and ability to respond to immune checkpoint inhibitors? (iii) Will suspension of clinical trials at many institutions hinder accrual momentum? (iv) Will data supporting major clinical breakthroughs be delayed or compromised substantially because of the pandemic? (v) How will NCI-designated cancer centers in hard-hit areas be impacted when they apply for renewal of their Cancer Center Support grant?
Biology/therapy
No vaccine for melanoma has been approved despite decades of research. The development of vaccines to SARS-CoV-2 has accelerated our knowledge of therapeutic vaccine development. COVID-19 vaccine development has enabled the comparison of vaccines developed by various approaches, such as CanSino (adenovirus AD5 vector), Oxford (adenovirus vector), Moderna and BioNTech/Pfizer (mRNA vector), Innovio (DNA electroporation), OncoSec (DNA electroporation + IL12), as well as via peptides (Novavax), for generation of humoral and T-cell responses directed against the SARS-CoV-2 spike protein. This knowledge may translate into the development of novel vaccines in melanoma and other cancers.
Cytokine release syndrome (CRS) can be a serious consequence of cytokine and checkpoint inhibitor immunotherapy for patients with melanoma and other cancers. CRS results in acute respiratory distress syndrome (ARDS) and occurs when the body mounts an extreme immune response, either because of enhanced activation by cancer immunotherapy or because of a superimposed viral infection. One of the key complications in patients during the current COVID-19 pandemic is a form of ARDS. Therefore, lessons learned previously in melanoma may inform treatment of COVID-19 and vice versa. Drugs targeted against cytokines or their production are currently in trials for both cancer and COVID-19. For example, catecholamines can increase cytokine (specifically IL6) production by signaling through the alpha-1 adrenergic receptor (a1-AR). By targeting various steps and molecules in this process, researchers hope to overcome the initial steps of CRS. Prazosin is an a1-AR antagonist used to treat hypertension. A retrospective analysis of non-COVID-19 patients with ARDS on prazosin demonstrated that these patients had lower rates of requiring ventilator support. Efforts to move this drug into clinical trials for COVID-19 are underway. Another drug, tocilizumab, which inhibits IL6 (one of the key cytokines involved in ARDS and which has been used to overcome CRS observed with CAR T therapy), is also under investigation in patients with COVID-19. So far, limited results demonstrating safety and efficacy of this drug in patients with COVID-19 have been published and several trials are underway, but off-protocol use of this drug has been widespread in some hospitals. Dexamethasone, commonly used for CRS-associated reactions, has proven to be an effective therapy for the inflammatory pneumonitis induced by COVID-19. Acalabrutinib, a BTK inhibitor, and ruxolitinib, a JAK/Stat inhibitor, have also shown the ability to reduce CRS, prompting clinical trials in patients with COVID-19 with some early promising results. Finally, mesenchymal stem cells, which can be derived from the bone marrow, umbilical cord, and placenta, are in clinical trials for the management of ARDS; however, their potency is unclear.
What have we learned? Just as we have applied lessons from treating CRS in patients receiving immunotherapy for melanoma and other cancers to managing complications in patients with COVID-19, we may learn from COVID-19 management how to better manage CRS in patients receiving cancer immunotherapy. We also hope to determine whether checkpoint blockade should be halted or discontinued in the event of acquiring a COVID-19 infection.
Psychosocial impact
COVID-19 has forced our patients to significantly curb the social interactions in their daily lives and has forced many patients into home confinement. This isolation has greatly increased their feelings of depression and hopelessness. If they do come into the oncology clinic, they are often not allowed to bring a visitor because of exposure risks. Having difficult discussions regarding disease progression or end-of-life issues with a patient who has no family or friends for support can cause extra psychologic trauma and confusion for the patient and is often heart-wrenching for the care team. In addition, faces, which are the most expressive part of our bodies, are now covered by masks for both patients and caregivers. There are no smiles to be shared. No hugs for comfort or encouragement. Often, there are no social workers physically present to be able to come speak with someone in need.
In these instances, telemedicine has emerged as a life-saving measure. Patients can connect virtually with either a social worker or psychiatrist and meet face to face without masks and in the privacy of their own homes. They are able to make a personal connection, albeit virtually. A comprehensive review of telemedicine and quality of life in patients with cancer demonstrated that a virtual connection was just as effective as an in-person visit on cancer patient's quality of life. But the most horrible impact of COVID-19 may well be on the terminally ill, including those with non-COVID-19 illnesses, such as advanced melanoma, in that they often die alone. Dying with your loved ones only allowed on a video screen is a previously unthinkable scenario and causes unfathomable grief.
Finally, the psychosocial impact of the pandemic on healthcare workers cannot be overlooked. Many physicians, including medical oncologists, have been pulled from regular duties and assigned clinical responsibilities in COVID-19 wards. By all accounts, the impact of dealing with this pandemic has been a huge psychologic drain, and often physicians and nurses were the ones holding the aforementioned video screens or being the conduit of daily information from the patient to their family or friends. In addition, physicians and other healthcare workers tried to minimize and manage the risks posed to themselves and their families, and some watched in horror as their own colleagues succumbed to this disease. Younger physician-scientists also appropriately worry about the impact to their careers. Helping our colleagues to find avenues to regain lost time and funding in the research realm is imperative. Finally, acknowledging the stress and anxiety this pandemic has had on the melanoma community, both patients and their healthcare team, is a first step in trying to achieve a critical balance of providing care while keeping safety an utmost priority.
Recommended readings, refs. 61–65 .
Key areas for future study as a consequence of the COVID-19 pandemic, Table 5 .
Key areas for future study as a consequence of the COVID-19 pandemic.
Our understanding of the biology and our success in managing melanoma have progressed greatly over the past half decade, with as many as 50% of patients with stage IV disease being anticipated to be long-term survivors and up to half of those patients with high-risk stage III disease who were once destined to progress to stage IV disease being prevented from disease relapse. Simultaneous progress has been made in primary and secondary prevention with new technologies for melanoma detection and new policies for sun and UV light protection. This progress has been manifest by the remarkable overall decline in annual mortality in the United States from melanoma.
However, such progress has created new disease states (e.g., immunotherapy- and targeted therapy–resistant disease), raised new questions, and exposed new challenges and opportunities for further research to continue this progress. Furthermore, the recent pandemic has created new challenges to the dermatologist and medical and surgical oncologist alike, while it has also accelerated the development of novel technologies for melanoma detection and the relaxation of clinical trial procedures that may pay dividends in broadening the reach of melanoma research. Yet at the same time, the pandemic is putting further strains on both patients and caregivers and the careers of young investigators entering this field.
This updated Melanoma Research Foundation–sponsored State of the Science report highlights the many new questions, challenges, and opportunities across the melanoma spectrum. We anticipate that over the next 5 years significant advances will be made in: noninvasive imaging complemented by AI, our understanding of clinical dormancy and how to exploit it for better patient outcomes, developing rationally based combinations of immune- and nonimmune-based drugs and more sophisticated biomarkers to help determine the optimal combination and sequence for individual patients, the development of more representative animal models of melanoma that will better inform clinical trial designs, and learning how to combat metastatic melanoma at challenging sites, such as the brain and leptomeninges. In this report we offer a roadmap for how research might proceed to sustain progress and ultimately reach our goal of preventing mortality and limiting morbidity from melanoma.
M.B. Atkins reports grants and personal fees from BMS, Merck, and Pfizer, personal fees from Novartis, Genentech/Roche, personal fees from Eisai, Exelixis, AstraZeneca, Agenus, Adagene, Aveo, Array, Pyxis Oncology, Leads BioPharma, Werewolf, Elpis, TRV, PACT, Iovance, Idera, and Apexigen, personal fees and other from Arrowhead, Neoleukin, and Immunocore outside the submitted work, and stock options from Werewolf and Pyxis Oncology. C. Curiel-Lewandrowski reports personal fees from BMS and Helssin's Pharm outside the submitted work. D.E. Fisher reports personal fees from Soltego and grants from NIH during the conduct of the study, as well as a patent for small-molecule regulators of pigmentation pending and licensed to Soltego Inc. H. Tsao reports personal fees from Epiphany Dermatology, Ortho Dermatologics, and Up-to Date outside the submitted work. J.A. Aguirre-Ghiso is a scientific cofounder of, scientific advisory board member, and equity owner in HiberCell and receives financial compensation as a consultant for HiberCell, a Mount Sinai spin-off company focused on the research and development of therapeutics that prevent or delay the recurrence of cancer. M.S. Soengas reports other from Bioncotech Therapeutics outside the submitted work. A.T. Weeraratna reports grants from NCI during the conduct of the study. K.T. Flaherty reports personal fees from Clovis Oncology, Strata Oncology, Checkmate Pharmaceuticals, Kinnate, Scorpion, PIC Therapeutics, Apricity, Tvardi, xCures, Monopteros, Vibliome, ALX Oncology, Lilly, Takeda, Boston Biomedical, and X4 Pharmaceuticals and grants and personal fees from Novartis during the conduct of the study. J.A. Sosman reports personal fees from Array, Bristol Myers Squibb, Genentech, Iovance, and Apexigen, and grants from Bristol Myers Squibb outside the submitted work. H.A. Tawbi reports grants and personal fees from BMS, Merck, Novartis, Genentech, grants from GlaxoSmithKline, and personal fees from Iovance and Eisai outside the submitted work. A.C. Pavlick reports personal fees from BMS and Regeneron outside the submitted work. S. Chandra reports other from Bristol Myers Squibb, Novartis, Regeneron/Sanofi, Pfizer, EMD Serono, and Exicure outside the submitted work. P.B. Chapman reports personal fees from Merck and Pfizer during the conduct of the study. A. Daud reports grants from Merck, BMS, OncoSec, Incyte, Xencor, and Novartis, personal fees from Pfizer, Trex, and Neuvogen, and grants and personal fees from Genentech/Roche outside the submitted work. Z. Eroglu reports personal fees from Array, grants and personal fees from Novartis, personal fees from Genentech, Regeneron, Sun Pharma, and Natera outside the submitted work. L.K. Ferris reports personal fees from Derm Tech outside the submitted work. B.A. Fox reports personal fees and nonfinancial support from AstraZeneca, personal fees from Boehringer Ingelheim, CellDex Therapeutics, Definiens, PrimeVax, and Ultivue, grants and nonfinancial support from Bristol Myers Squibb, OncoSec, and Shimadzu, grants from Incyte, Janssen/Johnson & Johnson, and MacroGenics, and nonfinancial support from PerkinElmer/Akoya and NanoString outside the submitted work, as well as a patent for cancer vaccine technology issued and licensed to UbiVac. J.E. Gershenwald reports personal fees from Merck, Bristol Myers Squibb, Novartis, Regeneron, and Syndax outside the submitted work. G.T. Gibney reports personal fees from Novartis, Regeneron, Bristol Myers Squibb, and Merck and other from Exelixis outside the submitted work. D. Grossman reports personal fees from Orlucent and other from DermTech outside the submitted work. B.A. Hanks reports grants and personal fees from Merck, grants from Tempest Therapeutics, D3 A*STAR Singapore, Leap Therapeutics, OncoMed Therapeutics, Seagen Inc., Sanofi, and Exicure Inc., personal fees from Novartis, G1 Therapeutics outside the submitted work, as well as a patent for composition of matter and methods for alteration of dendritic cell metabolism to augment cancer vaccine efficacy issued to B.A. Hanks, predictive biomarkers for cancer immunotherapy and methods of using same pending to B.A. Hanks, and compositions and methods for increasing the efficacy of anti-PD-1 antibody immunotherapy pending to B.A. Hanks. J.M. Jeter reports grants from Bristol Myers Squibb and Merck and personal fees from Array Biopharma outside the submitted work. D.B. Johnson reports grants and other from BMS and other from Catalyst, Iovance, Jansen, Merck, Novartis, and OncoSec outside the submitted work. S.N. Khleif reports grants from AstraZeneca, Bristol Myers Squibb, Heat Bio/Pelican Therapeutics, Lycera, MedImmune, Merck/EMD Serono, and Syndax during the conduct of the study, personal fees from Advaxis, Amoon, Adaptive Biotechnology, Aummune, CanImGuide Therapeutics, and Coherus, grants and personal fees from BioLine Therapeutics, IO Biotechnologies, and KAHR Medical, nonfinancial support from Cytomex, personal fees from Incyte, McKinsey Health, Nectin TX, Northwest Biotherapeutics, PDS Biotechnology, Tessa Therapeutics, and UbiVac outside the submitted work, and is founder/president/CEO/employee of Georgiamune, LLC- Biotechnology Company. J.M. Kirkwood reports grants and personal fees from Amgen, BMS, Checkmate Pharmaceuticals, Iovance, and Novartis Pharmaceuticals, personal fees from Harbour BioMed, Istari Oncology, OncoSec, Scopus BioPharma, and Pfizer, grants from Castle Biosciences and Immunocore LLC outside the submitted work. V.K. Sondak reports personal fees from Merck, BMS, Eisai, Novartis, and Regeneron outside the submitted work. R.J. Sullivan reports personal fees from Array Biopharma, Asana Biosciences, Eisai, Iovance, AstraZeneca, Pfizer, Novartis, Replimune, Syndax, OncoSec, and Compugen, grants and personal fees from Amgen and Merck outside the submitted work. No disclosures were reported by the other authors.
The Editor-in-Chief of Clinical Cancer Research is an author on this article. In keeping with AACR editorial policy, a senior member of the Clinical Cancer Research editorial team managed the consideration process for this submission and independently rendered the final decision concerning acceptability.
The authors are indebted to Dr. Robyn Burns, Science Officer for the Melanoma Research Foundation, for her expert assistance with the preparation of this article. Research reported in this publication was supported, in part, by the NIH SPORE grants (1P50CA221703 to J.E. Gershenwald and P50CA225450 to E. Hernando), DoD/CDMRP Team Science Award (CA170374 to J.E. Gershenwald), the Robert and Lynne Grossman Family Foundation (to J.E. Gershenwald), the Michael and Patricia Booker Melanoma Research Endowment (to J.E. Gershenwald), philanthropic contributions to the Melanoma Moon Shots Program of MD Anderson (to J.E. Gershenwald), NIH intramural research program (ZIABC008756 to G. Merlino), the InterMEL P01 (P01CA206980 to E. Hernando), and the Melanoma Research Foundation.
Citing articles via
Email alerts.
- Online First
- Collections
- Online ISSN 1557-3265
- Print ISSN 1078-0432
AACR Journals
- Blood Cancer Discovery
- Cancer Discovery
- Cancer Epidemiology, Biomarkers & Prevention
- Cancer Immunology Research
- Cancer Prevention Research
- Cancer Research
- Cancer Research Communications
- Clinical Cancer Research
- Molecular Cancer Research
- Molecular Cancer Therapeutics
- Info for Advertisers
- Information for Institutions/Librarians

- Privacy Policy
- Copyright © 2023 by the American Association for Cancer Research.
This Feature Is Available To Subscribers Only
Sign In or Create an Account
Skip to Content
- Conquer Cancer
- ASCO Journals
- f Cancer.net on Facebook
- t Cancer.net on Twitter
- q Cancer.net on YouTube
- g Cancer.net on Google
Types of Cancer
- Navigating Cancer Care
- Coping With Cancer
- Research and Advocacy
- Survivorship
Melanoma: Latest Research
ON THIS PAGE: You will read about the scientific research being done to learn more about melanoma and how to treat it. Use the menu to see other pages.
Doctors are working to learn more about melanoma, ways to prevent it, how to best treat it, and how to provide the best care to people diagnosed with this disease. The following areas of research may include new options for patients through clinical trials. This section is not meant to be a complete list of new clinical trials because this field is changing rapidly. Always talk with your doctor about the best diagnostic and treatment options for you.
Enhanced prevention and early detection methods. There is ongoing research on better prevention and early detection strategies for melanoma. Both primary and secondary prevention are important. Primary prevention involves keeping melanoma from developing, such as reducing exposure of ultraviolet (UV) radiation from the sun and avoiding the use of indoor tanning in any form. Secondary prevention includes methods of early detection. One promising area is the screening of people with a high risk of developing melanoma, as described earlier in this guide .
Targeted therapy. As discussed in Types of Treatment , targeted therapy is a treatment that targets specific genes or proteins. Research has identified a number of molecular pathways and activated or mutated genes in melanoma. Clinical trials are testing new drugs to inhibit the MAP kinase pathway and other pathways that melanoma might use to grow and spread. Strategies to prevent the melanoma from becoming resistant to treatment are also being tested, such as using combinations of drugs or exploring new schedules of giving drugs to patients.
Immunotherapy. As explained in Types of Treatment , immunotherapy is an important treatment option for melanoma. Researchers are studying new checkpoint inhibitors and immunotherapies directed at other parts of the immune system. These include TIM3 inhibitors, LAG3 inhibitors, OX40 agonists, CD137 agonists, GITR agonists, and IDO inhibitors. Also, a number of combinations of immunotherapies are being looked at, including all of the above drugs with PD-1 or PD-L1 inhibitors, as well as combinations of targeted therapies with PD-1 or PD-L1 inhibitors. Recently, the combination of a LAG3 inhibitor, called relatlimab, and nivolumab was shown to delay tumor growth when compared with nivolumab alone in people with newly diagnosed, unresectable stage III or metastatic melanoma. A fixed-dose combination of these 2 drugs, called Opdualag, has been approved to treat unresectable stage III or stage IV melanoma in people aged 12 and older. It is considered an alternative standard of care treatment to using a single PD-1 inhibitor and to using a combination of ipilimumab and nivolumab.
Adoptive cell therapy (ACT), chimeric antigen receptor T-cell (CAR-T) therapy, and T-cell receptor (TCR) therapy. Another type of experimental immunotherapy involves altering a person’s white blood cells, known as lymphocytes, in a laboratory. In ACT, this may involve a surgical procedure to remove a tumor, isolate the white blood cells, and expand them outside the body. In CAR-T therapy and TCR therapy, white blood cells are removed from the body using a procedure called pheresis. The white blood cells are modified to better recognize cancer cells. This is done to increase their ability to fight the tumor. Then, the cells are given back to the patient, often in combination with chemotherapy, interleukin-2, and/or other immunotherapies.
Vaccines. Therapeutic vaccines that may improve the specific immune response to melanoma have been the focus of multiple clinical trials. Melanoma peptide vaccines are being evaluated in clinical trials for patients with both localized and advanced melanoma. Research has shown that vaccination can cause the immune system to fight melanoma, even in advanced disease, but these treatments are still considered experimental. The vaccines are made using certain proteins found only on a melanoma tumor and are given as an injection. The person’s immune system then recognizes the proteins and destroys melanoma cancer cells. To date, no vaccines have shown a clinical benefit in patients. Learn more about the basics of cancer vaccines .
Palliative and supportive care. Clinical trials are underway to find better ways of reducing symptoms and side effects of current melanoma treatments to improve comfort and quality of life for patients.
Looking for More About the Latest Research?
If you would like more information about the latest areas of research in melanoma, explore these related items that take you outside of this guide:
To find clinical trials specific to your diagnosis, talk with your doctor or search online clinical trial databases .
Visit the Cancer.Net Blog to review news and information about melanoma, including research announced at recent scientific meetings or in ASCO’s peer-reviewed journals, including highlighted studies from 2022 and 2021 .
Listen to a podcast from an ASCO expert in melanoma discussing highlights from the 2021 ASCO Annual Meeting.
Get updates from Cancer.Net delivered right to your inbox. Subscribe to the Inside Cancer.Net email newsletter.
Visit the website of Conquer Cancer, the ASCO Foundation , to find out how to help support cancer research. Please note that this link takes you to a different ASCO website.
The next section in this guide is Coping with Treatment . It offers some guidance on how to cope with the physical, emotional, social, and financial changes that cancer and its treatment can bring. Use the menu to choose a different section to read in this guide.
Melanoma Guide
Cancer.Net Guide Melanoma
- Introduction
- Medical Illustrations
- Risk Factors and Prevention
- Symptoms and Signs
- Types of Treatment
- About Clinical Trials
- Latest Research
- Coping with Treatment
- Follow-Up Care
- Questions to Ask the Health Care Team
- Additional Resources
View All Pages
Timely. Trusted. Compassionate.
Comprehensive information for people with cancer, families, and caregivers, from the American Society of Clinical Oncology (ASCO), the voice of the world's oncology professionals.
Find a Cancer Doctor

Melanomas resist drugs by 'breaking' genes
Genomic deletions explain why some types of melanoma resist targeted therapies.
Melanoma is the deadliest form of skin cancer. With global incidence rates rising, new, more effective treatments are necessary to alleviate the health burden of the disease. Important advances in recent years include doctors using genetic tests to look for specific mutations they can target for more personalised, effective treatment.
Around 1 in 2 melanoma patients will have mutations in the BRAF gene. This gene normally makes a protein which helps control cell growth, but mutations can cause the cells to grow and divide uncontrollably instead, happening in many different types of cancer including melanoma.
The discovery of BRAF mutations has led to development of targeted therapies to inhibit its function. One of the standard treatment options for melanoma over the last ten years has been to simultaneously target both BRAF mutations and MEK. These two genes are part of the MAPK signalling pathway, which, in cancer, is rewired for uncontrolled growth. Targeting two different critical points in the same domino chain helps slow or stop cancer growth.
Despite great initial responses to the combined use of the first-generation inhibitors, around 50% of melanoma patients with BRAF mutations will relapse within one year. The cancer acquires resistance to the drugs, finding other ways to reactive the MAPK pathway through mechanisms which remain poorly understood.
"Melanoma drug resistance is a huge clinical problem because it occurs in almost all BRAF-mutated patients under BRAF/MEK inhibitor therapy and there are few or no therapeutic alternatives. There is an urgent need to understand the many different underlying mechanisms and find new strategies to deal with this constantly evolving arms race," says Dr. Francisco Aya Moreno, a medically-trained oncologist and recent PhD graduate at the Centre for Genomic Regulation (CRG) in Barcelona.
A study published today in the journal Cell Reports has disentangled the mechanisms behind one of the ways cancer cells develop resistance to targeted therapy. The study found that, in response to treatment, melanomas can 'break' parts of their BRAF gene, also known as genomic deletions. This helps the tumour create alternative versions of the protein (altBRAFs) which lack regions targeted by BRAF inhibitors, reactivating the MAPK pathway and making the drugs less effective. The finding was consistent across various lab models and patient tumour samples.
The findings are important because altBRAFs were thought to be made through alternative splicing, which is when cells use the same gene to synthesise different proteins. The discovery that genomic deletions, and not splicing, are the cause means a shift away from previous proposals for using drugs that target splicing as a therapeutic strategy.
"For years, we've known that some patients produce altBRAFs and these help the cancer resist treatment, but we misunderstood the mechanism behind their creation. Knowing that genomic deletions are the cause opens new avenues for developing therapies that could more effectively help patients with BRAF mutations," explains ICREA Research Professor Juan Valcarcel, co-author of the study and researcher at the Centre for Genomic Regulation.
Surprisingly, the researchers found evidence of the same genomic deletions in melanomas which hadn't been treated yet. In other words, melanomas can naturally develop mechanisms that mimic drug resistance, even without exposure to drugs. Identifying and targeting these early resistance mechanisms through profound genetic testing in a clinical setting before treatment begins could improve the efficacy of first-line therapies.
