- Introduction to Genomics
- Educational Resources
- Policy Issues in Genomics
- The Human Genome Project
- Funding Opportunities
- Funded Programs & Projects
- Division and Program Directors
- Scientific Program Analysts
- Contact by Research Area
- News & Events
- Research Areas
- Research investigators
- Research Projects
- Clinical Research
- Data Tools & Resources
- Genomics & Medicine
- Family Health History
- For Patients & Families
- For Health Professionals
- Jobs at NHGRI
- Training at NHGRI
- Funding for Research Training
- Professional Development Programs
- NHGRI Culture
- Social Media
- Broadcast Media
- Image Gallery
- Press Resources
- Organization
- NHGRI Director
- Mission & Vision
- Policies & Guidance
- Institute Advisors
- Strategic Vision
- Leadership Initiatives
- Diversity, Equity, and Inclusion
- Partner with NHGRI
- Staff Search
- Center for Precision Health Research
The investigators in the Center for Precision Health Research (CPHR) develop and evaluate cutting edge genomic and informatic tools to improve the diagnosis, treatment and prevention of disease to foster next-generation healthcare.
The Center for Precision Health Research (CPHR) was established in 2021 as a research group within NHGRI’s Intramural Research Program to foster the development of cutting edge genomic and informatic approaches to health. The investigators in CPHR study a broad spectrum of disorders, with a range of heritability from common diseases associated with common genetic variants to rare diseases associated with rare variants. They also study disorders that are not inherited and instead are due to new genetic mutations and somatic mosaicism.
CPHR investigators answer critical questions in biology and medicine by applying tools ranging from high-throughput biologic methods, large scale informatics methods, classic bench laboratory approaches and animal modeling. These researchers collectively aim to change the practice of medicine across the lifespan .
Research efforts within CPHR advance the understanding of and treatments for a number of diseases or conditions. Some studies occur at a basic research level, in which investigators use genetic variation to identify pathways that can be targets for common diseases, such as diabetes and common birth defects. Other studies use informatics approaches to systematically interrogate the genome and the phenome using large-scale electronic healthcare and research datasets to identify novel clinical and genetic insights into disease.
CPHR research efforts focus on the clinical management of rare diseases and trials of small molecule therapies for genetic disorders, diseases that affect the fetus and pregnant person, disorders that disproportionately affect under-represented groups, including children and minorities, and implementation science. Many of these clinical studies take place at the NIH Clinical Center, the world’s largest research hospital.
In addition to the investigator-led research sections and units, CPHR includes two core facilities that support novel modes of research across the NHGRI Intramural Research Program. The first is the Reverse Phenotyping Core, which supports researchers by performing genomic ascertainment research with post-hoc phenotyping to delineate novel gene-phenotype associations. The second is the Cohort Analytics Core, which will host numerous large-scale genotype and phenotype databases (e.g., the UK BioBank) and will support investigators’ analyses of these datasets using cutting-edge informatics techniques.
CPHR Seminar Series 4th Thursday of Every Month, 5-6 PM ET
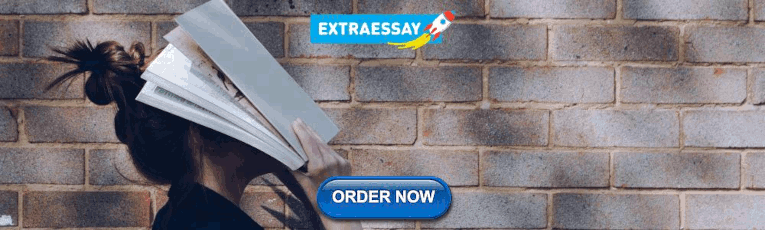
Senior Investigators
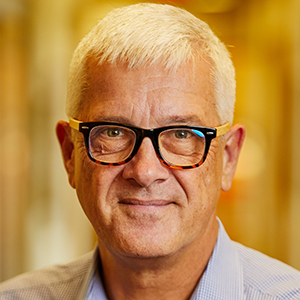
- Director & NIH Distinguished Investigator
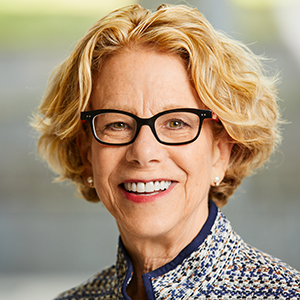
- Senior Investigator
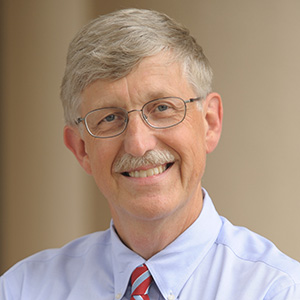
- NIH Distinguished Investigator