Even more surprisingly, further analyses revealed that genomic deletions might be a more widespread mechanism of oncogenesis and resistance than previously thought. Though uncommon, researchers found evidence of altBRAFs in melanomas with a normal-functioning BRAF gene, as well as in other types of cancer including non-small cell lung cancer, breast cancer, kidney cancer and prostate cancer. The findings could increase the patient population benefiting from targeted treatments which are currently under clinical development.
"There is an emerging class of drugs known as second generation RAF inibitors. Unlike BRAF inhibitors, these drugs have a broad spectrum, and so could potentially inhibit the function of altBRAFs. Clinical trials which are assessing their effectiveness should also expand to include melanoma patients with a normal functioning BRAF gene as well, and possibly to other cancer types which express altBRAFs," explains Dr. Aya Moreno.
Dr. Aya Moreno is part of the second cohort of the PhD4MD programme, a joint effort by Centre for Genomic Regulation (CRG), the Institute for Research in Biomedicine (IRB Barcelona), the August Pi i Sunyer Biomedical Research Institute (IDIBAPS) and the Vall d'Hebron Research Institute (VHIR) designed to leverage the medical insight of a doctor to drive research that benefits patients.
"Having the opportunity to approach this research with both a clinician's perspective and a scientist's curiosity has been invaluable. It allowed us to uncover not just how melanomas resist treatment but also how this knowledge could lead to more effective therapies for patients. This fusion of clinical insight and scientific investigation is crucial for making real progress in our fight against cancer," concludes Dr. Aya Moreno.
The study was led by Dr. Aya Moreno and co-supervised by Professor Juan Valcarcel at the Centre for Genomic Regulation and Dr. Ana Arance at IDIBAPS. It was also carried out in collaboration with Nuria López Bigas' research group at IRB Barcelona. Since completing his PhD at the CRG, Dr. Aya Moreno has returned to the Medical Oncology department in the Hospital Clinic in Barcelona.
- Personalized Medicine
- Skin Cancer
- Lung Cancer
- Diseases and Conditions
- Breast Cancer
- Colon Cancer
- Malignant melanoma
- Chemotherapy
- Monoclonal antibody therapy
- Breast cancer
- Antiretroviral drug
Story Source:
Materials provided by Center for Genomic Regulation . Note: Content may be edited for style and length.
Journal Reference :
- Francisco Aya, Pablo Lanuza-Gracia, Abel González-Pérez, Sophie Bonnal, Estefania Mancini, Nuria López-Bigas, Ana Arance, Juan Valcárcel. Genomic deletions explain the generation of alternative BRAF isoforms conferring resistance to MAPK inhibitors in melanoma . Cell Reports , 2024; 114048 DOI: 10.1016/j.celrep.2024.114048
Cite This Page :
Explore More
- How 3D Printers Can Give Robots a Soft Touch
- Combo of Multiple Health Stressors Harming Bees
- Methane Emission On a Cold Brown Dwarf
- Remarkable Memories of Mountain Chickadees
- Predicting Future Marine Extinctions
- Drain On Economy Due to Climate Change
- 'Tube Map' Around Planets and Moons
- 'Bizarre' Evolutionary Pattern: Homo Lineage
- Largest Known Marine Reptile
- Neolithic Humans Lived in Lava Tube Caves
Trending Topics
Strange & offbeat.
Thank you for visiting nature.com. You are using a browser version with limited support for CSS. To obtain the best experience, we recommend you use a more up to date browser (or turn off compatibility mode in Internet Explorer). In the meantime, to ensure continued support, we are displaying the site without styles and JavaScript.
- View all journals
- My Account Login
- Explore content
- About the journal
- Publish with us
- Sign up for alerts
- Open access
- Published: 16 April 2024
Uveal melanoma immunogenomics predict immunotherapy resistance and susceptibility
- Shravan Leonard-Murali 1 , 2 , 3 , 4 ,
- Chetana Bhaskarla ORCID: orcid.org/0009-0009-6588-8083 1 , 2 , 3 ,
- Ghanshyam S. Yadav 1 , 2 , 3 ,
- Sudeep K. Maurya 1 , 2 , 3 ,
- Chenna R. Galiveti 1 , 2 , 3 ,
- Joshua A. Tobin 1 , 2 , 3 ,
- Rachel J. Kann 1 ,
- Eishan Ashwat 1 ,
- Patrick S. Murphy 1 , 2 ,
- Anish B. Chakka ORCID: orcid.org/0000-0002-1095-1817 5 ,
- Vishal Soman 5 ,
- Paul G. Cantalupo 5 ,
- Xinming Zhuo 6 ,
- Gopi Vyas 6 ,
- Dara L. Kozak 6 ,
- Lindsey M. Kelly 6 ,
- Ed Smith ORCID: orcid.org/0009-0000-6024-5227 6 ,
- Uma R. Chandran 1 , 5 ,
- Yen-Michael S. Hsu 1 , 7 , 8 &
- Udai S. Kammula ORCID: orcid.org/0000-0001-6894-4356 1 , 2 , 3
Nature Communications volume 15 , Article number: 2863 ( 2024 ) Cite this article
514 Accesses
62 Altmetric
Metrics details
- Cancer genomics
- Surgical oncology
- Tumour biomarkers
- Tumour immunology
Immune checkpoint inhibition has shown success in treating metastatic cutaneous melanoma but has limited efficacy against metastatic uveal melanoma, a rare variant arising from the immune privileged eye. To better understand this resistance, we comprehensively profile 100 human uveal melanoma metastases using clinicogenomics, transcriptomics, and tumor infiltrating lymphocyte potency assessment. We find that over half of these metastases harbor tumor infiltrating lymphocytes with potent autologous tumor specificity, despite low mutational burden and resistance to prior immunotherapies. However, we observe strikingly low intratumoral T cell receptor clonality within the tumor microenvironment even after prior immunotherapies. To harness these quiescent tumor infiltrating lymphocytes, we develop a transcriptomic biomarker to enable in vivo identification and ex vivo liberation to counter their growth suppression. Finally, we demonstrate that adoptive transfer of these transcriptomically selected tumor infiltrating lymphocytes can promote tumor immunity in patients with metastatic uveal melanoma when other immunotherapies are incapable.
Similar content being viewed by others
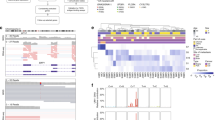
Molecular profiling of driver events in metastatic uveal melanoma
Joakim Karlsson, Lisa M. Nilsson, … Jonas A. Nilsson
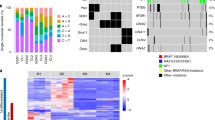
Multimodel preclinical platform predicts clinical response of melanoma to immunotherapy
Eva Pérez-Guijarro, Howard H. Yang, … Glenn Merlino
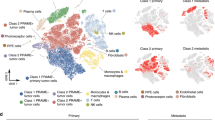
Single-cell analysis reveals new evolutionary complexity in uveal melanoma
Michael A. Durante, Daniel A. Rodriguez, … J. William Harbour
Introduction
Significant advances have been made in the treatment of metastatic cutaneous melanoma (CM) using immune checkpoint inhibition (ICI) targeting the cytotoxic T-lymphocyte-associated protein 4 (CTLA-4), the programmed cell death protein 1 (PD-1), and the lymphocyte-activation gene 3 protein (LAG-3) 1 , 2 , 3 , 4 , 5 . Unfortunately, ICI therapy has not shown comparable activity against most other solid tumors, especially those with low tumor mutational burden (TMB) 3 , 6 . To improve immunotherapeutic strategies for this large group of unresponsive cancers we focused our studies on uveal melanoma (UM), a prototypic ICI resistant cancer with low TMB 4 , 7 , 8 , 9 , 10 . With an annual incidence of ~6 per million in Europe and the United States, UM is a rare cancer accounting for 3% of all melanomas 9 , 10 . Although both CM and UM develop from transformed melanocytes, UM uniquely arises from the pigmented epithelium of the uveal tract, an immune privileged site 11 , and has an unusual predilection to aggressively metastasize to the liver which results in a dismal prognosis 9 . In further distinction, immunotherapies demonstrating efficacy against metastatic CM have shown disappointing results against UM 7 , 8 , leading to speculation that UM is an immunologically ‘cold’ variant of melanoma. However, there has been recent therapeutic progress with the clinical introduction of tebentafusp, a bispecific glycoprotein 100 peptide-HLA-directed CD3 T cell engager, which has intriguingly improved overall survival in patients with metastatic UM yet has demonstrated only limited ability to mediate tumor regression 12 , 13 . To reconcile these paradoxical findings and develop more effective immunotherapeutics for metastatic UM, we sought to build upon our previous discovery that a subset of UM metastases naturally harbor tumor infiltrating lymphocytes (TIL) with potent autologous anti-tumor reactivity 14 and that adoptive cell therapy (ACT) administering such TIL could mediate cancer regression in 35% of patients with metastatic UM, including individuals who were refractory to ICI 15 . These observations suggested that occult immune responses exist and can be exploited to treat metastatic UM.
In this work we perform comprehensive immunogenomic profiling on the largest and most diverse group of human UM metastases compiled to date to uncover the tumor microenvironmental properties that underlie its occult immunogenicity and promote its resistance and susceptibility to different classes of immunotherapy. We find that over half of these metastases harbor TIL with potent autologous tumor specificity, despite having low tumor mutational burden and resistance to prior immunotherapies, including ICI and the bispecific T cell engager tebentafusp. These T cell infiltrated metastases display activated antigen presenting cells, chronic interferon signaling, and diverse T cell receptor repertoires. However, we observe strikingly low intratumoral T cell receptor clonality and transcriptionally non-proliferative TIL within the tumor microenvironment even after ICI and tebentafusp therapy, demonstrating that these immunotherapies were insufficient to induce proliferation of the tumor reactive TIL. To harness the therapeutic potential of these quiescent TIL, we develop rapid tumor transcriptomic profiling to enable their selective in vivo identification and ex vivo liberation to counter their growth suppression. We demonstrate that adoptive transfer of these transcriptomic selected TIL can promote tumor immunity in patients with metastatic UM when other immunotherapies are incapable.
Clinicogenomic landscape of metastatic uveal melanoma
One hundred metastases were surgically procured from 84 UM patients as part of eligibility screening for TIL ACT clinical trials at the National Cancer Institute and the University of Pittsburgh Medical Center between 2013 and 2022 (NCT01814046 and NCT03467516) 15 , 16 . Resected metastases originated from 11 unique anatomic locations (Fig. 1a ), with liver as the predominant procurement site (56%) (Fig. 1b ). Patient demographics revealed a median age of 56 years (range = 17–78) and an even gender distribution (52% female, 48% male) (Supplementary Data 1 ). Patients had extensive metastatic disease burdens, with 95% having liver involvement, 75% having elevated LDH levels, and 71% with M1B or M1C stage (AJCC 8th edition) (Fig. 1c ). Metastases were harvested from both treatment naïve patients (24%) and treatment refractory patients (76%). Notably, 46 patients received prior ICI therapy (anti-CTLA4 only = 3, anti-PD-1 only = 11, sequential therapy = 8, combination therapy = 24) and 9 patients received tebentafusp, of whom none showed objective response (Fig. 1c ). Somatic mutational analysis of the metastases confirmed a low TMB (median = 0.64 mutations per megabase) with ubiquitous and mutually exclusive presence of established UM driver mutations ( GNAQ , GNA11 , CYSLTR2 or PLCB4 ) and frequent secondary alterations of BAP1 (62%) and SF3B1 (42%) (Fig. 1c and Supplementary Fig. 1a–e ). Somatic copy number alterations included chromosome 3 loss (46%) and 8q gain (85%) (Fig. 1c ). No associations were found between TMB and cohort demographics (Supplementary Fig. 1f and Supplementary Data 2 ). In sum, clinicogenomic profiling established this patient cohort to be broadly representative of advanced UM and the procured metastases to have canonical UM driver alterations and low TMB.
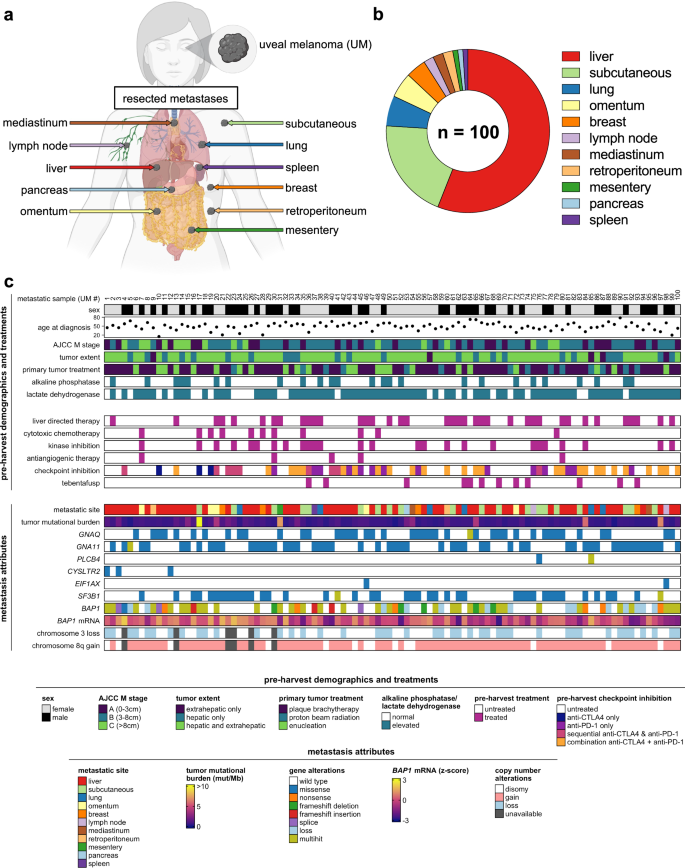
a Diversity of source tissues of resected metastases. Created with BioRender.com. b Distribution of source tissues of resected metastases. c Clinicogenomic annotation of individual metastases. Each column represents a single metastasis. BAP1 mRNA z -scores were calculated using log 2 (normalized counts).
Unbiased tumor transcriptomics reveals T cell-inflamed uveal melanoma metastases
Current tumor biomarkers for immunotherapy susceptibility, such as TMB and PD-L1, are rarely used in metastatic UM due to the uniformly low expression of these markers in this melanoma variant 3 , 4 , 17 . Thus, we first sought to discover alternative immune prognostic metrics by interrogating the transcriptome of UM metastases using total RNA sequencing and unbiased computational profiling. Further, to facilitate a clinically relevant and minimally invasive biopsy approach for in situ tumor characterization, we restricted our analysis to a single random biopsy from each resected metastasis (~2 mm central core fragment from 93 metastases and ~500,000 cells post tumor dissociation from 7 metastases). Principal component analysis (PCA) revealed the majority of transcriptional variance among the metastases was restricted to PCs 1, 2, and 3 (variance contributed: 19%, 13%, 12% respectively) while the remaining PCs (4–100) each contributed ~5% or less variance (Supplementary Fig. 2a ). Metastases were then mapped according to the three main PC coordinates (PCs 1, 2, and 3) (Supplementary Fig. 2b, c ). To determine whether specific cellular pathways and processes were associated with specific PCs, we correlated PC coordinates (1, 2, and 3) with enrichment scores for each of the canonical hallmark gene sets from the Human Molecular Signatures Database (Supplementary Data 3 ) 18 . Unsupervised clustering (Euclidean distance) of the PC-gene set correlations (Spearman’s rho) identified 4 discrete clusters (A, B, C, and D) with unique biologic motifs (Fig. 2a ). Cluster A included cellular metabolism pathways ( MYC TARGETS V1, MTORC1 SIGNALING, OXIDATIVE PHOSPHORYLATION ), Cluster B included immune and inflammatory signaling pathways ( INTERFERON ALPHA RESPONSE, INTERFERON GAMMA RESPONSE, ALLOGRAFT REJECTION, IL2 STAT5 SIGNALING ), Cluster C included liver dominant physiologic pathways ( BILE ACID METABOLISM, COAGULATION, CHOLESTEROL HOMEOSTASIS ), and Cluster D included cellular signaling and division ( WNT BETA CATENIN SIGNALING, MYC TARGETS V2, G2M CHECKPOINT ). The individual metastatic samples were further clustered by their relative expression of each of the hallmark gene set clusters to reveal striking variability across the tumor cohort (Fig. 2b ). Having identified transcriptomic differences among the metastases, we next sought to determine whether any of the three PCs independently correlated with the expression of the gene set clusters. Average Spearman’s rank correlation coefficients (rho) for each of the gene set cluster enrichment scores (A, B, C, and D) were mapped against the individual PCs (1, 2, and 3) (Fig. 2c ). We observed that cluster A (cellular metabolism) was strongly correlated with PC3 (mean rho = +0.76) but also weakly correlated with the negative aspect of PC1 (mean rho = −0.27). Cluster B (immune and inflammatory signaling) was exclusively correlated with the negative aspect of PC2 (rho = −0.32). Clusters C and D were not found to independently correlate with any of the three PCs. Given the independent association of PC2 with Cluster B immune pathways, we postulated that PC2 coordinate position was predominantly driven by intrinsic immune and inflammatory gene expression in these metastases. As support, when the enrichment scores for T cell activation gene sets were mapped onto three-dimensional PCA plots of the metastases, we observed that each gene set had a significant inverse relationship with the PC2 axis ( INTERFERON GAMMA RESPONSE versus PC2: rho = −0.56, p = 2.52e−9 ; INTERFERON ALPHA RESPONSE versus PC2: rho = −0.56, p = 2.89e−9 ; ALLOGRAFT REJECTION versus PC2: rho = −0.49 , p = 2.27e−7) (Fig. 2d and Supplementary Fig. 2d ). Collectively, unbiased computational profiling revealed PC2 coordinate mapping as an effective initial approach to segregate UM metastases with T cell-inflamed transcriptomic attributes.
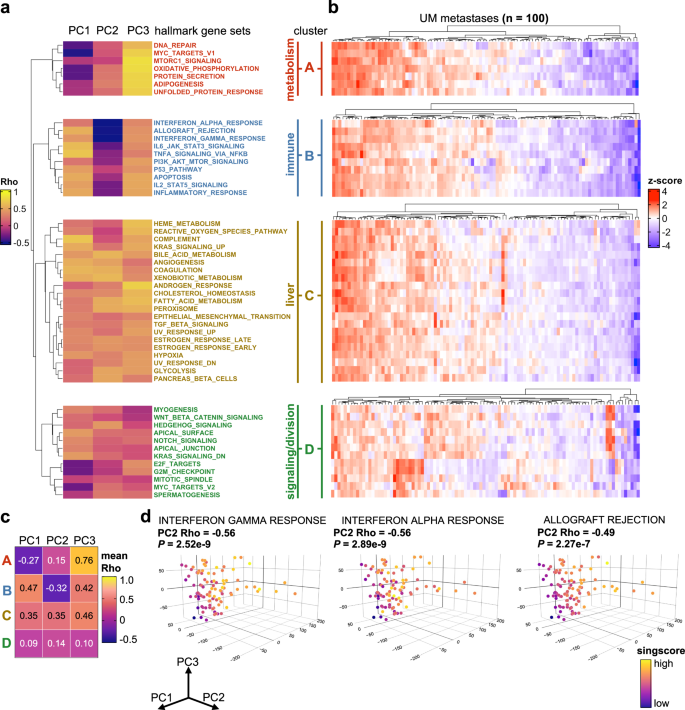
a Unsupervised clustering of Spearman’s rank correlation coefficients derived from correlating PC coordinates (columns) with enrichment of hallmark signatures (row) for each individual metastatic sample ( n = 100). Natural clusters identified by the row dendrogram are split, labeled (A, B, C, D), annotated, and color coded for visualization. b Heatmaps illustrating heterogeneity of hallmark signature enrichment across UM metastases ( n = 100). Rows correspond to hallmark signatures listed in ( a ). Columns within each heatmap represent individual metastases. Each heatmap was clustered by metastases separately to display tumor heterogeneity within each hallmark cluster. Z -scores were calculated per row. c Matrix of mean Spearman’s rank correlation coefficients for each cluster-PC combination. d Three-dimensional PCA plots displaying enrichment scores for selective hallmark immune related pathways identified in cluster B. Euclidean distance was used for hierarchical clustering ( a , b ). Statistical comparisons were performed using Spearman’s rank correlation ( a – d ).
Development of Uveal Melanoma Immunogenomic Score (UMIS)
We next sought to refine the rudimentary PC2 variable into a more specific and clinically applicable immune metric for UM metastases. First, we defined the 2394 genes that positively correlated with immune and inflammatory hallmark gene set enrichment (those with negative PC2 loadings). Rather than biasing this gene list with supervised filtering, we utilized the entire list of 2394 genes to facilitate discovery of novel biologic processes. Further, to enable single-sample prospective analysis, we employed a cohort-independent rank-based gene set scoring method (singscore 18 ) to calculate enrichment scores for individual biopsies based upon transcript abundance (transcripts per million; TPM). Using this approach, we generated a single continuous variable for each metastasis called Uveal Melanoma Immunogenomic Score (UMIS) which reflected the concordance and mean percentile rank of our list of 2394 genes within the sample transcriptome (Fig. 3a ). Cohort-independent UMIS (singscore) correlated strongly with the corresponding cohort-dependent score calculated using established pipelines ( GSVA ), supporting that our single-sample rank-based tool could be used for prospective evaluation of tumor biopsies without batch artifact (Fig. 3b ) 18 . Of the 2394 genes that constitute UMIS, 1527 were protein-coding and the remaining 867 were a mixture of non-coding, unclassified, and pseudo genes (Fig. 3c and Supplementary Data 4 ). Functional annotation of UMIS coding genes confirmed pathways related to immune and inflammatory response (Fig. 3d ). UMIS values ranged from 0.114 to 0.347 across the 100 metastases with a median score of 0.237, which was used as a cutoff to define high and low UMIS groups for categorical comparisons (Fig. 3e ). Gene set enrichment analysis of high versus low UMIS metastases demonstrated that the most significantly enriched pathways were in the high UMIS group and involved T cell activation (Fig. 3f and Supplementary Fig. 3a ). UMIS level was observed to be independent of metastatic site (Fig. 3g and Supplementary Data 5 ), TMB (Fig. 3h and Supplementary Data 4 ), somatic mutations and copy number alterations (Supplementary Fig. 3b and Supplementary Data 6 and 7 ), and class I human leukocyte antigen (HLA) alleles (Supplementary Fig. 3c ). Thus, UMIS represented a unique single-sample gene expression score derived from an unbiased mixture of coding, non-coding, and unannotated transcripts that could rank UM metastases based upon the expression level of immune and inflammatory genes.
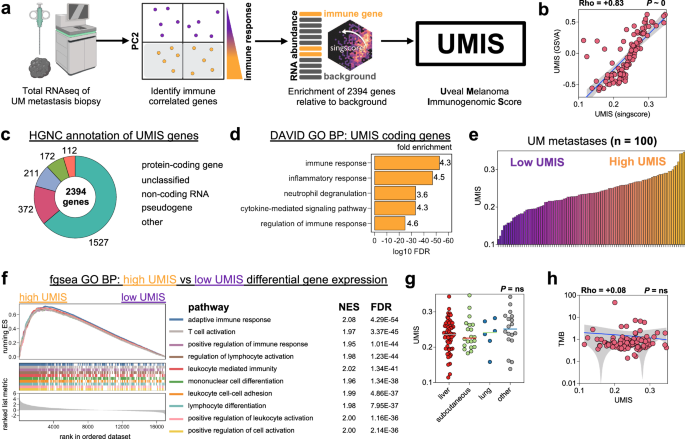
a Workflow for the development of UMIS. Created with BioRender.com. b Correlation of UMIS scores calculated by the cohort-independent method, singscore, with UMIS scores calculated by the cohort-dependent method, gene set variation analysis (GSVA). c Annotation of UMIS genes using Human Genome Organization (HUGO) Gene Nomenclature Committee (HGNC). d Functional annotation of protein-coding genes within UMIS using Database for Annotation, Visualization and Integrated Discovery (DAVID) and Human Molecular Signatures Database Gene Ontology Biological Process gene set collection. e Distribution of UMIS scores across the cohort of 100 metastases. f Gene set enrichment analysis of differentially expressed genes between high UMIS and low UMIS UM metastases. The ten pathways with the lowest FDR are displayed. g Comparison of UMIS by source tissue of resected metastases ( n = 100 biologically independent samples; liver = 56, subcutaneous = 20, lung = 6, other = 18). h Correlation of UMIS with TMB. Statistical comparisons were performed using Spearman’s rank correlation with overlaid simple linear regression to illustrate linearity ( b , h ), DAVID modified Fisher’s exact test ( d ), fast preranked gene set enrichment analysis ( f ), or Kruskal–Wallis test by ranks ( g ).
UMIS uncovers in vivo drivers of T cell recruitment and exclusion
To characterize the tumor microenvironmental cellular attributes contributing to UMIS, we performed whole-tumor single cell transcriptomics of six UM metastases with disparate UMIS values; 3 high UMIS (0.300, 0.268, 0.264) versus 3 low UMIS (0.199, 0.178, 0.162) (Supplementary Fig. 4a, b ). We cataloged the 93,670 analyzed cells by building a unique metastatic UM single cell atlas using a two-step process that first categorized cells into large buckets (tumor, immune, and stroma) then assigned specific cellular and lineage labels (myeloid versus lymphoid) to the immune cellular fraction (Fig. 4a, b ; Supplementary Fig. 4c–e and Supplementary Data 8 and 9 ) 19 , 20 , 21 . Our single cell analysis of low UMIS tumors had expectedly low numbers of immune cells. However, to maintain the true proportional landscape of specific cell types and avoid manipulation induced transcriptomic changes, we profiled the tumor digests without an additional enrichment step. We observed more lymphoid cells (proportion ratio = 10.50, p = 0.047) and fewer tumor cells (proportion ratio = 0.88, p = 0.047) in high UMIS versus low UMIS metastases (Fig. 4b and Supplementary Fig. 4f ). Further, the composition of these lymphoid fractions differed, with the high UMIS metastases being enriched with CD8+ T cells (proportion ratio = 6.31, p = 6.15e−7) and the low UMIS metastases being enriched with CD4+ T cells (proportion ratio = 0.63, p = 3.32e−4) and T helper/Th17 T cells (proportion ratio = 0.25, p = 0.024) (Fig. 4c ). A granular analysis of the lymphoid cells revealed that the high UMIS metastases were enriched for CD8+ exhausted T cells (proportion ratio = 40.73, p = 4.04e−7) and CD8+ cytotoxic T cells (proportion ratio = 4.70, p = 1.13e−5) (Fig. 4d ). Intriguingly, we observed that 9% of the CD8+ exhausted and 20% of the CD8+ cytotoxic TIL retained transcriptomic expression of TCF7 , suggesting possible progenitor capability (Supplementary Fig. 5a ). Differential gene expression of the lymphoid cells revealed the high UMIS metastases had upregulation of genes involving T cell activation ( TNFRSF9, TNFRSF4 ), T cell exhaustion ( PDCD1, CTLA4, LAG3, HAVCR2, VSIR ), lymphocyte activation ( STAT1 ), interferon response ( HLA-A, HLA-B, HLA-C, B2M, IFNGR1, IRF1, IFI27, IFI6, IFITM1, IFITM2, IFITM3 ), T cell memory ( IL7R ), lymphocyte trafficking ( CXCL13, CCR7, SELL, CXCR3 ), and T cell progenitor capability ( TCF7 ) (Fig. 4e ; Supplementary Fig. 5b and Supplementary Data 10 ) 22 , 23 , 24 , 25 , 26 , 27 , 28 . Taken together, these data demonstrate that the TIL found in high UMIS metastases had undergone activation and effector differentiation consistent with an in vivo adaptive anti-tumor response and indicative of a T cell-inflamed microenvironment.
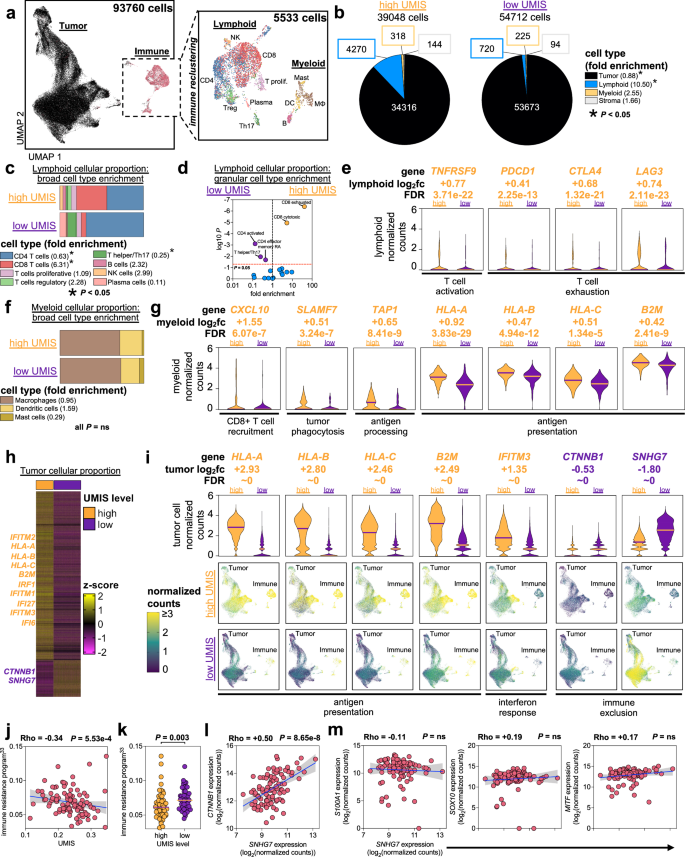
a Uniform manifold approximation and projection (UMAP) plot of all cells analyzed from 6 UM metastases. Magnified panel shows immune subset of cells after reclustering. Cell labeling is with a broad classification. b Proportion of overall cell types within UMIS groups. Fold enrichment refers to proportion ratio (high UMIS/low UMIS). c Proportion of lymphoid broad cell types within UMIS groups. Fold enrichment refers to proportion ratio (high UMIS/low UMIS). d Volcano plot of lymphoid granular cell types within UMIS groups. Fold enrichment refers to proportion ratio (high UMIS/low UMIS). e Selected genes from differential gene expression analysis of high UMIS versus low UMIS lymphoid cells. Bars indicate medians and log 2 fc refers to log2(fold change). f Proportion of myeloid broad cell types within UMIS groups. Fold enrichment refers to proportion ratio (high UMIS/low UMIS). g Selected genes from differential gene expression analysis of high UMIS versus low UMIS myeloid cells. Bars indicate medians and log 2 fc refers to log2(fold change). h Heatmap of differentially expressed genes between high UMIS and low UMIS tumor cells. Columns are individual cells, rows are genes. Cells are grouped by the UMIS level of their metastasis. The genes included had log2(fold change) ≥ |0.5| and FDR < 0.05. Z -scores were calculated per row. i Selected genes from differential gene expression analysis of high UMIS versus low UMIS tumor cells. Bars indicate medians and log 2 fc refers to log2(fold change). UMAP plots display all cells within each UMIS subset. j Correlation of UMIS with immune resistance program scores 33 in UM metastases ( n = 100). k Comparison of immune resistance program scores 33 by UMIS level in UM metastases (high UMIS n = 50, low UMIS n = 50; total n = 100 biologically independent samples). l Correlation of SNHG7 with CTNNB1 transcript expression in UM metastases ( n = 100). Units are log2(normalized counts) from bulk RNAseq. m Correlation of SNHG7 with canonical melanoma marker transcripts (S100A1, SOX10, MITF) in UM metastases ( n = 100). Units are log2(normalized counts) from bulk RNAseq. Statistical comparisons were performed using propeller (arcsin square root transformation of proportions) ( b – d , f ), Wilcoxon rank-sum test (two-tailed) ( e , g , i , k ) and Spearman’s rank correlation with overlaid simple linear regression to illustrate linearity ( j , l , m ).
We next investigated the myeloid cells found in high UMIS versus low UMIS metastases (Fig. 4f and Supplementary Fig. 5c ). Although there was no enrichment of specific myeloid cell types (macrophages, dendritic cells, mast cells) in either group, differential gene expression revealed the high UMIS myeloid cells had upregulated genes involving CD8+ T cell recruitment ( CXCL10, CXCL9 ), tumor phagocytosis ( SLAMF7 ), antigen processing ( TAP1, TAP2) , antigen presentation ( HLA-A, HLA-B, HLA-C, B2M, HLA-DPB1, HLA-DQB1, HLA-DRB1 ) and interferon response ( IRF1, IRF8, IFI27, IFI6 ) (Fig. 4g ; Supplementary Fig. 5d and Supplementary Data 11 ) 24 , 25 , 28 , 29 , 30 , 31 . These findings support that the T cell-inflamed microenvironment found in high UMIS metastases also included more active myeloid lineage antigen presenting cells (APCs) capable of recruiting CD8+ T cells.
Finally, since UMIS was derived using unbiased whole-tumor transcriptomics we postulated that this score may also reflect intrinsic differences among the tumor cells within high versus low UMIS metastases. Upon reclustering of tumor cells, we confirmed distinct separation of cells derived from high versus low UMIS metastases (Supplementary Fig. 6a ). Differential gene expression revealed high UMIS tumor cells had significantly increased expression of several interferon-inducible transcription factors and elements ( IRF1 , IFI27, IFI6, IFITM1, IFITM2, IFITM3 ) and each of the major histocompatibility complex (MHC) class I molecule heterodimer components ( HLA-A, HLA-B, HLA-C, B2M ) (Fig. 4h, i ; Supplementary Fig. 6b and Supplementary Data 12 ) 23 , 24 , 25 . These findings suggested that high UMIS metastases were composed of IFN-γ primed tumor cells that had upregulated MHC class I expression in response to chronic IFN-γ secretion from tumor specific CD8+ T cells 23 , 24 . In contrast, low UMIS tumor cells had 1.44-fold higher expression of CTNNB1 (log 2 (fold change) = −0.53, FDR ~ 0) which encodes the beta-catenin protein (Fig. 4h, l and Supplementary Data 12 ). Activation of the Wnt/beta-catenin pathway has been implicated in T cell exclusion and may explain the paucity of CD8+ T cell infiltrate in low UMIS metastases 30 , 32 . In support, we found a significant inverse relationship across the total metastatic cohort ( n = 100) between UMIS and the expression of a previously reported immune resistance program (Fig. 4j, k ) 33 . Interestingly, the most upregulated gene in low UMIS tumor cells was the long non-coding RNA, SNHG7 , which was 3.48-fold upregulated in low UMIS tumor cells (log 2 (fold change) = −1.80, FDR ~ 0) and has been previously reported as a positive regulator of CTNNB1 expression in several cancers (Fig. 4h, i and Supplementary Data 12 ) 34 , 35 , 36 , 37 , 38 . Our findings confirmed a strong association between SNHG7 and CTNNB1 expression level in UM metastases ( n = 100) (Fig. 4l ) that was independent of tumor cell abundance as measured by melanoma-specific gene expression ( S100A1, SOX10, MITF ) and total RNA quantity ( ACTB, GAPDH ) (Fig. 4m and Supplementary Fig. 6c ).
In sum, single cell transcriptomics demonstrated that UMIS was a holistic metric that reflected the gene expression of the lymphoid, myeloid, and tumor compartments within the tumor microenvironment. Further, UMIS classification of metastases revealed increased CTNNB1 expression by low UMIS tumors cells as a putative driver of immune exclusion. In contrast, high UMIS metastases displayed lower tumor cell CTNNB1 expression, more activated APCs, greater CD8+ T cell recruitment, and robust interferon signaling.
UMIS predicts anti-tumor potency of ex vivo expanded TIL
To validate the transcriptomics demonstrating T-cell inflamed gene expression, we next interrogated the specific anti-tumor potency of the endogenous TIL from each of the UM metastases. Currently, the assessment of TIL tumor reactivity requires patients to undergo surgical resection of metastases followed by several weeks of ex vivo TIL expansion and finally resource intensive coculture with autologous tumor cells. Thus, we also investigated whether UMIS could serve as a rapid and minimally invasive clinical tool to predict the tumor specific potency of endogenous TIL. We compared UMIS values, derived from a single random biopsy from each source metastasis ( n = 100), with the level of TIL anti-tumor reactivity found after conventional ex vivo expansion (Fig. 5a ). TIL cultures ( n ~ 24) were initiated from each freshly resected metastasis using a standardized ex vivo tumor fragmentation approach to address tumor heterogeneity, as previously described 15 . The individual TIL fragment cultures were tested for tumor specificity by coculture with autologous tumor digest (versus normal tissue controls) followed by measurement of 4-1BB upregulation on CD3+ cells (flow cytometry) and IFN-γ release (ELISA), which were found to be strongly correlated (Fig. 5b, c ). The percentage of TIL cultures having tumor-specific reactivity from each metastasis was used as a standardized reactivity metric for comparing the level of anti-tumor TIL responses across tumors (Fig. 5d ). We found the frequency of tumor reactive TIL cultures varied significantly among the total cohort (median = 6%; range = 0–100%) with 55 metastases having measurable anti-tumor reactivity and the remaining 45 metastases with no detected reactivity (Fig. 5e ). Further, the metastases that had undergone prior ICI ( n = 53) and tebentafusp therapy ( n = 12) showed no difference in the mean percentage of tumor reactive TIL cultures when compared to samples that had not undergone these treatments (ICI 22% vs. no ICI 23%, p = ns; tebentafusp 32% vs. no tebentafusp 21%, p = ns) (Fig. 5e and Supplementary Data 13 ). The percentage of tumor reactive TIL cultures was also independent of metastatic site, TMB, specific mutation expression, copy number alterations, and class I HLA alleles (Supplementary Fig. 7a–d and Supplementary Data 13 – 15 ). When UMIS of each source metastasis was compared to the percentage of tumor reactive TIL cultures that were generated several weeks later, we found a strong positive correlation (rho = +0.47, p = 7.06e−7) (Fig. 5f ). Notably, reactive TIL cultures were rarely expanded from metastases with a UMIS less than 0.2, suggesting the use of this cutoff as a preoperative threshold to avoid futile surgical resection of non-inflamed UM metastases. Interestingly, we did observe a small subset of discordant metastases ( n = 16) with high UMIS values that yielded TIL with no detectable anti-tumor reactivity based upon coculture with autologous tumor digest (Fig. 5f ). However, upon assessing the quality of these specific tumor digest samples, we found they had significantly lower viability when compared to digests ( n = 34) that yielded concordance between high UMIS and co-culture reactivity (median digest viability: 78% vs. 93%, p = 0.046) (Fig. 5f and Supplementary Fig. 7e ). Since UMIS quantitation was neither associated with nor dependent upon tumor digest viability (Supplementary Fig. 7f ), the discordance with anti-tumor reactivity observed with this outlier subset likely stemmed from insufficient stimulatory capacity of these low viability tumor digests. To further characterize the performance of UMIS in our discovery cohort of 100 UM metastases, we benchmarked its ability to predict co-culture anti-tumor reactivity against several other tumor biopsy metrics including TMB, percentage of infiltrating CD8+ T cells, and several published gene expression profiles for T cell inflammation (Fig. 5g, h and Supplementary Data 16 ) 30 , 39 , 40 , 41 . We found that UMIS was the strongest performer as both a correlative metric (rho = +0.47, p = 7.06e−7) and classification metric (AUC = 0.85) for predicting ex vivo TIL reactivity (Fig. 5g, h ). Not surprisingly, TMB had no predictive value (rho = +0.01, p = ns; AUC = 0.51) (Fig. 5g, h ). In further support of UMIS as a preoperative biomarker, we found that UMIS level could identify metastases with the greatest yield of tumor reactive TIL among synchronous metastases in individual UM patients (Fig. 5i ). In a prospective and independent validation cohort of metastatic UM biopsies, we corroborated the predictive ability of UMIS for ex vivo TIL reactivity ( n = 20, AUC = 0.76) (Fig. 5j ). Additionally, we validated that UMIS remained consistent across spatially distinct areas of individual tumors (Supplementary Fig. 8a ) and could also be obtained from minimally invasive core biopsies (Supplementary Fig. 8b ). Taken together, these findings establish that UMIS, obtained from a metastatic biopsy, could serve as a minimally invasive preoperative biomarker to both identify UM metastases harboring tumor reactive TIL and predict the percentage of tumor reactive TIL cultures that could be expanded without the limitations associated with conventional coculture assays.
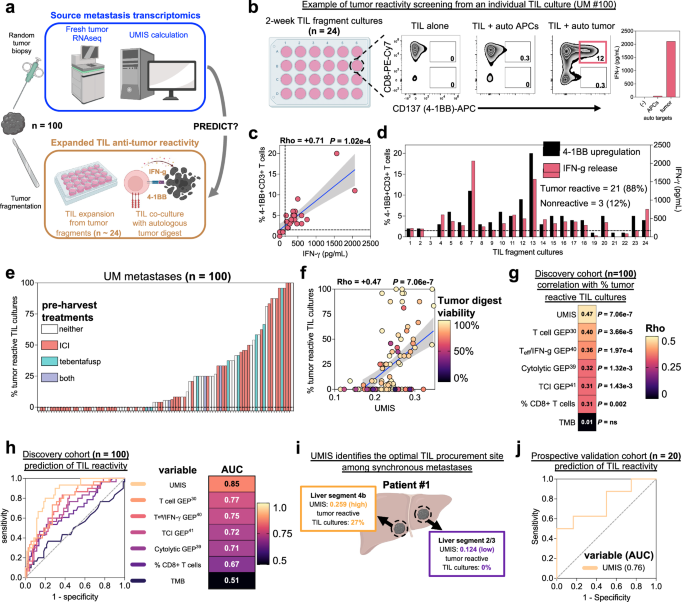
a Workflow for parallel analysis of source tumor transcriptomics and expanded TIL anti-tumor reactivity. Created with BioRender.com. b Example of TIL culture anti-tumor reactivity screening from source tumor UM #100. From left, tumor fragments ( n = 24) are cultured individually for ~2 weeks before overnight coculture with autologous tumor cells and measurement of 4-1BB (CD137) expression by flow cytometry and IFN-γ release by ELISA. Final reactivity measurement subtracts background reactivity of TIL (TIL alone) and non-specific reactivity (TIL + autologous APCs). c Correlation between %4-1BB + CD3+ cells and IFN-γ release among the 24 fragment cultures from UM #100 after overnight tumor coculture. d Individual TIL fragment culture anti-tumor reactivity as assessed by 4-1BB upregulation and IFN-γ release from source tumor UM #100. TIL cultures were classified as tumor reactive if their 4-1BB expression was >1% (dotted line) and twice background or IFN-γ release was >100 pg/ml (dotted line) and twice background. Percentage tumor reactive TIL cultures was defined as 100*(tumor reactive TIL cultures)/(total TIL cultures). e Distribution of percent tumor reactive TIL cultures among the cohort of 100 metastases color-coded by pre-harvest treatments. f Correlation of UMIS with percent tumor reactive TIL cultures. Color of each point denotes tumor digest viability percentage. g Correlative benchmarking of UMIS against published gene expression profiles and tumor biomarkers ( n = 100 metastases). All correlations are with percent tumor reactive TIL cultures. h Predictive benchmarking of UMIS against published gene expression profiles and tumor biomarkers ( n = 100 metastases). Receiver operating characteristic (ROC) curves and accompanying statistics are for variables’ prediction of ≥33% tumor reactive TIL cultures. i Disparate UMIS and TIL culture reactivity from synchronous hepatic metastases in UM patient #1. Created with BioRender.com. j Validation of UMIS’ ability to predict ex vivo TIL reactivity in an independent metastatic biopsy cohort ( n = 20 metastases). Receiver operating characteristic (ROC) curve and area under curve (AUC) value is for UMIS’ prediction of ≥33% tumor reactive TIL cultures. Statistical comparisons were performed using Spearman’s rank correlation with overlaid simple linear regression to illustrate linearity ( c , f , g ) or univariate logistic regression ( h , j ). Gene expression profiles were calculated using singscore to best assess their cohort-independent predictive ability (Supplementary Data 16 ) 30 , 39 , 40 , 41 .
UMIS identifies quiescent TIL resistant to ICI and tebentafusp but sensitive to ex vivo expansion and adoptive transfer
Having found that UMIS strongly correlated with the level of TIL anti-tumor reactivity in metastases, we were surprised to find that high UMIS status was not significantly associated with improved survival in our UM cohort (Supplementary Fig. 9a ). Furthermore, despite discovering tumor reactive TIL within the metastases of 23 UM patients (50%) who received prior ICI and 7 patients (78%) who received tebentafusp, we noted that none of these patients showed objective tumor regression with these therapies. To investigate these paradoxical findings, we analyzed the intratumoral TCR repertoire within the source metastases 42 . We found that the in situ diversity of the TCR beta (TRB; n = 88) and TCR alpha (TRA; n = 82) chains varied significantly across the total cohort of metastases (Shannon index ranges; TRB = 0.08–4.84, TRA = 0.69–4.72) (Fig. 6a and Supplementary Fig. 10a ). UMIS was found to strongly correlate with both TRB diversity (rho = +0.54, p = 5.40e−8) (Fig. 6a ) and TRA diversity (rho = +0.45, p = 2.14e−5) (Supplementary Fig. 10a ) suggesting that high UMIS metastases had more polyclonal T cell infiltrates. In contrast, TRB and TRA clonality, an in vivo surrogate for relative TIL clonal expansion, was low and minimally variant across the samples (1 − Pielou’s index ranges; TRB = 0–0.89, TRA = 0–0.23) (Fig. 6a and Supplementary Fig. 10a ). We expectedly found no correlations between UMIS and the clonality of the TRB (rho = +0.02, p = ns) (Fig. 6a ) and TRA (rho = −0.07, p = ns) chains (Supplementary Fig. 10a ). The in vivo quiescence of these TIL was further corroborated by single cell TCR repertoire analysis demonstrating low clonality (Supplementary Fig. 10b ) and single cell transcriptomics which found that the percentage of proliferative T cells was equivalently low in high and low UMIS metastases (Figs. 4c and 6b ). Interestingly, prior ICI therapies ( n = 53) had no influence on TCR diversity compared with untreated samples (Fig. 6a, c and Supplementary Fig. 10a, d ). In contrast, prior tebentafusp treatment ( n = 12) was associated with greater TCR diversity, consistent with the ability of this bispecific T cell engager to recruit T cells to these metastases (Fig. 6a, c and Supplementary Fig. 10a, c, d ). However, neither prior ICI nor tebentafusp therapy were associated with an increase in TCR clonality (Fig. 6a, c and Supplementary Fig. 10a, c, d ), indicating that these immunotherapies were incapable of inducing in vivo proliferation of the endogenous TIL. When specific TRB and TRA sequences were compared across the metastases ( n = 100), we found that most of the sequences were private, with rare and limited public expression suggesting unique, rather than shared, antigen targeting (Supplementary Fig. 10e ). Cumulatively, these TCR repertoire studies demonstrated that although high UMIS metastases were infiltrated with a unique polyclonal population of TIL, these T cells remained quiescent, even after receiving ICI and tebentafusp therapy.
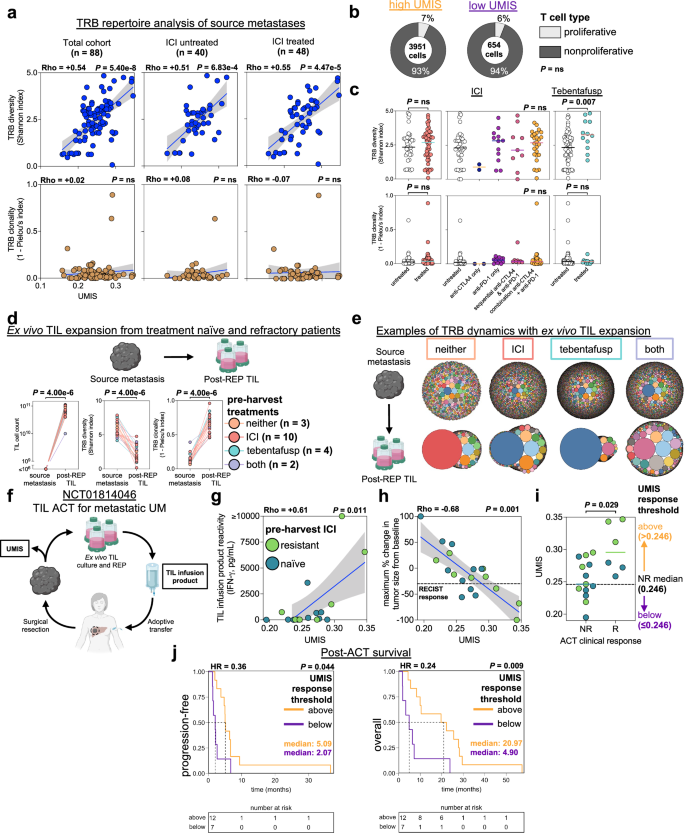
a T cell receptor beta (TRB) repertoire analysis of bulk RNAseq of UM metastases ( n = 88). Immune checkpoint inhibition (ICI) refers to treatment history prior to metastatic biopsy. b Proportion of proliferative T cells in UMIS groups by single cell atlas. c Comparisons of TRB diversity and clonality in ICI or tebentafusp untreated versus treated metastases (ICI: 48 treated, 40 untreated; tebentafusp: 12 treated, 76 untreated). d Ex vivo TIL expansion from treatment naïve and refractory patients ( n = 19). Listed therapies were received prior to metastatic biopsy. Changes in TIL cell counts (left), T cell receptor beta (TRB) diversity (middle), and TRB clonality (right) are shown for source metastases and corresponding TIL cultures post rapid expansion protocol (post-REP TIL). Metastases’ TIL counts were conservatively estimated to be ≤10 6 . TRB repertoires were characterized with targeted TCR repertoire analysis. Schematic created with BioRender.com. e Examples of TRB dynamics with ex vivo TIL expansion. Bubble plots represent unique TRB clonotypes (color coded) with bubble size indicating percentage of total clonotypes. Shown are representative examples for each pre-harvest treatment group (neither = UM #73, ICI = UM #50, tebentafusp = UM #59, both = UM #49). Schematic created with BioRender.com. f Schematic for evaluation of UMIS in the context of NCT01814046 (ACT of TIL for metastatic UM) 15 . Created with BioRender.com. g Correlation of source metastasis UMIS with TIL infusion product reactivity ( n = 17). h Correlation of source metastasis UMIS with maximum percent change in tumor size from baseline (RECIST v1.1) after TIL ACT. RECIST response line is drawn at −30% ( n = 19). i Comparison of UMIS between responders (R; n = 6) and nonresponders (NR; n = 13) to TIL ACT. The median UMIS of the NR group (0.246) was used as a clinical response threshold for outcome analyses. j Time-to-event curves of post-ACT survivals by UMIS response thresholds ( n = 19). Progression-free survival used progressive disease as the event (median follow-up (months): high = 5.09, low = 2.07). Overall survival used death as the event (median follow-up (months): high = 20.97, low = 4.90). Hazard ratios (HR) are for above versus below threshold groups. Statistical comparisons were performed using Spearman’s rank correlation with overlaid simple linear regression to illustrate linearity ( a , g , h ), Fishers exact test ( b ), Wilcoxon rank-sum test (two-tailed) ( c , i ), Kruskal–Wallis test by ranks ( c ), Wilcoxon signed-rank test (two-tailed) ( d ) or logrank test ( j ).
To determine whether the deficient proliferation of the intratumoral T cells was due to T cell exhaustion or other intrinsic proliferative defects, we performed clinical scale ex vivo rapid expansion (REP) of TIL from UM metastases that were either naïve to ICI and tebentafusp ( n = 3), or refractory to ICI ( n = 10), tebentafusp ( n = 4), or both therapies ( n = 2) (Fig. 6d, e and Supplementary Fig. 11a–c ). We observed that TIL from each of the metastases demonstrated approximately 5-log expansion, reaching massive cell counts (median = 7.31e10, range = 1.00e10–1.12e11) (Fig. 6d, e and Supplementary Fig. 11a ). Further, these expanded TIL demonstrated a significant decrease in TCR diversity ( p = 4e−6) and a significant increase in TCR clonality ( p = 4e−6) as compared to their source metastases using highly specific targeted TCR sequencing (Fig. 6d, e and Supplementary Fig. 11a–c ). These findings suggested that the endogenous TIL were not limited by intrinsic proliferative deficiencies, but instead their growth was likely suppressed by the tumor microenvironment. Taken together, we observed the quiescence of endogenous TIL in UM metastases was not reversed with ICI or tebentafusp but could be revived with ex vivo liberation and expansion.
Based upon the observation that UMIS from a metastatic biopsy could predict the ex vivo potency of quiescent endogenous TIL, we postulated that UMIS might also predict the clinical efficacy of adoptive transfer of these TIL after ex vivo liberation and expansion (Fig. 6f ). Of the 100 UM metastases profiled, 19 had been used to manufacture TIL for a previously reported ACT trial in patients with metastatic UM (NCT01814046) 15 . Among this treatment cohort, which included 6 responders and 13 nonresponders, we observed a strong correlation between source tumor UMIS and the ex vivo anti-tumor reactivity of the post-REP TIL infusion product ( n = 17, rho = +0.61, p = 0.011; 2 infusion products were not tested due to insufficient tumor) (Fig. 6g ). Additionally, we found that UMIS as a continuous variable strongly correlated with magnitude of clinical tumor regression after adoptive transfer in patients with metastatic UM, including ICI refractory individuals ( n = 19, rho = −0.68, p = 0.001) (Fig. 6h ). To help define a UMIS threshold value that might have future clinical utility in predicting RECIST objective responses (≥30% reduction), we utilized the median UMIS value of the non-responder group (UMIS = 0.246) as a response threshold (Fig. 6i ). We observed that patients having source metastases above this threshold had significantly improved progression-free and overall survival after TIL ACT versus those below the threshold (Fig. 6j ). In sum, these findings demonstrate that UMIS, performed on a pre-treatment metastatic biopsy, correlated with the clinical outcome after adoptive transfer of TIL and may serve as a future predictive biomarker for the treatment of metastatic UM with ACT.
Here we profiled the immunogenomic landscape of metastatic UM using bulk and single cell transcriptomics, TCR repertoire analysis, TIL reactivity assessment, and clinical adoptive cell therapy. Our findings establish that metastatic UM is not an immunologically ‘cold’ cancer, but instead over half of the analyzed UM metastases harbored tumor reactive TIL, despite having one of the lowest mutational burdens of any solid cancer 4 , 9 , 43 , 44 and an equally limited responsiveness to approved immunotherapies, including ICI therapy and tebentafusp 4 , 7 , 8 , 12 , 13 , 45 , 46 . To avoid sampling bias in our study, we analyzed a large clinically representative group of UM patients ( n = 84) and their metastases ( n = 100) which were procured from a diverse array of organ sites ( n = 11). Further, the metastases in the current study were genomically validated to be of uveal origin by expression of canonical UM somatic alterations and low TMB 4 , 9 , 43 , 47 , 48 . Thus, we believe our study cohort to accurately represent the metastatic immunogenomic landscape of this rare cancer and uniquely suited to answer two critical questions that have significant therapeutic implications for UM: what factors drive T cell inflammation in metastatic UM, and why does metastatic UM respond so poorly to currently approved immunotherapies?
To define drivers of immune response against metastatic UM, we utilized bulk total RNA sequencing of metastatic biopsies and found that T cell-inflamed metastases naturally segregated from T cell excluded metastases based upon an unsupervised transcriptomic signature composed of coding, non-coding, and unannotated transcripts. Rather than biasing this gene list with supervised filtering, we integrated the entire 2394 gene set into a gene expression score called UMIS. Based upon a unique single cell transcriptomic atlas that we developed specifically for metastatic UM, we found that UMIS could holistically reflect the multiple cellular components of the tumor microenvironment 49 . Metastases with low UMIS (versus high UMIS) had a paucity of TIL and were composed of tumor cells with higher beta-catenin transcript expression ( CTNNB1) , which has been described as a transcriptional repressor of BATF3 -lineage dendritic cell recruitment of CD8+ T cells 30 , 50 , 51 . In contrast, high UMIS metastases had lower tumor cell expression of CTNNB1 , increased APC expression of T cell chemoattractant ligands ( CXCL10 and CXCL9) , greater tumor reactive TIL recruitment, and markedly elevated MHC expression on multiple cell populations within the tumor microenvironment, suggesting prominent interferon signaling. Thus, we believe Wnt/beta-catenin signaling to play an important role in promoting immune exclusion in metastatic UM, similar to prior reports in metastatic CM 30 , 32 , 50 , 51 , 52 . Surprisingly, we identified only a single metastasis with a possible activating somatic mutation of the Wnt/beta-catenin pathway, suggesting hotspot mutations are not a common driver of beta-catenin overexpression in UM metastases 53 . However, we did observe a strong correlation between the expression of the long non-coding RNA, SNHG7 , and CTNNB1 . Based upon several reports that SNHG7 is a positive regulator of CTNNB1 and compelling evidence that in vitro knockdown of SNHG7 leads to downregulation of the Wnt/beta-catenin pathway in various other cancers 34 , 35 , 36 , 37 , 38 we are investigating the mechanistic role of this non-coding RNA in driving T cell exclusion in UM metastases and therapeutic strategies to potentially abrogate its effect in low UMIS metastases.
To better understand why UM metastases rarely regress with approved immunotherapies, we evaluated TIL from ICI and tebentafusp resistant patients. From our total cohort of TIL samples ( n = 100), we observed that 55% of UM metastases harbored tumor reactive TIL and there was no difference in the percentage of tumor reactive TIL cultures expanded from ICI and tebentafusp treated metastases versus untreated. Yet, despite the presence of potent TIL in these metastases, we found they were strikingly quiescent with an absence of in vivo TIL expansion using TCR clonality analysis and single cell transcriptomics. Interestingly, we observed prior tebentafusp therapy was associated with increased in vivo TCR diversity in our samples, demonstrating its ability as a T cell recruiter 12 , 13 . However, neither tebentafusp nor ICI therapy were associated with an increase in TCR clonality. The quiescence of these T cells within the tumor microenvironment may explain the low rates of objective tumor regression and the intriguing decoupling of overall response rate as a surrogate for overall survival in UM patients treated with tebentafusp 12 , 13 , 54 . In contrast, TCR repertoire studies of cutaneous melanoma metastases have reported significant variance in TCR clonality, with higher pre-treatment clonality being associated with improved response to PD-1 blockage 55 , 56 , 57 , 58 . Interestingly, we found the quiescent TIL from ICI and tebentafusp treated UM metastases could demonstrate significant ex vivo expansion, indicating that these T cells were not limited by intrinsic factors such as exhaustion, but rather by extrinsic constraints within the UM tumor microenvironment. In support, we previously observed that adoptive transfer of tumor reactive TIL could mediate objective regression in ICI refractory UM patients 15 . In sum, these findings reveal that occult T cell responses do exist against metastatic UM but require therapeutic strategies such as ACT to overcome their growth-suppressed state within the tumor microenvironment.
Finally, our study revealed the importance of UMIS as a tumor intrinsic biomarker to predict TIL potency and clinical response after adoptive transfer in UM patients. Whereas recent reports have proposed phenotypic and transcriptomic markers for the purpose of defining neo-antigen specific TCR sequences from TIL 27 , 59 , 60 , we believe UMIS represents a unique tumor biomarker for the identification of tumor reactive TIL capable of ex vivo expansion for clinical adoptive transfer. Importantly, a UMIS level of less than 0.2 identified metastases that were unlikely to yield potent TIL, suggesting that preoperative UMIS measurement could prevent futile invasive surgical harvests. We found UMIS performed significantly better as a tumor intrinsic biomarker of TIL potency when compared to several focused gene expression signatures of T cell inflammation. We postulate that the superior performance of UMIS was a result of its unique derivation from a large unbiased mixture of coding and non-coding transcripts. Further, rather than narrowly reflecting the gene expression of only immune cells, UMIS was developed as a whole-tumor metric that reflected the gene expression of the lymphoid, myeloid, and tumor compartments within the tumor microenvironment.
Potential limitations of our study include selection bias of the patients and metastases analyzed. The rare nature of UM limited our sample size to 100 metastases. A subset of patients contributed multiple metastases to the study (14 patients with multiple metastases included). We chose to include these metastases to maximize sample size in this rare cancer but recognize that this may be a source of selection bias. Given that UM patients presented with the intent of ACT screening, which has strict eligibility requirements, the analyzed cohort may not represent elderly individuals or those demonstrating rapidly progressive metastatic disease and declining performance. Further, although UMIS was found to correlate with TIL reactivity across 24 geographically unique tumor fragments, the predictive ability of UMIS in larger and more heterogenous lesions needs further study. Finally, while our ongoing independent validation cohort ( n = 20) has corroborated the ability of UMIS to predict TIL reactivity, longer clinical follow up is required to evaluate survival in this group of patients.
Patient samples and clinical annotation
Patients were screened and tumor samples were obtained after informed consent in conjunction with tumor procurement banking protocols associated with two adoptive TIL transfer clinical trials: NCT03467516 (Hillman Cancer Center, UPMC, Pittsburgh, PA, USA) and NCT01814046 (Surgery Branch, NCI, Bethesda, MD, USA). Patients gave informed consent in accordance with the Declaration of Helsinki, and the trials were reviewed and approved by the NCI and UPMC Institutional Review Boards. There was no requirement for previous systemic therapy, given the lack of known effective systemic treatments for metastatic UM at the time of study. If patients did receive previous systemic treatment, more than 4 weeks must have elapsed before initiation of the current trial therapy, and patients’ toxicities must have recovered to a grade 1 or less (except for toxicities such as alopecia or vitiligo). All patients were required to have progressive and measurable metastatic disease with an Eastern Cooperative Oncology Group performance status of 0 or 1 and life expectancy greater than 3 months at the time of enrollment. Patients were required to have adequate hematological, renal, and hepatic function. Patients were excluded if they had active systemic infections, coagulation disorders, or other active major medical illnesses of the immune system 15 .
Clinical information, including demographics and treatments, were collected relative to the date of metastatic tumor harvest. Sex was self-reported by patients and consistent with biological sex determined by genomic analysis. Time-to-event data was collected relative to multiple dates: date of primary diagnosis, date of metastatic diagnosis, and date of ACT (if applicable). In cases of patients with multiple metastatic biopsies an algorithm was adopted for selection of a representative biopsy for the purposes of patient-centered time-to-event analysis (in descending order of priority: metastasis harvested prior to any ACT, metastasis whose TIL was utilized for subsequent ACT, more recently harvested metastasis. Response to ACT was evaluated using RECIST v1.1 criteria 61 .
Tumor procurement, ex vivo TIL culture and tumor reactivity testing
All patients had surgical metastatectomies as screening for clinical trials NCT03467516 (Hillman Cancer Center, UPMC, Pittsburgh, PA, USA) and NCT01814046 (Surgery Branch, NCI, Bethesda, MD, USA) to procure tumor tissue to generate autologous TIL for therapy 16 . After surgical procurement of a metastatic lesion, the fresh tumor underwent sterile dissection in the UPMC Immunological and Cell Products Laboratory (Pittsburgh, PA, USA) or the Surgery Branch Cell Production Facility (Bethesda, MD, USA). Representative samples of tumor were sent for formal pathological confirmation of UM. Tumor samples procured at the Surgery Branch, NCI, were transferred via a Material Transfer Agreement to the University of Pittsburgh where the analyses were conducted. To develop a clinically relevant core biopsy approach for in situ tumor characterization, a single random biopsy was obtained from each resected metastasis (~2 mm central core fragment from 93 metastases and ~500,000 cells post tumor dissociation from 7 metastases) and were snap-frozen in liquid nitrogen and stored long term at −80 °C for future DNA and RNA extraction.
TIL cultures were initiated from geographically discrete 1–2 mm 3 tumor fragments ( n ~ 24) that were placed individually in wells of a 24-well culture plate containing complete media with human AB serum and recombinant interleukin-2 (6000 IU/ml; Clinigen). Remaining fresh tumor was processed by mechanical and enzymatic digestion with the human Tumor Dissociation Kit (Miltenyi Biotec) and gentleMACS Dissociator (Miltenyi Biotec) to provide a single cell suspension of autologous tumor targets for TIL reactivity testing. Tumor digests underwent flow cytometric phenotyping with propidium iodide followed by the following anti-human monoclonal antibodies: CD3-APC-Cy7, CD8-PE-Cy7, CD4-PE (BD Biosciences). Tumor digest viability was determined by percent propidium iodide negative cells by flow cytometry or percent trypan blue negative cells by manual cell counting (UM #47). After ~2 weeks of growth, individual TIL fragment cultures were tested for tumor specificity by coculture with autologous tumor cells (versus normal tissue controls) followed by measurement of 4-1BB upregulation on CD3+ cells by flow cytometry using anti-human CD137 (4-1BB)-APC (BD Biosciences) and IFN-γ release by ELISA 14 , 15 . Tumor single cell suspensions (digests) and peripheral blood mononuclear cells were cryopreserved in freezing media and stored long term in liquid nitrogen. Monocytes were isolated from peripheral blood mononuclear cells using the CD14+ MicroBead isolation kit (Miltenyi Biotec). All flow cytometry data was analyzed with FlowJo v10.8 Software (BD Life Sciences).
Clinical scale ex vivo rapid expansion protocol (REP) involved selection of individual fragment T cell cultures for further expansion based on proliferative capacity and evidence of autologous tumor reactivity. Final large-scale expansion of selected TIL cultures was done with anti-CD3 antibody (30 ng/ml, Ortho Biotech or 50 ng/ml, Miltenyi Biotec) and recombinant interleukin-2 (3000 IU/ml; Clinigen) in the presence of irradiated peripheral blood mononuclear feeder cells 15 . The specific anti-tumor reactivity of the infused TIL from NCT01814046 (Surgery Branch, NCI, Bethesda, MD, USA) was assessed by ELISA-based assays. Following overnight co-culture of the TIL with their autologous source tumor, the supernatant from these respective co-cultures was assessed by ELISA to determine the tumor-induced IFN-γ production as described previously 15 .
DNA extraction, library preparation, sequencing, and somatic analysis
Whole genome sequencing of the majority of samples was performed at the UPMC Genome Center ( n = 93). Genomic DNA was isolated from tumor samples or peripheral blood mononuclear cells on the automated Chemagic 360 (PerkinElmer) instrument according to the manufacturer’s instructions. Extracted DNA was quantitated using Qubit dsDNA BR Assay Kit (Thermo Fisher Scientific). DNA libraries were prepared using the KAPA Hyper Plus Kit (KAPA Biosystems). Genomic DNA was processed through fragmentation, enzymatic end-repair and A-tailing, ligation, and quality check a Standard Sensitivity NGS Fragment Analyzer Kit (Agilent). Libraries with an average size of 450 base pairs (range = 300–600 base pairs) were quantified by qPCR on the LightCycler 480 (Roche) using the KAPA qPCR quantification kit (KAPA Biosystems). The libraries were normalized and pooled as per manufacturer protocol (Illumina). Sequencing was performed using the NovaSeq 6000 platform (Illumina) with 151 base pair paired end reads to an average target depth of 70X coverage. The sequencing data was demultiplexed with bcl2fastq2 v2.20 (Illumina) to produce the fastq files.
The samples were mapped with Sentieon v1.3.4 62 . Somatic variants were called by TNhaplotyper2 on tumor-normal mode with the best-practice recommended whole genome sequencing setting. Variants were annotated with Funcotator from GATK v4.0.5 63 . Copy number alterations were called with an in-house developed ensemble method (CNVsenate) with mapped BAM and somatic SNV VCF files. CNVsenate gathers calling results from GATK v4.0.5 63 , CNVkit v0.9.5 64 , CNVnator v0.2.7 65 , Manta v1.3.2 66 , Sentieon CNV (201911) 62 and combines calling with SURVIVOR2 v1.0.3 67 , then uses machine learning and event size for filtering. The filtered results were annotated with AnnotSV v1.1.1 68 for affected genes. The denoised copy ratio for chromosomal segments from GATK was primarily used. A customized script was used to calculate the denoised copy ratio for chromosomal arms. Copy number gain was defined as chromosomal arm denoised copy ratio ≥1.25, while copy number loss was defined as chromosomal arm denoised copy ratio ≤0.80.
Somatic SNV VCF files were converted to MAF format with vcf2maf v1.6.19 69 and annotated with VEP v102 70 . The MAF cohort was filtered with a genomic data commons-like strategy, including for population allele frequency <2%, coding regions, and presence in dbSNP 71 and COSMIC 72 . Further filtering was done for only somatic mutations with variant allele frequency ≥5%. Mutations were manually tabulated for one sample that was unable to be processed into the MAF format (UM #20). All computational processes above were performed on a linux-based amazon web services ec2 instance on the DNAnexus platform (DNAnexus). The MAF file was then processed and summarized using maftools v2.10.05 73 .
For six samples (UM #4, #13, #22, #23, #26 and #30) without sufficient tumor tissue for whole genome sequencing we utilized whole exome sequencing performed as described previously 14 to assess for canonical UM somatic mutations. For one sample (UM #53) without sufficient tumor tissue for whole genome sequencing DNA and RNA were extracted from paraffin embedded tumor tissue and processed with the Oncomine Comprehensive Assay v3 DNA and RNA primer sets (Thermo Fisher Scientific) according to the manufacturer’s protocol. Alterations assessed were per the UPMC Oncomine panel which has been described previously 74 .
RNA extraction, library preparation, sequencing, and read alignment
Total RNA was isolated from tumor samples on the automated Chemagic 360 (PerkinElmer) instrument according to the manufacturer’s instructions. Extracted RNA was quantitated with the Qubit RNA BR Assay Kit (Thermo Fisher Scientific) followed by an RNA quality check using Fragment Analyzer (Agilent). For each sample, RNA libraries were prepared from 100 ng of RNA using the KAPA RNA HyperPrep Kit with RiboErase (Kapa Biosystems) according to the manufacturer’s protocol, followed by a quality check using Fragment Analyzer (Agilent) and quantification by qPCR with the Kapa qPCR quantification kit (Kapa Biosystems). The libraries were normalized, pooled, and sequenced using the NovaSeq 6000 platform (Illumina) to an average of ~50 million 101 base pair paired end reads. The sequencing data was demultiplexed with bcl2fastq2 v2.20 (Illumina) to produce the fastq files.
Bulk transcriptomic computational analyses
Sequencing data was quality controlled with FastQC v0.11.7 75 before and after adapter trimming with cutadapt v1.18 76 along with assessment of estimated ribosomal content with sortmerna v4.3.4 77 . Trimmed reads were then aligned with STAR v2.7.5a 78 using the Gencode v38 GTF and GRCh38 fasta references 79 . Uniquely mapped percentage of reads and total uniquely mapped reads metrics after STAR mapping were used as further quality control metrics. The BAM file was indexed with samtools v1.10 80 . Gene counts from the STAR BAM files were calculated with htseq-count v0.13.5 81 .
Gene names were converted from Ensembl v103 82 to HUGO gene symbols with biomaRt v2.50.3 83 . Redundant gene counts after name conversion were summed. Transcripts per million (TPM) were calculated in standard fashion using gene lengths calculated with FeatureCounts v1.6.2 84 . Raw counts were normalized with DESeq2 v1.34.0 85 using default and recommended parameters. Variance stabilizing transformation was performed on the normalized counts and used for principal component analysis (PCA) with PCAtools v2.10.0 86 . PCA was performed using the 10% most variant genes ( n = 5942) in the dataset. Differential gene expression by UMIS level was performed without any adjustment parameters with default and recommended settings.
Enrichment scores of gene sets were calculated with singscore v1.14.0 18 using TPM input. Calculations utilized the unidirectional expected-upregulated mode, with the exception of the immune resistance program score 33 which was calculated using the bidirectional mode using separate expected-upregulated and expected-downregulated gene sets. UMIS was calculated with singscore using the unidirectional expected-upregulated mode using with the 2394 genes that positively correlated with immune and inflammatory hallmark gene set enrichment (negative PC2 gene loading) (Supplementary Data 4 ). A cohort-dependent version of UMIS was also calculated using gene set variation analysis (GSVA) with GSVA v1.42.0 87 using default settings and the same list of genes (Supplementary Data 4 ). This was only done for the purposes of comparison to the cohort-independent implementation with singscore and was not used elsewhere. Functional annotation of genes within UMIS ( n = 2394) was performed with the Database for Annotation, Visualization and Integrated Discovery (DAVID) online tool (accessed March 20th, 2022) 88 after filtering for protein coding genes using Human Genome Organization (HUGO) Gene Nomenclature Committee (HGNC) complete set annotation (accessed October 6th, 2021). Similarly, functional annotation of differentially expressed genes between UMIS levels was performed with clusterProfiler v4.2.2 89 using the fgsea v3.16 method on only protein-coding genes using HGNC complete set annotation (accessed October 6th, 2021). Correlation and clustering analysis of PCs used the Human Molecular Signatures Database Hallmark gene set collection 90 while functional annotation used the Human Molecular Signatures Database Gene Ontology Biological Process gene set collection 91 .
Human leukocyte antigen (HLA) typing of patients was performed using tumor bulk total RNAseq data. The arcasHLA v0.5.0 92 package was run with default settings to produce an output of genotypes for samples. Representative data for patients with multiple tumor samples was selected using the same algorithm as described previously in the survival analysis. TCR repertoires of tumors were analyzed from bulk total RNAseq data using MiXCR v3.0.12 93 with allowPartialAlignments=true as recommended for bulk RNAseq data. Counts were tabulated per amino acid CDR3 clonotype and used to calculate diversity (Shannon index) and clonality (1–Pielou’s index) for TRB and TRA chains 42 . Samples with one or zero detected unique clonotypes were excluded from diversity and clonality analysis due to mathematically undefinable clonality; this resulted in exclusion of 12 metastases from TRB analysis and 18 metastases from TRA analysis. Public versus private repertoire analysis was performed using immunarch v0.6.9 94 .
Targeted TCR repertoire library preparation, sequencing, and analysis
Targeted TCR repertoires of paired tumors and post-REP TIL were derived from respective total RNA. Libraries were prepared using the QIAseq Immune Repertoire RNA Library Kit (Qiagen) per manufacturer’s instructions. Libraries underwent quality check using a Standard Sensitivity NGS Fragment Analyzer Kit (Agilent) and quantification by qPCR with the Kapa qPCR quantification kit (Kapa Biosystems). The libraries were normalized, pooled, and sequenced using the MiSeq platform (Illumina) to an average of ~2.5 million 251 base pair paired end reads. The sequencing data was demultiplexed with bcl2fastq2 v2.20 (Illumina) to produce the fastq files. Sequencing data was processed using the Qiagen Biomedical Genomics Analysis 23.0 (Qiagen) per default recommended settings. Counts were tabulated from output files per amino acid CDR3 clonotype and used to calculate diversity (Shannon index) and clonality (1–Pielou’s index) for TRB and TRA chains.
Single cell RNA sequencing library preparation and sequencing
Selected tumors and previous treatments were UM #72 (tebentafusp), 83 (ICI and tebentafusp), 100 (ICI), 46 (liver directed therapy), 79 (cytotoxic chemotherapy and ICI), and 80 (liver directed therapy, kinase inhibition, antiangiogenic therapy and ICI). Cryopreserved single cell suspensions of selected tumors were prepared for input into the Chromium Next GEM Single Cell 5’ Reagent Kit v2 (10X Genomics) by thawing in complete media with human AB serum, sequential filtration through 70 mm and 30 mm MACS SmartStrainers (Miltenyi Biotec) and removal of dead cells using the Dead Cell Removal Kit (Miltenyi Biotec) per manufacturer’s protocol. Cell suspensions were inspected to confirm adequate viability (≥70%). Each tumor sample was processed in a separate lane of the Chip K, with ~35,000 cells loaded per sample. The 5’ gene expression and TCR V(D)J libraries were then prepared per manufacturer’s instructions. Prior to sequencing, libraries underwent quality check using a Standard Sensitivity NGS Fragment Analyzer Kit (Agilent) and quantification by qPCR with the Kapa qPCR quantification kit (Kapa Biosystems). The libraries were normalized, pooled, and sequenced using the NovaSeq6000 platform (Illumina) to an average of ~30,000 paired-end reads per 5’ gene expression library per cell and ~5000 paired-end reads per TCR V(D)J library per cell with parameters per manufacturer’s protocol. The sequencing data was demultiplexed with bcl2fastq2 v2.20 (Illumina) to produce the fastq files.
Single cell RNA sequencing computational processing
Sequencing data was processed using 10X Genomics Cell Ranger multi v6.1.2 95 using 10X Genomics Cloud Analysis with introns excluded and an estimated expected cell count of 20,000. Bioinformatic processing of each sample involved adjustment for ambient RNA contamination with SoupX v1.5.2 96 (default settings), normalization with the sctransform v2 method within Seurat 4.1.1 97 , 98 (default settings), and estimation and removal of doublets with DoubletFinder v2.0.3 99 (default settings). Cells remaining after quality control and removal of doublets were then input into our cataloging algorithm. This involved first assigning cells to large buckets using UCell v2.1.0 20 in the following order: immune (UCell score >0 for gene set of PTPRC ), tumor (UCell score >0 for gene set of SOX10, S100A1, MITF, MLANA, PMEL, TYR ), stroma (all remaining cells). A TIL atlas was created using a published dataset 19 with harmony v0.1.0 100 and symphony v0.1.0 21 using settings appropriate for the normalization method of the published dataset. Our samples’ immune fractions were then mapped onto the atlas using symphony settings recommended for data normalized with sctransform v2. The higher resolution “level 2” annotation, which included 31 phenotypes, was utilized. The addition of tumor and stroma cells to these immune cells completed our cellular cataloging and various levels (overall, broad, granular) were also assigned to the cells (Supplementary Data 9 ). For pooled analysis, samples were integrated with Seurat 4.1.1 using settings appropriate for sctransform v2-normalized data. Dimensionality reduction and differential gene expression were performed on the integrated Seurat object. Counts of specific cell types were derived from this integrated Seurat object. Comparison of cell type proportions by UMIS level was performed with the propeller function within the speckle v0.0.3 101 package using the arcsin square root transformation of proportions method.
TCR V(D)J repertoires were filtered for most frequent TRA and TRB chains using scRepertoire v1.4.0 102 . Counts were tabulated for cells with paired TRA and TRB chains per unique TRA-TRB amino acid CDR3 clonotype and used to calculate diversity (Shannon index) and clonality (1–Pielou’s index).
Statistical analysis
Statistics were calculated using R v4.1.2 103 (R Core Team) with RStudio v.2022.12.0 + 353 104 (Rstudio Team) or GraphPad Prism v9.5.0 (GraphPad Software), and specific statistical analyses used are highlighted in the respective figure legends. In general, continuous-continuous associations were assessed with the Spearman’s rank correlation with simple linear regression with 95% confidence intervals only to illustrate linearity. Unpaired categorical-continuous associations were assessed with the Wilcoxon rank-sum test (two-tailed) or Kruskal–Wallis one-way analysis of variance test as appropriate. Paired categorical-continuous associations were assessed with the Wilcoxon signed-rank test (two-tailed). Categorical-categorical associations were assessed with the Fisher’s exact test. Receiver operating characteristic curves were generated using univariate logistic regression and mapping of true positive 1 − specificity versus sensitivity. Areas under the ROC curves were calculated using the trapezoid rule. Time-to-event curves using the Kaplan–Meier method were generated with survminer v0.4.9 105 and comparisons between categorical groups were assessed with the logrank test. Clustering analysis was performed with ComplexHeatmap v2.10.0 106 and used the default method of Euclidean distance. Where appropriate, multiple comparison adjustment was performed with the false discovery rate (FDR) method using the p.adjust function with method = “fdr” in R. In R the lowest possible numeric value is roughly 1e−324. Thus, we presented values less than 1e−324 as ~0 rather than listing arbitrary lower limit numbers.
Utility visualization software
Aside from software previously mentioned, the following were used for various visualizations throughout the manuscript: tidyverse v1.3.2 107 , ggplot2 v3.4 108 , RColorBrewer v1.1-3 109 , ggprism v1.0.4 110 , patchwork v1.1.2 111 , packcircles v0.3.4 112 , plotly v4.10.0.9001. Illustrations were created with BioRender.com.
Reporting summary
Further information on research design is available in the Nature Portfolio Reporting Summary linked to this article.
Data availability
Bulk total RNAseq raw sequencing data generated in this study have been deposited in the database of Genotypes and Phenotypes (dbGaP) under accession number phs003330.v1.p1 [ https://www.ncbi.nlm.nih.gov/projects/gap/cgi-bin/study.cgi?study_id=phs003330.v1.p1 ]. These data are available under restricted access for patient confidentiality reasons and access can be obtained by request via the dbGaP system by following the instructions provided by the website. Approval is determined by the National Cancer Institute Data Access Committee, which can be emailed at [email protected]. Access to data is generally granted within a month of successful application and available indefinitely thereafter. Selected raw data are protected and are not publicly available due to data privacy laws but may be shared upon request. Source data are provided with this paper.
Code availability
Software packages were implemented as described in the “Methods” and no custom packages were created.
Hodi, F. S. et al. Improved survival with ipilimumab in patients with metastatic melanoma. N. Engl. J. Med. 363 , 711–723 (2010).
Article CAS PubMed PubMed Central Google Scholar
Larkin, J. et al. Five-year survival with combined nivolumab and ipilimumab in advanced melanoma. N. Engl. J. Med. 381 , 1535–1546 (2019).
Article CAS PubMed Google Scholar
Samstein, R. M. et al. Tumor mutational load predicts survival after immunotherapy across multiple cancer types. Nat. Genet. 51 , 202–206 (2019).
Yarchoan, M., Hopkins, A. & Jaffee, E. M. Tumor mutational burden and response rate to PD-1 inhibition. N. Engl. J. Med. 377 , 2500–2501 (2017).
Article PubMed PubMed Central Google Scholar
Tawbi, H. A. et al. Relatlimab and nivolumab versus nivolumab in untreated advanced melanoma. N. Engl. J. Med. 386 , 24–34 (2022).
Kalbasi, A. & Ribas, A. Tumour-intrinsic resistance to immune checkpoint blockade. Nat. Rev. Immunol. 20 , 25–39 (2020).
Algazi, A. P. et al. Clinical outcomes in metastatic uveal melanoma treated with PD-1 and PD-L1 antibodies. Cancer 122 , 3344–3353 (2016).
Piulats, J. M. et al. Nivolumab plus ipilimumab for treatment-naïve metastatic uveal melanoma: an open-label, multicenter, phase II trial by the Spanish Multidisciplinary Melanoma Group (GEM-1402). J. Clin. Oncol. 39 , 586–598 (2021).
Jager, M. J. et al. Uveal melanoma. Nat. Rev. Dis. Primers 6 , 24 (2020).
Article PubMed Google Scholar
Singh, A. D., Turell, M. E. & Topham, A. K. Uveal melanoma: trends in incidence, treatment, and survival. Ophthalmology 118 , 1881–1885 (2011).
Niederkorn, J. Y. Ocular immune privilege and ocular melanoma: parallel universes or immunological plagiarism? Front. Immunol. 3 , 148 (2012).
Carvajal, R. D. et al. Clinical and molecular response to tebentafusp in previously treated patients with metastatic uveal melanoma: a phase 2 trial. Nat. Med. 28 , 2364–2373 (2022).
Nathan, P. et al. Overall survival benefit with tebentafusp in metastatic uveal melanoma. N. Engl. J. Med. 385 , 1196–1206 (2021).
Rothermel, L. D. et al. Identification of an immunogenic subset of metastatic uveal melanoma. Clin. Cancer Res. 22 , 2237–2249 (2016).
Chandran, S. S. et al. Treatment of metastatic uveal melanoma with adoptive transfer of tumour-infiltrating lymphocytes: a single-centre, two-stage, single-arm, phase 2 study. Lancet Oncol. 18 , 792–802 (2017).
Crompton, J. G., Klemen, N. & Kammula, U. S. Metastasectomy for tumor-infiltrating lymphocytes: an emerging operative indication in surgical oncology. Ann. Surg. Oncol. 25 , 565–572 (2018).
Javed, A. et al. PD-L1 expression in tumor metastasis is different between uveal melanoma and cutaneous melanoma. Immunotherapy 9 , 1323–1330 (2017).
Foroutan, M. et al. Single sample scoring of molecular phenotypes. BMC Bioinform. 19 , 404 (2018).
Article CAS Google Scholar
Nieto, P. et al. A single-cell tumor immune atlas for precision oncology. Genome Res. 31 , 1913–1926 (2021).
Andreatta, M. & Carmona, S. J. UCell: robust and scalable single-cell gene signature scoring. Comput. Struct. Biotechnol. J. 19 , 3796–3798 (2021).
Kang, J. B. et al. Efficient and precise single-cell reference atlas mapping with Symphony. Nat. Commun. 12 , 5890 (2021).
Article ADS CAS PubMed PubMed Central Google Scholar
Smith-Garvin, J. E., Koretzky, G. A. & Jordan, M. S. T cell activation. Annu. Rev. Immunol. 27 , 591–619 (2009).
Ivashkiv, L. B. IFNγ: signalling, epigenetics and roles in immunity, metabolism, disease and cancer immunotherapy. Nat. Rev. Immunol. 18 , 545–558 (2018).
Dersh, D., Hollý, J. & Yewdell, J. W. A few good peptides: MHC class I-based cancer immunosurveillance and immunoevasion. Nat. Rev. Immunol. 21 , 116–128 (2021).
Diamond, M. S. & Farzan, M. The broad-spectrum antiviral functions of IFIT and IFITM proteins. Nat. Rev. Immunol. 13 , 46–57 (2013).
Chandran, S. S. et al. Tumor-specific effector CD8+ T cells that can establish immunological memory in humans after adoptive transfer are marked by expression of IL7 receptor and c-myc. Cancer Res. 75 , 3216–3226 (2015).
Krishna, S. et al. Stem-like CD8 T cells mediate response of adoptive cell immunotherapy against human cancer. Science 370 , 1328–1334 (2020).
Chow, M. T. et al. Intratumoral activity of the CXCR3 chemokine system is required for the efficacy of anti-PD-1 therapy. Immunity 50 , 1498–1512.e5 (2019).
Chen, J. et al. SLAMF7 is critical for phagocytosis of haematopoietic tumour cells via Mac-1 integrin. Nature 544 , 493–497 (2017).
Spranger, S., Bao, R. & Gajewski, T. F. Melanoma-intrinsic β-catenin signalling prevents anti-tumour immunity. Nature 523 , 231–235 (2015).
Article ADS CAS PubMed Google Scholar
Duraiswamy, J. et al. Myeloid antigen-presenting cell niches sustain antitumor T cells and license PD-1 blockade via CD28 costimulation. Cancer Cell 39 , 1623–1642.e20 (2021).
Luke, J. J., Bao, R., Sweis, R. F., Spranger, S. & Gajewski, T. F. WNT/β-catenin pathway activation correlates with immune exclusion across human cancers. Clin Cancer Res. 25 , 3074–3083 (2019).
Jerby-Arnon, L. et al. A cancer cell program promotes T cell exclusion and resistance to checkpoint blockade. Cell 175 , 984–997.e24 (2018).
Yu, F. et al. Loss of lncRNA-SNHG7 promotes the suppression of hepatic stellate cell activation via miR-378a-3p and DVL2. Mol. Ther. Nucleic Acids 17 , 235–244 (2019).
Chen, Y. et al. Knockdown of lncRNA SNHG7 inhibited cell proliferation and migration in bladder cancer through activating Wnt/β-catenin pathway. Pathol. Res. Pract. 215 , 302–307 (2019).
Bian, Z. et al. The role of long noncoding RNA SNHG7 in human cancers (Review). Mol. Clin. Oncol. 13 , 45 (2020).
Najafi, S. et al. Oncogenic roles of small nucleolar RNA host gene 7 (SNHG7) long noncoding RNA in human cancers and potentials. Front. Cell Dev. Biol. 9 , 809345 (2021).
Ren, J. et al. Long noncoding RNA SNHG7 promotes the progression and growth of glioblastoma via inhibition of miR-5095. Biochem. Biophys. Res. Commun. 496 , 712–718 (2018).
Rooney, M. S., Shukla, S. A., Wu, C. J., Getz, G. & Hacohen, N. Molecular and genetic properties of tumors associated with local immune cytolytic activity. Cell 160 , 48–61 (2015).
Fehrenbacher, L. et al. Atezolizumab versus docetaxel for patients with previously treated non-small-cell lung cancer (POPLAR): a multicentre, open-label, phase 2 randomised controlled trial. Lancet 387 , 1837–1846 (2016).
Ayers, M. et al. IFN-γ-related mRNA profile predicts clinical response to PD-1 blockade. J. Clin. Invest. 127 , 2930–2940 (2017).
Chiffelle, J. et al. T-cell repertoire analysis and metrics of diversity and clonality. Curr. Opin. Biotechnol. 65 , 284–295 (2020).
Robertson, A. G. et al. Integrative analysis identifies four molecular and clinical subsets in uveal melanoma. Cancer Cell 32 , 204–220.e15 (2017).
Field, M. G. et al. Punctuated evolution of canonical genomic aberrations in uveal melanoma. Nat. Commun. 9 , 116 (2018).
Article ADS PubMed PubMed Central Google Scholar
Carvajal, R. D. et al. Advances in the clinical management of uveal melanoma. Nat. Rev. Clin. Oncol. 20 , 99–115 (2023).
Pelster, M. S. et al. Nivolumab and ipilimumab in metastatic uveal melanoma: results from a single-arm phase II study. J. Clin. Oncol. 39 , 599–607 (2021).
Karlsson, J. et al. Molecular profiling of driver events in metastatic uveal melanoma. Nat. Commun. 11 , 1894 (2020).
Shain, A. H. et al. The genetic evolution of metastatic uveal melanoma. Nat. Genet. 51 , 1123–1130 (2019).
Durante, M. A. et al. Single-cell analysis reveals new evolutionary complexity in uveal melanoma. Nat. Commun. 11 , 496 (2020).
Spranger, S., Dai, D., Horton, B. & Gajewski, T. F. Tumor-residing Batf3 dendritic cells are required for effector T cell trafficking and adoptive T cell therapy. Cancer Cell 31 , 711–723.e14 (2017).
Sharma, P., Hu-Lieskovan, S., Wargo, J. A. & Ribas, A. Primary, adaptive, and acquired resistance to cancer immunotherapy. Cell 168 , 707–723 (2017).
Wang, Y. et al. Multimodal single-cell and whole-genome sequencing of small, frozen clinical specimens. Nat. Genet. 55 , 19–25 (2023).
Bugter, J. M., Fenderico, N. & Maurice, M. M. Mutations and mechanisms of WNT pathway tumour suppressors in cancer. Nat. Rev. Cancer 21 , 5–21 (2021).
Mariani, P. et al. Immunohistochemical characterisation of the immune landscape in primary uveal melanoma and liver metastases. Br. J. Cancer 129 , 772–781 (2023).
Valpione, S. et al. The T cell receptor repertoire of tumor infiltrating T cells is predictive and prognostic for cancer survival. Nat. Commun. 12 , 4098 (2021).
Yusko, E. et al. Association of tumor microenvironment T-cell repertoire and mutational load with clinical outcome after sequential checkpoint blockade in melanoma. Cancer Immunol. Res. 7 , 458–465 (2019).
Riaz, N. et al. Tumor and microenvironment evolution during immunotherapy with nivolumab. Cell 171 , 934–949.e16 (2017).
Tumeh, P. C. et al. PD-1 blockade induces responses by inhibiting adaptive immune resistance. Nature 515 , 568–571 (2014).
Hanada, K. I. et al. A phenotypic signature that identifies neoantigen-reactive T cells in fresh human lung cancers. Cancer Cell 40 , 479–493.e6 (2022).
Lowery, F. J. et al. Molecular signatures of antitumor neoantigen-reactive T cells from metastatic human cancers. Science 375 , 877–884 (2022).
Eisenhauer, E. A. et al. New response evaluation criteria in solid tumours: revised RECIST guideline (version 1.1). Eur. J. Cancer 45 , 228–247 (2009).
Kendig, K. I. et al. Sentieon DNASeq variant calling workflow demonstrates strong computational performance and accuracy. Front. Genet. 10 , 736 (2019).
Van der Auwera, G. A. et al. From FastQ data to high confidence variant calls: the Genome Analysis Toolkit best practices pipeline. Curr. Protoc. Bioinform. 43 , 11.10.11–11.10.33 (2013).
Google Scholar
Talevich, E., Shain, A. H., Botton, T. & Bastian, B. C. CNVkit: genome-wide copy number detection and visualization from targeted DNA sequencing. PLoS Comput. Biol. 12 , e1004873 (2016).
Abyzov, A., Urban, A. E., Snyder, M. & Gerstein, M. CNVnator: an approach to discover, genotype, and characterize typical and atypical CNVs from family and population genome sequencing. Genome Res. 21 , 974–984 (2011).
Chen, X. et al. Manta: rapid detection of structural variants and indels for germline and cancer sequencing applications. Bioinformatics 32 , 1220–1222 (2016).
Jeffares, D. C. et al. Transient structural variations have strong effects on quantitative traits and reproductive isolation in fission yeast. Nat. Commun. 8 , 14061 (2017).
Geoffroy, V. et al. AnnotSV: an integrated tool for structural variations annotation. Bioinformatics 34 , 3572–3574 (2018).
Kandoth, C. mskcc/vcf2maf: vcf2maf v1.6.19 (2020).
McLaren, W. et al. The Ensembl variant effect predictor. Genome Biol. 17 , 122 (2016).
Smigielski, E. M., Sirotkin, K., Ward, M. & Sherry, S. T. dbSNP: a database of single nucleotide polymorphisms. Nucleic Acids Res. 28 , 352–355 (2000).
Tate, J. G. et al. COSMIC: the Catalogue Of Somatic Mutations In Cancer. Nucleic Acids Res. 47 , D941–D947 (2019).
Mayakonda, A., Lin, D. C., Assenov, Y., Plass, C. & Koeffler, H. P. Maftools: efficient and comprehensive analysis of somatic variants in cancer. Genome Res. 28 , 1747–1756 (2018).
Paniccia, A. et al. Prospective, multi-institutional, real-time next-generation sequencing of pancreatic cyst fluid reveals diverse genomic alterations that improve the clinical management of pancreatic cysts. Gastroenterology 164 , 117–133.e17 (2023).
Andrews, S. FastQC: a quality control tool for high throughput sequence data (2010).
Martin, M. Cutadapt removes adapter sequences from high-throughput sequencing reads. EMBnet J. 17 , 10–12 (2011).
Kopylova, E., Noé, L. & Touzet, H. SortMeRNA: fast and accurate filtering of ribosomal RNAs in metatranscriptomic data. Bioinformatics 28 , 3211–3217 (2012).
Dobin, A. et al. STAR: ultrafast universal RNA-seq aligner. Bioinformatics 29 , 15–21 (2013).
Frankish, A. et al. GENCODE 2021. Nucleic Acids Res. 49 , D916–D923 (2021).
Li, H. et al. The sequence alignment/map format and SAMtools. Bioinformatics 25 , 2078–2079 (2009).
Anders, S., Pyl, P. T. & Huber, W. HTSeq—a Python framework to work with high-throughput sequencing data. Bioinformatics 31 , 166–169 (2015).
Cunningham, F. et al. Ensembl 2022. Nucleic Acids Res. 50 , D988–D995 (2022).
Smedley, D. et al. The BioMart community portal: an innovative alternative to large, centralized data repositories. Nucleic Acids Res. 43 , W589–598 (2015).
Liao, Y., Smyth, G. K. & Shi, W. featureCounts: an efficient general purpose program for assigning sequence reads to genomic features. Bioinformatics 30 , 923–930 (2014).
Love, M. I., Huber, W. & Anders, S. Moderated estimation of fold change and dispersion for RNA-seq data with DESeq2. Genome Biol. 15 , 550 (2014).
Blighe, K. PCAtools: PCAtools: Everything Principal Components Analysis. R package version 2.10.0 (2022).
Hänzelmann, S., Castelo, R. & Guinney, J. GSVA: gene set variation analysis for microarray and RNA-seq data. BMC Bioinform. 14 , 7 (2013).
Article Google Scholar
Sherman, B. T. et al. DAVID: a web server for functional enrichment analysis and functional annotation of gene lists (2021 update). Nucleic Acids Res. 50 , W216–221 (2022).
Wu, T. et al. clusterProfiler 4.0: a universal enrichment tool for interpreting omics data. Innovation 2 , 100141 (2021).
CAS PubMed PubMed Central Google Scholar
Liberzon, A. et al. The Molecular Signatures Database (MSigDB) hallmark gene set collection. Cell Syst. 1 , 417–425 (2015).
The Gene Ontology Consortium. The Gene Ontology Resource: 20 years and still GOing strong. Nucleic Acids Res . 47 , D330–D338 (2019).
Orenbuch, R. et al. arcasHLA: high-resolution HLA typing from RNAseq. Bioinformatics 36 , 33–40 (2020).
Bolotin, D. A. et al. MiXCR: software for comprehensive adaptive immunity profiling. Nat. Methods 12 , 380–381 (2015).
Nazarov, V. et al. immunarch: Bioinformatics Analysis of T-Cell and B-Cell Immune Repertoires R package version 1.0.0 (2022).
Zheng, G. X. et al. Massively parallel digital transcriptional profiling of single cells. Nat. Commun. 8 , 14049 (2017).
Young, M. D. & Behjati, S. SoupX removes ambient RNA contamination from droplet-based single-cell RNA sequencing data. Gigascience 9 , 12 (2020)
Choudhary, S. & Satija, R. Comparison and evaluation of statistical error models for scRNA-seq. Genome Biol. 23 , 27 (2022).
Hao, Y. et al. Integrated analysis of multimodal single-cell data. Cell 184 , 3573–3587.e29 (2021).
McGinnis, C. S., Murrow, L. M. & Gartner, Z. J. DoubletFinder: doublet detection in single-cell RNA sequencing data using artificial nearest neighbors. Cell Syst. 8 , 329–337.e4 (2019).
Korsunsky, I. et al. Fast, sensitive and accurate integration of single-cell data with harmony. Nat. Methods 16 , 1289–1296 (2019).
Phipson, B. et al. propeller: testing for differences in cell type proportions in single cell data. Bioinformatics 38 , 4720–4726 (2022).
Borcherding, N., Bormann, N. L. & Kraus, G. scRepertoire: an R-based toolkit for single-cell immune receptor analysis. F1000Res 9 , 47 (2020).
The R Development Core Team. R: A Language and Environment for Statistical Computing (2021).
RStudio Team. RStudio: Integrated Development for R (2020).
Kassambara, A. et al. survminer: Drawing Survival Curves using ‘ggplot2’. R package version 0.4.9 (2021).
Gu, Z., Eils, R. & Schlesner, M. Complex heatmaps reveal patterns and correlations in multidimensional genomic data. Bioinformatics 32 , 2847–2849 (2016).
Wickham, H. et al. Welcome to the tidyverse. J. Open Source Softw. 4 , 1686 (2019).
Wickham, H . ggplot2: Elegant Graphics for Data Analysis (Springer-Verlag, 2016).
Brewer, C. RColorBrewer: ColorBrewer Palettes. R package version 1.1-3 (2022).
Dawson, C. ggprism: A. ‘ggplot2’ Extension Inspired by ‘GraphPad Prism’. R package version 1.0.4 (2022).
Pedersen, T. patchwork: The Composer of Plots. R package version 1.1.2 (2022).
Bedward, M., Eppstein, D. & Menzel, P. packcircles: Circle Packing. R package version 0.3.5 (2022).
Download references
Acknowledgements
We thank the members of the UPMC clinical trial and research teams for their efforts in this study and the Surgery Branch, NCI, for providing selective tumor samples. We thank Clinigen for providing interleukin-2 for clinical and research studies and thank all the patients who participated in this study. This research was supported by the UPMC Immune Transplant and Therapy Center and in part by the University of Pittsburgh Center for Research Computing, RRID:SCR_022735, through the resources provided. Specifically, this work used the HTC cluster, which is supported by NIH award number S10OD028483. This work utilized the UPMC Hillman Cancer Center Immunologic Monitoring and Cellular Products Laboratory, a shared resource at the University of Pittsburgh supported by the CCSG P30 CA047904. S.L.-M. was supported by a National Institutes of Health National Cancer Institute training grant (T32CA113263).
Author information
Authors and affiliations.
UPMC Hillman Cancer Center, University of Pittsburgh, Pittsburgh, PA, USA
Shravan Leonard-Murali, Chetana Bhaskarla, Ghanshyam S. Yadav, Sudeep K. Maurya, Chenna R. Galiveti, Joshua A. Tobin, Rachel J. Kann, Eishan Ashwat, Patrick S. Murphy, Uma R. Chandran, Yen-Michael S. Hsu & Udai S. Kammula
Solid Tumor Cellular Immunotherapy Program, UPMC Hillman Cancer Center, University of Pittsburgh, Pittsburgh, PA, USA
Shravan Leonard-Murali, Chetana Bhaskarla, Ghanshyam S. Yadav, Sudeep K. Maurya, Chenna R. Galiveti, Joshua A. Tobin, Patrick S. Murphy & Udai S. Kammula
Division of Surgical Oncology, Department of Surgery, University of Pittsburgh, Pittsburgh, PA, USA
Shravan Leonard-Murali, Chetana Bhaskarla, Ghanshyam S. Yadav, Sudeep K. Maurya, Chenna R. Galiveti, Joshua A. Tobin & Udai S. Kammula
Department of Epidemiology, University of Pittsburgh, Pittsburgh, PA, USA
Shravan Leonard-Murali
Department of Biomedical Informatics, University of Pittsburgh, Pittsburgh, PA, USA
Anish B. Chakka, Vishal Soman, Paul G. Cantalupo & Uma R. Chandran
UPMC Genome Center, University of Pittsburgh, Pittsburgh, PA, USA
Xinming Zhuo, Gopi Vyas, Dara L. Kozak, Lindsey M. Kelly & Ed Smith
UPMC Immunologic Monitoring and Cellular Products Laboratory, University of Pittsburgh, Pittsburgh, PA, USA
Yen-Michael S. Hsu
Division of Hematology/Oncology, Department of Medicine, University of Pittsburgh, Pittsburgh, PA, USA
You can also search for this author in PubMed Google Scholar
Contributions
S.L.-M., C.B., G.Y., S.M., C.G., J.T., E.A. and P.M. carried out the experiments. S.L.-M., R.K. and U.K. analyzed data and prepared visualizations. S.L.-M., P.M., A.C., V.S., P.C. and U.C. designed the RNAseq bioinformatic pipeline. S.L.-M., P.M., E.S., G.V., L.K., D.K. and X.Z. performed next-generation sequencing. U.K. conducted associated TIL ACT clinical trials. Y.-M.H. and U.K. supervised TIL manufacturing associated with TIL ACT clinical trials. S.L.-M. collected clinicogenomic patient and metastasis data. S.L.-M. and U.K. conceived the project, designed the experiments, and wrote the manuscript.
Corresponding author
Correspondence to Udai S. Kammula .
Ethics declarations
Competing interests.
The authors declare no competing interests.
Ethical approval
We have complied with all relevant ethical regulations in the conduct and reporting of this study.
Peer review
Peer review information.
Nature Communications thanks Duc-Hau Le, Yong-Chen Lu, Lars Ny and the other, anonymous, reviewer(s) for their contribution to the peer review of this work. A peer review file is available.
Additional information
Publisher’s note Springer Nature remains neutral with regard to jurisdictional claims in published maps and institutional affiliations.
Supplementary information
Supplementary figs., peer review file, description of additional supplementary files, supplementary data 1, supplementary data 2, supplementary data 3, supplementary data 4, supplementary data 5, supplementary data 6, supplementary data 7, supplementary data 8, supplementary data 9, supplementary data 10, supplementary data 11, supplementary data 12, supplementary data 13, supplementary data 14, supplementary data 15, supplementary data 16, supplementary data 17, supplementary data 18, reporting summary, source data, source data, rights and permissions.
Open Access This article is licensed under a Creative Commons Attribution 4.0 International License, which permits use, sharing, adaptation, distribution and reproduction in any medium or format, as long as you give appropriate credit to the original author(s) and the source, provide a link to the Creative Commons licence, and indicate if changes were made. The images or other third party material in this article are included in the article’s Creative Commons licence, unless indicated otherwise in a credit line to the material. If material is not included in the article’s Creative Commons licence and your intended use is not permitted by statutory regulation or exceeds the permitted use, you will need to obtain permission directly from the copyright holder. To view a copy of this licence, visit http://creativecommons.org/licenses/by/4.0/ .
Reprints and permissions
About this article
Cite this article.
Leonard-Murali, S., Bhaskarla, C., Yadav, G.S. et al. Uveal melanoma immunogenomics predict immunotherapy resistance and susceptibility. Nat Commun 15 , 2863 (2024). https://doi.org/10.1038/s41467-024-46906-4
Download citation
Received : 20 June 2023
Accepted : 08 March 2024
Published : 16 April 2024
DOI : https://doi.org/10.1038/s41467-024-46906-4
Share this article
Anyone you share the following link with will be able to read this content:
Sorry, a shareable link is not currently available for this article.
Provided by the Springer Nature SharedIt content-sharing initiative
By submitting a comment you agree to abide by our Terms and Community Guidelines . If you find something abusive or that does not comply with our terms or guidelines please flag it as inappropriate.
Quick links
- Explore articles by subject
- Guide to authors
- Editorial policies
Sign up for the Nature Briefing: Cancer newsletter — what matters in cancer research, free to your inbox weekly.

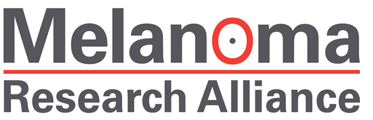
What’s Next in Melanoma Treatment?
By Cody Barnett, MPH, MRA Senior Director of Communications & Patient Engagement | 20 March 2018 | Science , Treatment

We are at an unprecedented moment in the field of melanoma. Since 2011, 11 new treatments have dramatically expanded the arsenal of FDA-approved treatment options. These advances have substantially improved the three-year survival rates for about half of patients with late-stage melanoma and given all patients hope for the future.
Despite these incredible advances, too many patients still aren’t benefiting from even the most recent advances. In fact, over 9,000 people in the U.S. alone will die due to melanoma this year. Thankfully, researchers are working around the clock on a variety of novel and iterative improvements that will move the needle further and bring us that much closer to a world without melanoma.
In a presentation given at MRA’s Patient and Foundations Forum held late last month, Dr. Douglas Johnson, MRA Young Investigator awardee and Assistant Professor of Medicine and Melanoma Clinical Director at the Vanderbilt-Ingram Cancer Center, outlined three broad questions that he said researchers are actively working toward answering:
- Why don’t some patients respond to treatment?
How can we enhance immunotherapy?
How can we overcome limitations in targeted therapy?
While the future of melanoma treatment won’t be unearthed by the answer to a single question, together they will move us towards our common goal of ending death and suffering due to melanoma.
Why don’t some people respond to immunotherapy?
The mantra for immunotherapy is that when it works, it really works. However, we don’t fully understand what makes people more (or less) likely to respond extremely well to these breakthrough treatments. Many researchers think that the secret to predicting how a patient will respond rests in the characteristics of the tumor, the patient’s immune system, and possibly (because results are still rather preliminary), in the patient’s gut microbiome (gut bacteria). Tumor factors, such as the number of mutations present in the cancer cells, are a big response predictor, with more mutated tumors typically being easier to treat. Another predictor is whether T cells, a type of white blood cell, are located in or near the tumor. Tumors with greater numbers of ‘infiltrating’ T cells are more likely to respond to immunotherapy. Finally, researchers aren’t yet sure what specific mechanisms are at play, but they believe that “good” bacteria in our microbiome work to prime the immune system and prepare it to better target and attack cancer.
If we can understand why some people do (or do not) respond to immunotherapy, we can then start to look at how to manipulate these factors in patients to extend the benefits of immunotherapy – and the durable responses it can provide – to more people.
In addition to improving the number of people who respond to immunotherapy, many researchers are examining how they can improve immunotherapy altogether by reducing the number and severity of side effects that patients face or by leveraging the power of immunotherapy to treat melanoma that has spread to the brain. To accomplish this, researchers are looking at novel ways to combine existing treatments and investigating altogether new drug targets and immunotherapy strategies.
Many researchers believe that by combining different drugs into a singular treatment approach, we can boost multiple parts of the immune system simultaneously and dramatically improve the effectiveness of the overall treatment. There are many clinical trials that are investigating this approach with a variety of agents.
One example of this kind of synergy is the FDA-approved immunotherapy combination of ipilimumab and nivolumab. These drugs target two different immune checkpoints, CTLA-4 (targeted by the drug ipilimumab ) and PD-1 (targeted by the drugs pembrolizumab and nivolumab ). For some patients, this combination produces better outcomes than either agent alone, but there is a distinct increase in severe adverse events. Researchers are working earnestly to identify new targets that could be used to further enhance the immune system’s ability to fight melanoma. Additional targets for immunotherapy also create the opportunity to develop additional combination therapies.
Most immunotherapy side effects are easily managed by medication, but severe adverse reactions are not uncommon. In fact, severe reactions occur in up to 20% of patients with some immunotherapy agents and when these drugs are used in combination, in over 50% (although even severe events typically resolve quickly with proper treatment). With immunotherapies in use in an increasing range of cancers, managing or otherwise mitigating these side effects, which can cause patients to temporarily or permanently stop treatment, is a high priority for future research and is currently being invested in through a unique partnership between MRA and the American Cancer Society. Learn more about this partnership here .
Researchers and clinicians to date have had only moderate success at treating melanoma that has spread to the brain. There is concerted effort to better understand brain metastases and to develop treatments that are able to effectively reach tumors in the brain. There is early evidence that the FDA-approved combination of nivolumab + ipilimumab and other investigational agents are effective in at least some patients. Due to the high number of patients with melanoma who develop brain metastasis – this is a high priority for future research.
About half of all melanoma cells have genetic mutations in the BRAF (pronounced bee-raf) protein. BRAF and MEK, another protein, are molecules that help regulate the way cells grow.
A BRAF mutation triggers cells to develop abnormally and divide out of control. Targeted therapy drugs block the activity of the MEK protein and the mutated BRAF protein. In this way, the drugs slow or stop the growth and spread of melanoma. Almost all metastatic melanoma patients with BRAF mutations see rapid improvement in symptoms and tumor shrinkage. Targeted therapies also carry fewer serious side effects than immunotherapies. The problem is that most patients will develop some type of resistance, meaning that over time melanoma can short circuit the medication and grow despite it. For most patients, acquired resistance develops in about nine to twelve months.
Researchers are actively working to create targeted therapies that last longer and work for more patients. One way researchers have found success is by combining a BRAF inhibitor with a MEK inhibitor – such as the FDA-approved dabrafenib + mekinist or vemurafenib + cobimetinib . The combined therapy works better, for longer, and with fewer serious side effects than either drug on its own. Researchers are working to find additional targets in order to create new combinations. Key to doing this is finding additional mutations that future drugs can be aimed at. Drugs targeting mutations in the NRAS protein are an important area being studied in clinical trials. Researchers are also examining if intermittent dosing – one week on and one week off (or some other schedule) – is effective at stopping or at least slowing targeted therapy resistance.
While Dr. Johnson framed his presentation with three key questions that clinicians and researchers alike are working towards answering, no one answer to any of these questions will move us into the future of melanoma treatment by itself. Instead, the future of melanoma treatment will be discovered by continually building on previous discoveries – just like standing on the shoulders of giants.
“Metastatic melanoma was previously a field without any effective treatment options,” said Dr. Johnson. “Through a concerted effort to understand the biology of cancer and the immune system, we now have numerous effective treatments. Continued partnerships between scientists and clinicians are necessary to move the bar higher and cure more patients.”
Learn more about treatment approaches for melanoma here . Learn more about clinical trials here .
I've lost my password
Sign Up for Emails
Annual progress reports, privacy statement.

An official website of the United States government
The .gov means it’s official. Federal government websites often end in .gov or .mil. Before sharing sensitive information, make sure you’re on a federal government site.
The site is secure. The https:// ensures that you are connecting to the official website and that any information you provide is encrypted and transmitted securely.
- Publications
- Account settings
Preview improvements coming to the PMC website in October 2024. Learn More or Try it out now .
- Advanced Search
- Journal List
- Cancers (Basel)
- PMC10417305

Recent Advances in Clinical Research for Skin Cancer Chemoprevention
1 Department of Pharmaceutical Sciences, College of Pharmacy, Western University of Health Sciences, Pomona, CA 91766, USA; [email protected] (R.T.); [email protected] (S.H.); ude.unretsew@neserdnab (B.A.); ude.unretsew@dihahsa (A.S.); ude.unretsew@gnawj (J.W.)
Samuel Hanoun
Bradley andresen, ayaz shahid, jeffrey wang, kristen m. kelly.
2 Department of Dermatology, University of California, Irvine, CA 92697, USA; ude.icu.sh@yllekmk
Frank L. Meyskens, Jr.
3 Departments of Medicine and Biological Chemistry, Chao Family Comprehensive Cancer Center, University of California, Irvine, CA 92868, USA; ude.icu.sh@eksyemlf
Simple Summary
Skin cancer is the most common cancer type in the United States and the world. Both non-melanoma and melanoma skin cancer show a clear association with overexposure to solar ultraviolet radiation. Chemoprevention is an appealing strategy to control the increasing rate of skin cancer. Since the target population for cancer chemoprevention is healthy individuals with high cancer risk, pharmacological agents that can be used for preventive purposes should be both effective and safe. The present review outlines the current state of skin cancer chemoprevention clinical trials, in terms of study populations, agents, outcomes (including cancer risk reduction), predictive biomarkers, and adverse reactions. The most studied agents include non-steroidal anti-inflammatory drugs, retinoids, 5-fluorouracil, and nicotinamide. The route of administration can be oral or topical. Since the trial outcomes for most of these agents are inconsistent, there is a need for additional research in this area.
Neoplasm arising from the keratinocytes or melanocytes in the skin is the most prevalent type of cancer in the United States and worldwide. Since ultraviolet (UV) radiation may be a causing factor for several types of skin cancer, effective strategies to manage skin cancer include preventive measures such as minimizing exposure to UV and applying sunscreens. However, the effect of sunscreen in reducing skin cancer incidence remains uncertain. An alternative approach to prevent skin cancer is chemoprevention, which is defined as using either natural products or synthetic compounds to inhibit, delay, or reverse the development of cancer. Preclinical studies have demonstrated the effectiveness of multiple pharmacological agents and dietary supplements. However, whether preclinical findings can be translated into clinical application is unknown. This review evaluates the state of recent clinical trials investigating chemopreventive agents focusing on skin cancer to compare the target populations, interventions, endpoints, and outcomes of these trials. The ClinicalTrials and PubMed databases were searched for their available literature using the key words “skin cancer” and “chemoprevention”. The objective of this review is to provide updated information on the effectiveness and side effects of promising chemopreventive agents in human subjects and to identify research gaps.
1. Introduction
Skin cancer is one of the most common types of malignancy in the United States and the world, with rising incidence [ 1 , 2 ]. It is estimated that one out of five Americans will develop skin cancer by the age of 70, with Caucasians displaying the highest incidence [ 3 ]. Non-melanoma skin cancer (NMSC), including basal cell carcinoma (BCC) and squamous cell carcinoma (SCC), is the most common. Merkel cell carcinoma (MCC) less common, while malignant melanoma is one of the types of skin cancer with a higher potential for mortality. Skin cells can undergo neoplastic transformation due to DNA damage caused by environmental factors like exposure to ultraviolet (UV) radiation from sunlight or artificial sources like tanning beds and sunlamps [ 3 ]. UV not only induces DNA damage, but also creates an inflammatory and immunosuppressive microenvironment in which premalignant cells may grow into tumors [ 4 ].
BCC is the most common form of skin cancer, with an estimated 3.6 million cases yearly in the United States [ 5 ]. BCC is an uncontrolled and abnormal growth of skin cells in the epidermis, specifically the basal cell layer ( Figure 1 ). BCCs are usually found on skin areas exposed to the sun, including the face, neck, ears, scalp, shoulders, and back. Besides UV radiation, risk factors for BCC include older age, male gender, fair skin, and outdoor careers [ 5 ]. Genetic mutations in the genes of the hedgehog pathway contribute to the majority of BCCs [ 5 ]. BCCs grow slowly, cause minimal damage, and rarely metastasize when detected and treated early. However, some lesions can be highly destructive if left untreated.

Risk factors for the most common types of skin cancer. BCC: basal cell carcinoma; SCC: squamous cell carcinoma.
SCC is the second most prevalent type of skin cancer, with an estimated annual occurrence of 1.8 million cases within the United States [ 6 ]. SCC is an uncontrolled and abnormal growth of squamous cells that are in the epidermis layer ( Figure 1 ). Like BCC, SCC is also found in similar places exposed to the sun, such as the face, ears, scalp, neck, and hands. SCCs share similar risk factors to BCC but with the addition of a weakened immune system, sun-sensitive conditions such as Xeroderma pigmentosum , skin precancers such as actinic keratosis (AK), and a history of human papillomavirus infection [ 7 ]. In particular, organ transplant recipients who receive long-term immunosuppressive treatment are at greater risk for skin cancer: for BCC, there is a 10-fold increased risk, while the risk of developing SCC is greater by 65–250 times [ 8 ]. Compared to BCC, SCC may grow relatively more rapidly and metastasize more quickly if not detected or treated early.
Melanoma or malignant melanoma develops from melanocytes, which are specialized cells responsible for producing pigment in the skin ( Figure 1 ). Melanoma is a potentially lethal form of skin cancer. Over the past few decades, the incidence of melanoma is increasing in a rate more than any other malignancy in the United States [ 9 ]. Unlike other types of skin cancer, melanoma can grow in existing moles or may develop in various skin regions, even in areas not typically exposed to the sunlight. Risk factors for melanoma include UV exposure, weakened immune system, atypical moles, fair skin, skin cancer history, and family history of melanoma [ 10 ].
A rare type of skin cancer is MCC, which is an aggressive neuroendocrine carcinoma of the skin. The risk factors for MCC include older age, fair skin, extensive UV exposure, history of multiple skin cancers, and chronic immunosuppression due to HIV or solid organ transplantation [ 11 ]. About 80% of MCC is caused by Merkel cell polyomavirus infection, while 20% is caused by UV-mediated skin damage.
Other than immunosuppressants, recently, concerns have been raised about increased skin cancer risks associated with some commonly prescribed drugs: tumor necrosis factor alpha inhibitors, angiotensin-receptor blockers, phosphodiesterase type 5 inhibitors, and 3-hydroxy-3-methylglutaryl coenzyme A (HMG-CoA)-reductase inhibitors [ 12 ]. These drugs may interact with UV radiation, leading to photosensitivity responses in susceptible individuals [ 13 ].
In contrast to many other types of cancers, skin cancers emerge on the surface of the body, are usually visible, and thus, can be detected early by regular skin examinations at home and by dermatologists. Although the majority cases of NMSC can be cured with surgical excision, due to the high incidence, treatment of these diseases leads to a huge burden on health care systems [ 14 ]. Aside from early detection, managing the known risk factors contributing to skin cancer development is important, e.g., using UV-protective strategies such as avoiding excessive sun exposure and applying sunscreen. However, the implementation of UV-protective strategies is inconsistent; there is inadequate evidence as to whether sunscreen use can reduce the risk of skin cancer [ 14 , 15 , 16 ]. Cancer prevention has become one area of priority in “The War on Cancer” and represents a recently renewed goal aiming for reducing the cancer death rate by at least 50% over the next 25 years [ 17 ]. Chemoprevention, which is defined as the use of natural products or pharmacological agents to inhibit, block, or reverse cancer initiation, promotion, and progression, has also been investigated for skin cancer prevention [ 18 , 19 ]. The number of preclinical studies on skin cancer chemoprevention has been growing. However, there are few clinical studies able to provide sufficient evidence for recommending the use of chemopreventive agents for high-risk individuals.
This review aims to examine the status of recent skin cancer chemoprevention clinical trials regarding target populations, interventions, mechanisms of action, biomarkers, and outcomes. The ClinicalTrials and PubMed databases were systematically searched to identify relevant trials. By investigating these trials, the present review provides updated efficacy and side effect data for promising chemopreventive agents and identifies critical research gaps.
A systematic search was conducted in the ClinicalTrials.gov database using the search terms “skin cancer” and “chemoprevention”. A total of 72 studies were identified, which were then filtered by registration date from 1995 to 2022, resulting in 65 studies. Exclusion criteria were then applied to eliminate studies involving other cancer types, resulting in a final analysis of 18 studies that were specific to skin cancer ( Table 1 ).
Search results from the ClinicalTrials.gov database.
NMSC: non-melanoma skin cancer; BCC: basal cell carcinoma; SCC: squamous cell carcinoma; AK: actinic keratosis; XP: Xeroderma pigmentosum ; DFMO: difluoromethylornithine; PUFA: polyunsaturated fatty acid; 5-FU: 5-fluorouracil.
The PubMed database was also searched using the keywords “skin cancer” and “chemoprevention”, which generated 115 results. Further filtering was performed to select only clinical trials and randomized controlled trials with a registration date from 2010 to 2023, resulting in 55 studies. Exclusion criteria were applied to eliminate studies involving arsenical skin lesions, colorectal, advanced premalignant lesions, lung cancer prevention, subcutaneous pocket infection, surgical site infection, prevention of surgical site infection, gynecological cancer, periocular actinic keratinocytes, breast cancer, second primary tumor, pre-engraftment bloodstream infection, and hepatocellular carcinoma. Studies that did not evaluate drug effects or adverse drug reactions (ADRs), such as those assessing quality of life or predictors of toxicity, were also excluded. The remaining studies were screened, resulting in 21 studies that were considered relevant to skin cancer chemoprevention ( Table 2 ).
PubMed database outcomes.
3. Cancer Types or Conditions of the Clinical Trials
The results of the clinical trial search reveal that most studies focused on NMSC, with a few studies specifically focusing on BCC or SCC. Some studies did not specify cancer types as they utilized precancerous biomarkers or focused on pharmacokinetics or intervention safety. Out of the 18 studies from the ClinicalTrials.gov site, 10 were centered on NMSC prevention, with one each focused on SCC, BCC, AK, and skin immunity, and four were unspecified. No study was focused on melanoma prevention.
The PubMed search results generated more outcomes than ClinicalTrials.gov, and similarly, most studies (10) targeted NMSC, followed by specified BCC (4) and melanoma (3). One study focused on examining how intervention impacted skin immunity. All studies retrieved from PubMed had explicitly stated the targeted conditions.
When the results from both ClinicalTrials.gov and PubMed were combined, NMSC was still the most widely studied cancer type. Fewer trials were conducted for melanoma. Skin immunity was also included as a condition, as it may be a predictor of future skin cancer risk.
4. Interventions
Many of the studies found in the ClinicalTrials and PubMed databases were focused on the efficacy of preventive interventions for skin cancers and the adverse effects associated with preventive agents. Most of the studies were conducted with monotherapy, and commonly tested therapeutic agents were nicotinamide, retinoids, non-retinoid topical agents, nonsteroidal anti-inflammatory agents (NSAIDs), and nicotinamide ( Table 3 ). The most frequently studied agent was oral nicotinamide 500 mg, with study durations ranging from 6 to 12 months. Topical 5-fluorouracil 5% strength and topical diclofenac 3% strength were the next most frequently studied agents, with study durations ranging from 2 to 4 weeks and 1 to 9 months, respectively. Celecoxib 200 mg twice daily and aspirin 81 to 100 mg were other agents that appeared multiple times in the search results. Statins, anti-diabetic medications, and dietary supplements were less commonly evaluated but were present in the filtered results ( Table 3 ).
Summary of chemopreventive agents identified from the ClinicalTrials and PubMed databases.
PAR: retinoic acid receptors.
Both topical and systemic (oral) agents were studied. Although a considerable number of studies showed notably positive outcomes, certain studies did not exhibit any significant positive results or were not published. The lack of significant results may suggest the need for a larger sample size, a longer duration of treatment, or a different combination of agents. Overall, further clinical research is required to confirm the effectiveness of these agents in skin cancer prevention and the identification of potential adverse effects.
5. Populations
In the search results from the PubMed and ClinicalTrials databases, different target populations were assessed, including healthy individuals and those with an increased risk of skin cancer. The latter included patients with actinic keratoses, a history of NMSC, as well as organ transplant recipients taking immunosuppressants. People at high risk for skin cancer also include patients with the hereditary conditions such as Xeroderma pigmentosum . Healthy individuals with sun-damaged skin were included in some trials. Due to the high-impact Veterans Affairs (VA) Keratinocyte Carcinoma Chemoprevention Trials (VAKCCT), elderly individuals with a history of NMSC have received the most attention in identified clinical studies. The higher incidence of skin cancer and the potential to gain benefit from preventive interventions may be reasons that the elderly with a history of NMSC or actinic keratoses have been the most studied populations. Immunosuppressed organ transplant recipients represent a special population that have increased risk for skin cancer, particularly NMSC. It is very common for patients to develop multiple NMSC [ 24 ]. Once the first cancer is diagnosed, the patient has a higher risk of developing additional cancer. In contrast, research has paid the least attention to healthy individuals. It is equally important to investigate chemoprevention in healthy individuals for agents with proven safety because the agents can apply to the prevention of future development of skin cancer and to supplement the use of sunscreen in general population.
6. Endpoints
A variety of outcomes were measured in the identified trials. The incidence or rate of NMSC was the primary endpoint. Another interesting endpoint that was evaluated in some trials was cost-effectiveness or the ability to save money by using a particular drug. With current research, cost-effectiveness studies may not be the most beneficial due to limited FDA-approved options. To enable early intervention and halt disease progression, crucial endpoints for future research should include biological and immune function markers indicative of future cancer development. Moreover, endpoints such as improved quality of life, symptom relief, and prevention of tumor regression demand further investigation.
7. ClinicalTrials.Gov Outcomes
Out of the 18 search results, 9 clinical trials have published their findings ( Table 1 ). For example, topical 5-fluorouracil (5-FU) reduced the risk of SCC for up to one year, but no benefit was observed for BCC ( {"type":"clinical-trial","attrs":{"text":"NCT00847912","term_id":"NCT00847912"}} NCT00847912 ) [ 25 ]. Oral celecoxib (200 mg) significantly reduced BCC burden ( {"type":"clinical-trial","attrs":{"text":"NCT00023621","term_id":"NCT00023621"}} NCT00023621 ) [ 20 ]. Another trial found that a low dose of eflornithine (or difluoromethylornithine, DFMO) effectively reduced skin biopsy nuclear abnormality in patients with AK ( {"type":"clinical-trial","attrs":{"text":"NCT00021294","term_id":"NCT00021294"}} NCT00021294 ) [ 26 ]. The results from some studies retrieved from the ClinicalTrials.gov database were insignificant, such as interventions with high-dose topical tretinoin and oral acitretin on NMSC ( Table 1 ). Some trials have not been completed or did not report data.
8. PubMed Database Outcomes
Table 2 lists 20 studies identified from the PubMed database, most of which were focused on agents with anti-inflammatory activities. These agents can be classified into chemotherapy, retinoids, NSAIDs, vitamins, dietary supplements, or agents used for other disorders that can be repurposed for cancer chemoprevention. Their proposed mechanisms of action are shown in Table 3 . These agents can be administered orally, topically, or both. The topical delivery provides advantages of higher skin targeting effects and fewer systemic side effects. For studies found in both ClinicalTrials and PubMed, the trials are only listed in Table 1 .
9. Examples of Chemoprevention Trials
9.1. nicotinamide.
Multiple studies evaluated nicotinamide, a form of vitamin B3 or niacin, as a preventative measure for skin cancer. The mechanisms of action for nicotinamide possibly involve increasing DNA repair by blocking UV-induced cellular ATP loss and reducing UV-induced immunosuppression [ 35 , 52 ]. Due to the chemopreventive effects of nicotinamide in preclinical models and earlier small-scale studies in human subjects, a phase III randomized controlled trial was conducted to assess the efficacy of nicotinamide as a chemopreventive agent for NMSC in the Oral Nicotinamide to Reduce Actinic Cancer (ONTRAC) trial, published in 2015 [ 32 ]. This trial, with a large sample size of 386 immunocompetent participants, was able to conclude statistically significant differences between nicotinamide use and placebo for decreasing rates of new-onset AK and NMSC [ 32 ]. Based on the evidence, nicotinamide is recommended by up to 76.9% of Mohs surgeons for NMSC prevention [ 53 ].
Later, at least two clinical trials evaluated the effects of oral nicotinamide in immunocompromised individuals. One phase II randomized controlled trial of nicotinamide was conducted to evaluate the skin cancer chemoprevention in renal transplant recipients [ 33 ]. This study could not conclude statistically significant results due to a small sample size ( n = 22), although they reported reductions in AKs in the nicotinamide group compared to the placebo group [ 33 ]. More recently, given nicotinamide’s potential activity against immunosuppression, the Oral Nicotinamide to Reduce Actinic Cancer after Transplant (ONTRANS) trial was conducted on solid-organ transplant recipients with a history of multiple NMSC who received nicotinamide for 12 months [ 34 ]. The incidence of NMSC was nearly identical in nicotinamide and placebo groups. Therefore, the use of nicotinamide as a preventative measure for NMSC produced negative results in immunosuppressed solid-organ transplant recipients [ 32 ]. Although most studies supported the safety of nicotinamide as a chemopreventive agent with little to no side effects at doses as high as three grams daily [ 32 ], in the ONTRAC trial, patients who received nicotinamide, compared to the patients who received placebo, had significantly more mucocutaneous infections (lip, mucosal, nail, skin, and wound infections, as well as paronychia and sinusitis) [ 54 ].
The potential efficacy of nicotinamide was also shown in individuals living in arsenic-contaminated areas based on preclinical studies [ 55 ].
Nicotinamide is a substrate of nicotinamide N-methyltransferase (NNMT), which catalyzes the N-methylation of nicotinamide and regulates its level. In the past two decades, NNMT has been shown involved in carcinogenesis and tumor progression, including skin cancer [ 56 ]. The interaction of NNMT and nicotinamide needs further investigations.
9.2. NSAIDs
Cyclooxygenase-2 (COX-2) and its metabolic product prostaglandin E 2 (PGE 2 ) can be induced by UV radiation and play important roles in skin inflammation and carcinogenesis [ 57 ]. Nonsteroidal anti-inflammatory drugs (NSAIDs) exhibit anti-inflammatory effects by inhibiting COX-2 selectively or non-selectively and inhibiting the production of PGE 2 and have been investigated in multiple trials. NSAIDs include celecoxib, diclofenac, etodolac, rofecoxib, ibuprofen, naproxen, indomethacin, MF-tricyclic, sulindac, piroxicam, and aspirin. Since the anti-inflammatory activity of NSAIDs is believed to be mediated by the inhibition of COX-2, while gastrointestinal toxicity is due to COX-1 inhibition, several COX-2 selective inhibitors, such as celecoxib, were developed to avoid gastrointestinal adverse reactions [ 58 ]. None of the clinical studies could confirm that NSAIDs have significant effects as skin cancer chemopreventive agents, but there were other findings to note. One study focused on NSAIDs’ effects on BCC chemoprevention in mice and humans that are carriers of the mutant PTCH1, a receptor of the hedgehog pathway [ 20 ]. Oral celecoxib, a selective COX-2 inhibitor, was given to the human participants at a dose of 200 mg twice daily. Although celecoxib showed a 75% decrease in BCC tumor burden in mice, in the human trial, the effects of oral celecoxib in reducing BCC burden in all subjects were insignificant. However, when considering only 60% of the patients with less severe diseases (<15 BCCs at study entry), celecoxib significantly reduced the BCC numbers and burden. In another study, research on celecoxib for the chemoprevention of NMSC was conducted [ 36 ]. Participants were randomized and began treatment with either celecoxib or placebo and then evaluated at 3, 6, 9, and 11 months after randomization. No difference in AKs was found at nine months of treatment, but at 11 months, there was a significant reduction in the mean NMSCs per patient in the celecoxib group. After adjusting for age, sex, skin cancer history, etc., the results were still found to be statistically significant. However, the results were inconclusive due to the early termination of the study by the FDA based on another finding of an association between a COX-2 inhibitor and an increased risk of cardiovascular adverse events [ 36 ]. The efficacy results are consistent with previous observational studies, however, due to potential increased cardiovascular risks, celecoxib’s use as a chemopreventive agent could be limited.
A prospective cohort study examined the association between NSAIDs and NMSC in veterans with a higher risk of skin cancer [ 38 ]. The investigators hypothesized that NSAIDs and COX-2-selective inhibitors would provide transient protection against keratinocyte carcinoma, with COX-2-selective inhibitors having greater effects. The study participants were all from the Veterans Affairs Topical Tretinoin Chemoprevention Trial (VATTC), with 1131 veterans recruited. During a median follow-up time of 2 years for BCC and 2.5 years for SCC, 472 occurrences of BCC and 309 occurrences of SCC were observed. Time-fixed analyses and time-varying analyses were performed to avoid potential confounding bias. The time-fixed analyses produced a negative association but were determined not to be valid, and the time-varying analyses produced null results. Overall, this study did not prove a negative association between the use of NSAIDs and the risk of NMSC. The study concluded that the inverse dose response observed in the current study and in prior studies may be an artifact of analytic method.
Since regular use of the non-selective COX-1/COX-2 inhibitor aspirin (acetylsalicylic acid) has been associated with the risk reduction of multiple cancer types [ 57 ], the chemopreventive properties of aspirin were studied for skin cancer. One study tested the effect of aspirin on melanoma in elderly patients, but the results did not provide strong evidence that aspirin was associated with a reduced incidence of melanoma [ 37 ]. Another study examined the effects of aspirin alone or combined with folic acid in a randomized, double-blind, placebo-controlled clinical trial [ 39 ]. A total of 1121 patients were enrolled in the trial of prevention of colorectal adenomas, which was repurposed for BCC. BCC was confirmed by a blinded review of the pathology reports. Although aspirin and folic acid failed to show statistically significant effects on reducing the risk of BCC, subgroup analysis indicates that BCC risk was lower with aspirin use in those with previous skin cancer, while folic acid was unrelated to BCC incidence. Consistently, a retrospective study (2010–2018) conducted using the Humana Health Insurance database concluded that aspirin use was associated with a significantly decreased risk of BCC [ 59 ]. Therefore, given the high incidence and cost of BCC treatment, the low cost of aspirin and its widely accepted use may promote its preventive use for this type or other types of skin cancer.
Given the systemic adverse effects observed for most NSAIDs, topical delivery has been investigated for achieving a local preventive activity. Diclofenac sodium 3%, in combination with hyaluronic acid 2.5% (diclofenac 3%/HA 2.5%; Solaraze ® , Fougera Pharms) is the only NSAID approved in the United States for the topical treatment of AK lesions (for review, see [ 60 ]). The topical Solaraze ® proved to be effective for patients with existing AK. It is well tolerated, with skin irritation as the main side effect [ 57 ]. Another topical COX-2 inhibitor is piroxicam, which is a nonspecific COX-1 and COX-2 inhibitor, with higher inhibitory activity (10-fold) for COX-1. Finally, 1% piroxicam gel, topically applied daily for 12 weeks, was shown to effectively induce the complete regression of 48% of evaluated AKs, with an adverse effect of only skin irritation [ 40 ]. Thus, the topical application of NSAIDs is promising for providing cancer preventive efficacy with minor side effects.
9.3. Retinoids
Since the retinoid signaling pathway plays an important role in organ homeostasis and carcinogenesis, the natural and synthetic vitamin A derivatives, retinoids, may be effective for the prevention and the treatment of several types of cancer, including skin cancer (for review, see [ 61 ]). Initially, oral retinoids demonstrated efficacy as chemopreventive agents against NMSC and other types of cancer [ 24 , 48 ]. Therefore, there was a trend during the 1960s and 1970s to develop synthetic retinoids for cancer prevention and treatment [ 62 ]. Oral isotretinoin, acitretin, and etretinate have been reported to reduce BCCs in patients with Xeroderma pigmentosum , organ transplantation recipients, and individuals with basal cell nevus (Gorlin) syndrome (BCNS) [ 48 ]. However, the protective effects were lost after the therapy was discontinued. Lower doses with fewer side effects were ineffective [ 24 ]. Long-term and high-dose use of systemic retinoids has been associated with significant dose-dependent side effects [ 62 ]. For example, published in 2012, systematic use of acitretin for 2 years in nontransplantation patients at high risk for NMSC did not show statistically significant reduction in the rate of new NMSC, while the patients who received acitretin reported significantly more mucositis and skin toxicities compared to the placebo group [ 21 ]. Therefore, systematic use of retinoids is limited in the general population with no or few skin cancers.
Topical use of retinoids, e.g., tretinoin, has been used for decades for the treatment of acne and photoaging, without systematic side effects [ 24 ]. Thus, topical retinoids have been investigated in the Veterans Affairs Topical Tretinoin Chemoprevention (VATTC) Trial [ 24 ]. In this trial, 1131 patients were given topical 0.1% tretinoin or a matching vehicle control for 1.5–5.5 years. Reported in 2012, the primary outcomes, the rates of new BCC and SCC, did not differ significantly for the treatment. The tretinoin group showed worse cutaneous symptoms. This trial concluded that in high-risk patients, high-dose topical tretinoin was ineffective at reducing risk of NMSC.
Tazarotene (Tazorac ® , Allergan, Irvine, CA) is a topical retinoid with relative specificity for retinoic acid receptor (RAR)-β and RAR-γ receptors. A randomized, double-blind, vehicle-controlled study in patients with basal cell naevus syndrome (BCNS) evaluated the efficacy of topically applied tazarotene for BCC chemoprevention ( n = 34 subjects), along with an open-label trial evaluating tazarotene’s efficacy for chemotherapy of BCC lesions ( n = 36 subjects) for a maximum follow-up period of 3 years. Only 6% of patients had a chemopreventive response, and only 6% of treated BCC target lesions were clinically cured. Thus, this study provides no evidence for either chemopreventive or chemotherapeutic effect of tazarotene against BCCs in patients with BCNS. Therefore, despite the robust effects of topical retinoids in preclinical studies, they failed to demonstrate the effects in clinical studies.
9.4. 5-Fluorouracil (5-FU)
5-FU is a chemotherapy agent that belongs to a class of antimetabolites. It is used topically to treat skin cancer and AK. An earlier study that is frequently referenced in prevalent skin cancer publications is Predictors of squamous cell carcinoma in high-risk patients in the VATTC trial [ 41 ]. The study followed participants, mostly men with a median age of 72 with a history of heavily sun damaged skin. The subjects were required to have at least two forms of NMSC in the five years prior to their enrollment and had a follow up period of approximately four years. The purpose of this study was to find alternative preventive measures other than systemic retinoids, which have significant toxicity. A total of 1131 participants were screened, and of those participants, 23% developed at least one new SCC. The most important predictors of new SCC were identified as the number of prior carcinomas, the number of prior in situ carcinomas, the number of AKs prior to the study, the amount of sun exposure, and a history of 5-FU use. Participants that fell in the category of having many predictors had a significantly higher hazard ratio than those in the category of least predictors. Through a univariable analysis, all predictors were found to be statistically significant, while total sun exposure was found to have a greater association with newly developed SCC. The study concluded that a history of 5-FU use was strongly associated with an increased risk of future SCCs. On the other hand, using angiotensin-converting enzyme inhibitors or angiotensin receptor blockers reduced risk of SCC development. Furthermore, the study concluded that the administration of high-dose topical tretinoin was ineffective in diminishing the risk of NMSC.
Topical 5-FU (5%) has been investigated in multiple clinical trials of skin cancer chemoprevention, and most produced positive results. In the randomized Veterans Affairs Keratinocyte Carcinoma Chemoprevention (VAKCC) Trial of 932 veterans at high risk for NMSC, a 2–4-week duration of topical 5-FU reduced the risk of SCC for 1 year, but no effects were seen on BCC incidence in the first year [ 25 ]. There were no effects on SCC or BCC incidence at 4 years. Due to a potent chemopreventive effect in immunocompetent patients, a recent phase II open-label randomized controlled trial compared topical 5-FU, 5% imiquimod, and sunscreen in organ transplant recipients [ 45 ]. The pilot feasibility study suggested that topical 5-FU may be superior to imiquimod and sunscreen in AK clearance and prevention. Thus, 5-FU topical chemoprevention should be further investigated in SCC/AK prevention for immunocompromised patients.
The oral prodrug of 5-FU, capecitabine, was examined for the prevention and treatment of AK and NMSC. A systemic review indicated that capecitabine treatment may be associated with a decrease in the incidence of SCCs in organ transplant recipients [ 63 ]. ADRs, including fatigue, nausea, vomiting, diarrhea, elevated creatinine level, hand–foot syndrome, hyperuricemia, weight loss, anemia, and cardiomyopathy, limited the duration of chemoprevention in several patients.
9.5. Difluoromethylornithine (DFMO)
Difluoromethylornithine (DFMO), an inhibitor of ornithine decarboxylase, inhibits polyamine synthesis, which can be increased in UV-induced skin cancer [ 64 ]. A number of clinical trials have evaluated the effects of systemic DFMO, alone or in combination with other agents, for preventing skin cancer. Although the benefit of DFMO is promising, it has been reported to cause some side effects, such as hearing loss [ 64 ]. Therefore, topical application of DFMO is an option for reduced systemic effects. A previous study ( {"type":"clinical-trial","attrs":{"text":"NCT00021294","term_id":"NCT00021294"}} NCT00021294 ) investigated the efficacy of topical administration of DFMO (10%), triamcinolone (1%), and the combination of DFMO plus triamcinolone for the reduction of cell nuclear abnormality in moderately sun-damaged skin [ 26 ]. Eucerin ® (Beiersdorf Inc., Hamburg, Germany), a commercially available cream, was used as a vehicle. A total of 102 participants with sun-damaged skin on their posterolateral forearms were recruited for this study, and 185 skin biopsies were collected, with 16,395 nuclei recorded. High-resolution imagery of nuclei was utilized to assess the reduction of nuclei post-treatment and to compare them to baseline levels. Four treatment groups were established, including applying DFMO + Eucerin ® , DFMO + triamcinolone, triamcinolone + Eucerin ® , and Eucerin ® + Eucerin ® as a placebo. Participants applied 1 inch of cream to their forearms once daily throughout the study. The study found that applying these treatments resulted in a significant reduction in cell nuclear abnormality by 15–20%. These findings suggest that low-dose topical applications of DFMO, triamcinolone, and the combination of DFMO plus triamcinolone may effectively improve nuclear abnormality in moderately sun-damaged skin.
Since topical DFMO and topical diclofenac (an NSAID) as monotherapy have demonstrated chemopreventive activity against SCC, a phase IIB randomized trial of topical DFMO and diclofenac was conducted to evaluate the effects on sun-damaged skin in 136 patients who completed the study over three months. The goal was to examine whether the combination was more effective than monotherapy in reversing karyometric average nuclear abnormality, a predictor for SCC. However, the nuclear abnormalities increased in all three groups. The addition of topical DFMO to topical diclofenac did not enhance its activity but induced cutaneous inflammation as a side effect [ 27 ]. Based on this study, questions were raised regarding the efficacy of these agents, the status of cancer risk in the study population, and the validity of nuclear abnormalities as a marker [ 65 ].
9.6. Polyunsaturated Fatty Acids (PUFAs)
A study investigated the potential protective effect of the dietary supplement omega-3 polyunsaturated fatty acids (PUFAs) against photo-immunosuppression caused by solar UV radiation [ 30 ]. A total of 79 healthy female participants between the ages of 18 and 60 years were randomly assigned to either receive an oral placebo control lipid supplement or oral omega-3 PUFA (70% EPA and 10% DHA). The participants received nickel contact hypersensitivity patches to assess changes in photo-immunosuppression. After supplementation with either the control or PUFA, nickel was applied to the participants’ skin sites pre-exposed to three consecutive days of solar-simulated radiation (SSR) at a dose of 3.8 J/cm 3 and three unexposed control sites. The study found that omega-3 PUFAs were protective against photo-immunosuppression by lowering 50% of immunosuppression, measured by nickel contact hypersensitivity. The significance of this study lies in providing a potential solution to minimize skin damage caused by sunlight that can ultimately lead to skin cancer. This is particularly important because conventional sunscreens are inadequate in protecting against photo-immunosuppression compared to UV-induced erythema and are often misused or underutilized. Future well-designed human studies are needed to evaluate the effects of PUFAs.
9.7. Antidiabetic Drugs
Patients with type II diabetes show a higher risk of NMSC. These patients may benefit from the use of the commonly prescribed antidiabetic drug metformin to reduce the risk of NMSC and other types of cancer [ 47 ]. A secondary analysis of patients enrolled in the VAKCC trial was conducted to compare the risk for NMSC development between metformin users and non-users ( {"type":"clinical-trial","attrs":{"text":"NCT00847912","term_id":"NCT00847912"}} NCT00847912 ). Metformin-users had a significantly lower risk for SCC and BCC compared to non-users [ 66 ]. Another antidiabetic drug pioglitazone showed robust efficacy in preventing SCC in preclinical models [ 51 ], but no clinical trial data have been published yet. Future clinical trials are needed to answer the question of whether antidiabetic drugs are effective for skin cancer chemoprevention.
10. Safety Issues
Since the target population for cancer chemoprevention is healthy individuals with increased cancer risk, pharmacological agents that can be used for preventive purposes should be both effective and safe. Although the primary endpoints are usually efficacy, many clinical studies have reported side effects besides chemopreventive efficacy. Although some agents have demonstrated statistically significant efficacy, safety issues emerged after long-term and/or high-dose administration of these agents.
Among the chemopreventive interventions tested, trials using COX-2 inhibitors seemed to have the greatest risk to patients. Nearly all articles reported general adverse effects of these medications as well as serious cardiovascular effects. Common side effects associated with COX-2 inhibitors include infections, gastrointestinal disorders, musculoskeletal effects, and skin disorders. Cardiovascular effects included hypertension, myocardial infarction, stroke, congestive heart failure, or cardiovascular deaths. To better evaluate the safety profile, one trial performed a safety analysis that incorporated all randomly assigned patients that took at least one dose of the trial medication, placebo, or celecoxib [ 36 ]. The adverse events were compared by using either a chi-squared or Fisher exact test. Of the 183 subjects (87 in the celecoxib group and 96 in the placebo group), a total of 16 subjects reported experiencing severe adverse events, including 9 from the celecoxib group and 7 from the placebo group, with no deaths reported among all the subjects. The analysis concluded no significant differences between the placebo and the experimental groups.
Numerous studies have demonstrated toxicity associated with retinoids, particularly with systemic use [ 62 ]. Long-term retinoid therapy, especially at high doses, has been associated with teratogenicity [ 67 ], skeletal toxicity, such as the calcification of tendons and ligaments around joints, and hyperostosis of the spine, as well as osteoporosis [ 62 ]. Topical forms of retinoids can also induce toxicity, such as skin irritation. The safety issues may be related to a trend of less recent clinical trials on retinoids.
On the other hand, vitamins or dietary supplements, e.g., nicotinamide, are safe for long term use. Future studies should aim for proving the efficacy of these natural products.
11. Limitation of the Current Review
The limitations of this review include a narrow range of database searches using only two keywords. Additionally, this review could have included a more in-depth analysis of the studies’ methodologies to assess the quality of evidence. This review does not include intensive mechanisms of action or molecular targets for chemopreventive agents. Furthermore, this review’s focus on English-language studies may have resulted in the exclusion of relevant studies published in other languages.
12. Conclusions
This review provides an overview of the current state of the clinical research on the chemoprevention of skin cancer. While a significant proportion of the studies included in this review yielded positive efficacy results with statistical significance, the findings are not consistent when similar studies were conducted in different populations. For example, the results in the clinical trials for nicotinamide were not totally reproducible, and the data so far cannot provide sufficient evidence for drug efficacy due to doubts in the data analyses [ 68 ]. Further research is required to confirm the effectiveness of these agents in skin cancer prevention in various patient populations and to identify the complete profiles of adverse effects. The available evidence from multiple trials, including those found in the PubMed and ClinicalTrials databases, indicate that skin cancer chemoprevention is a largely under-researched area. Despite the high prevalence of skin cancer and the limited treatment options available, there has been a lack of attention given to this critical area of research, since cancer chemoprevention trials require relatively longer follow-up time compared to cancer treatment trials. Although certain skin cancer conditions, such as BCC, have been evaluated in several studies, others, including melanoma, have not received adequate attention. There is a significant need for future research studies to address the need for skin cancer chemoprevention particularly in subgroups of patients who are immunocompromised. Since systemic use of chemopreventive agents, such as COX-2 inhibitors and retinoids, can cause side effects, topical drug delivery shows advantages and should receive more attention. Systemic use of vitamins and dietary supplement should be a safer option. To address the safety issues of cancer chemopreventive agents, the effort should be directed to identify chemopreventive dietary supplements and to repurpose FDA-approved agents.
Funding Statement
This work was partly supported by the National Cancer Institute of the National Institutes of Health under the award number R01CA269653 (Y.H.). This work was also supported by the Western University of Health Sciences, College of Pharmacy, PharmD Student Summer Research Fellowship (R.T. and S.H.).
Author Contributions
Conceptualization, Y.H.; methodology, R.T. and S.H.; writing—original draft preparation, R.T. and S.H.; writing—review and editing, J.W., K.M.K., F.L.M.J. and B.A.; visualization, A.S.; supervision, Y.H.; funding acquisition, R.T., S.H. and Y.H. All authors have read and agreed to the published version of the manuscript.
Conflicts of Interest
The authors declare no conflict of interest.
Disclaimer/Publisher’s Note: The statements, opinions and data contained in all publications are solely those of the individual author(s) and contributor(s) and not of MDPI and/or the editor(s). MDPI and/or the editor(s) disclaim responsibility for any injury to people or property resulting from any ideas, methods, instructions or products referred to in the content.
- Skip to main content
- Skip to FDA Search
- Skip to in this section menu
- Skip to footer links

The .gov means it’s official. Federal government websites often end in .gov or .mil. Before sharing sensitive information, make sure you're on a federal government site.
The site is secure. The https:// ensures that you are connecting to the official website and that any information you provide is encrypted and transmitted securely.
U.S. Food and Drug Administration
- Search
- Menu
- FDA Organization
- Center for Drug Evaluation and Research | CDER
CDER Center for Clinical Trial Innovation (C3TI)
The Center for Drug Evaluation and Research (CDER) Center for Clinical Trial Innovation (C3TI) is a central hub that supports innovative approaches to clinical trials that are designed to improve the efficiency of drug development. C3TI aims to promote existing CDER programs and spur future clinical trial innovation activities through enhanced communication and collaboration.
C3TI will enable internal and external parties to access information on clinical trial innovation efforts more easily, engage in collaborations, identify resources that can further support the use of innovative modalities, and find development programs where a concerted approach to the use of clinical trial innovations would be impactful. The goals of these efforts are to help those involved in clinical research in staying current with clinical trial innovations, improve the efficiency and effectiveness of clinical trials, help increase the participation of diverse populations in clinical trials, and, in turn, accelerate the development of safe and effective new drugs.
C3TI is governed by leadership from the following CDER offices:
- Office of the Center Director
- Office of Communications
- Office of Compliance
- Office of Medical Policy
- Office of New Drugs
- Office of Translational Sciences
Connect with us
Featured C3TI Demonstrations & Activities

Video by CDER Center Director and Office of New Drugs leadership
Watch Dr. Patrizia Cavazzoni and Dr. Kevin Bugin share more about the vision and mission of C3TI
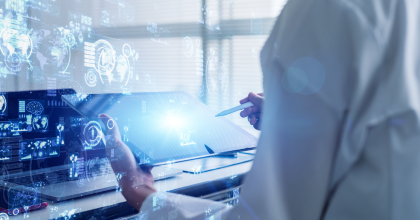
Demonstration Program
Learn about C3TI demonstration projects and how they provide the opportunity to test, implement, and scale the integration of innovation into clinical trials

Discover the core activities of C3TI established to enable and amplify innovative approaches to clinical trials

Frequently Asked Questions
See how C3TI impacts drug development, regulatory review, and sponsors’ interactions with CDER staff
Evidence Review of the Adverse Effects of COVID-19 Vaccination and Intramuscular Vaccine Administration
Vaccines are a public health success story, as they have prevented or lessened the effects of many infectious diseases. To address concerns around potential vaccine injuries, the Health Resources and Services Administration (HRSA) administers the Vaccine Injury Compensation Program (VICP) and the Countermeasures Injury Compensation Program (CICP), which provide compensation to those who assert that they were injured by routine vaccines or medical countermeasures, respectively. The National Academies of Sciences, Engineering, and Medicine have contributed to the scientific basis for VICP compensation decisions for decades.
HRSA asked the National Academies to convene an expert committee to review the epidemiological, clinical, and biological evidence about the relationship between COVID-19 vaccines and specific adverse events, as well as intramuscular administration of vaccines and shoulder injuries. This report outlines the committee findings and conclusions.
Read Full Description
- Digital Resource: Evidence Review of the Adverse Effects of COVID-19 Vaccination
- Digital Resource: Evidence Review of Shoulder Injuries from Intramuscular Administration of Vaccines
- Press Release
Recent News
NAS Launches Science and Innovation Fund for Ukraine
Science Academies Issue Statements to Inform G7 Talks
Supporting Family Caregivers in STEMM
A Vision for High-Quality Preschool for All
- Load More...
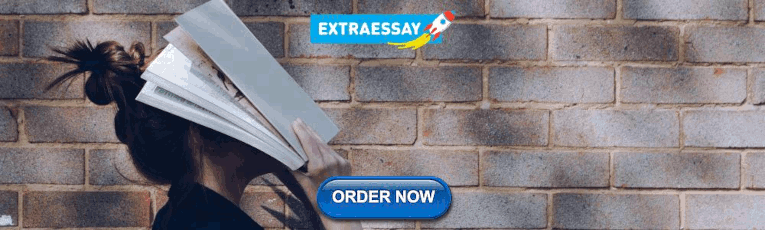
IMAGES
VIDEO
COMMENTS
The Skin SPORE program's main focus is on melanoma research activities, but it also includes projects in other skin cancer types, such as cutaneous T-cell lymphoma. NCI's National Clinical Trials Network (NCTN) is a collection of organizations and clinicians that coordinates and supports cancer clinical trials at more than 3,000 sites across ...
Retrospective comparison of a weight-based dose every 2 weeks with a fixed dose every month: a real-life analysis of nivolumab in the treatment of advanced melanoma, Melanoma Research, (2024 ...
The melanoma care team at Mayo Clinic includes surgical oncologists, medical oncologists, radiation oncologists, dermatologists, radiologists and pathologists. We work together and with other scientists to bring research advancements into care as quickly as possible. How has research advanced the treatment of early-stage melanoma in recent years?
Epidemiology. While the incidence and mortality of most cancers have declined over the past several decades, the incidence of melanoma continues to rise, particularly in the countries of fair-skinned populations of European descent. In 2020, the global burden of melanoma increased to 325,000 cases from 230,000 cases in 2012, a 41% increase ( 1 ).
In 2020, a grim milestone was reached when the global melanoma incidence passed 300,000 newly diagnosed cases in a single year 1. The most common form of this cancer, cutaneous melanoma, occurs on ...
2232 n engl j med 384;23 nejm.org June 10, 2021 The new england journal of medicine points, including cytotoxic T-lymphocyte antigen 4 (CTLA-4) and programmed death 1 (PD-1) pro-tein, pioneered by ...
Melanoma is the most serious type of skin cancer. It develops when skin cells multiply rapidly as a consequence of mutations in their DNA caused by UV exposure. Melanomas originate in the pigment ...
Melanoma is the most lethal type of skin cancer that originates from the malignant transformation of melanocytes. ... the ongoing clinical trials and future perspectives of clinical advances were ...
Although the incidence of primary cutaneous melanoma has steadily increased for several decades and remains the most lethal of the primary cutaneous neoplasms, the 3-year overall survival (OS) rates have remained relatively constant from 26.4% to as low as 4.7% across the subcategories of stage IV metastatic disease from 2004 to 2009. 1,2 As of 2018, the SEER database estimated that the 5-year ...
Abstract. Background: Early diagnosis is the key to improving outcomes for patients with melanoma, and this requires a standardized histological assessment approach. The objective of this survey was to understand the challenges faced by clinicians when assessing melanoma cases, and to provide a perspective for future studies.
mRNA vaccine for melanoma reduces relapse after therapy, trial finds. A personalized mRNA cancer vaccine combined with immunotherapy cuts the risk of recurrence by nearly half, researchers say. In ...
Abstract. The use of immunotherapy in the treatment of advanced and high-risk melanoma has led to a striking improvement in outcomes. Although the incidence of melanoma has continued to rise, median survival has improved from approximately 6 months to nearly 6 years for patients with advanced inoperable stage IV disease.
Artificial intelligence algorithms powered by deep learning improve skin cancer diagnostic accuracy for doctors, nurse practitioners and medical students in a study led by the Stanford Center for Digital Health. ... Linos, associate dean of research and the Ben Davenport and Lucy Zhang Professor in Medicine, is the senior author of the study ...
Abstract. Five years ago, the Melanoma Research Foundation (MRF) conducted an assessment of the challenges and opportunities facing the melanoma research community and patients with melanoma. Since then, remarkable progress has been made on both the basic and clinical research fronts. However, the incidence, recurrence, and death rates for melanoma remain unacceptably high and significant ...
Five years ago, the Melanoma Research Foundation (MRF) conducted an assessment of the challenges and opportunities facing the melanoma research community and patients with melanoma. Since then, remarkable progress has been made on both the basic and clinical research fronts. ... Future studies should be aimed at determining the actual ...
Visit the Cancer.Net Blog to review news and information about melanoma, including research announced at recent scientific meetings or in ASCO's peer-reviewed journals, including highlighted studies from 2022 and 2021. Listen to a podcast from an ASCO expert in melanoma discussing highlights from the 2021 ASCO Annual Meeting.
Immune checkpoint inhibition has fundamentally altered the treatment paradigm of resectable and unresectable melanoma, resulting in dramatic improvements in patient outcomes. With these advances, the five-year overall survival in patients with newly diagnosed unresectable disease has eclipsed 50%. Ongoing research is focused on improving outcomes further, with a considerable emphasis on ...
Research Overview. Since its founding in 2007, the Melanoma Research Alliance has become the largest non-profit funder of melanoma research worldwide. Through 415 awards, MRA has directly invested more than $150 million, and leveraged an additional $417 million in collaborative and follow-on funding towards its mission.
Background: Early diagnosis is the key to improving outcomes for patients with melanoma, and this requires a standardized histological assessment approach. The objective of this survey was to understand the challenges faced by clinicians when assessing melanoma cases, and to provide a perspective for future studies. Methods: Between April 2022 and February 2023, national and international ...
DONATE. As 2023 comes to a close, we'd like to reflect on some of the major advancements in the treatment of cutaneous and metastatic uveal melanoma— bringing hope to many patients and their loved ones. The MRF's mission is to eradicate melanoma by accelerating medical RESEARCH while EDUCATING to and ADVOCATING for the melanoma community and.
A study has disentangled the mechanisms behind one of the ways melanoma cancer cells develop resistance to treatment. The study found that, in response to some drugs, melanomas can 'break' parts ...
Here, the authors analyse 100 uveal melanoma metastases using bulk and single cell RNA-seq, TCR analysis, and immune reactivity to show potent, yet, quiescent tumour infiltrating lymphocytes that ...
While the future of melanoma treatment won't be unearthed by the answer to a single question, together they will move us towards our common goal of ending death and suffering due to melanoma. ... As the largest non-profit funder of melanoma research, MRA has dedicated over $150 million to date in support of the fight against melanoma. Click ...
In a groundbreaking advance in March 2022 led by Hopkins scientists and oncologists, the FDA approved the combination of anti-PD-1 with another checkpoint blocker, anti-LAG-3, as the first systemic treatment a patient might receive for advanced melanoma. In other research, the adjuvant (post-surgical) use of anti-PD-1 drugs or BRAF/MEK ...
Melanoma vaccines: Vaccines to treat melanoma are being studied in clinical trials. These vaccines are, in some ways, like the vaccines used to prevent diseases such as polio, measles, and mumps that are caused by viruses. Such vaccines usually contain weakened viruses or parts of a virus that can't cause the disease.
Although certain skin cancer conditions, such as BCC, have been evaluated in several studies, others, including melanoma, have not received adequate attention. There is a significant need for future research studies to address the need for skin cancer chemoprevention particularly in subgroups of patients who are immunocompromised.
Melanoma represents a public health issue. One of the biggest goals of current research is to develop new therapeutic options for patients affected by this aggressive tumor. We conducted a retrospective study including 105 patients diagnosed with cutaneous and ocular melanoma, with stages varying from pT1a to pT4b and pT4e, respectively, and we performed immunohistochemistry reactions with the ...
Linkedin. The Center for Drug Evaluation and Research (CDER) Center for Clinical Trial Innovation (C3TI) is a central hub that supports innovative approaches to clinical trials that are designed ...
WASHINGTON — A new report from the National Academies of Sciences, Engineering, and Medicine reviews evidence for 19 potential harms of the COVID-19 vaccines, and for nine potential shoulder injuries from intramuscular administration of vaccines more broadly. The committee that conducted the review identified sufficient evidence to draw 20 conclusions about whether these vaccines could cause ...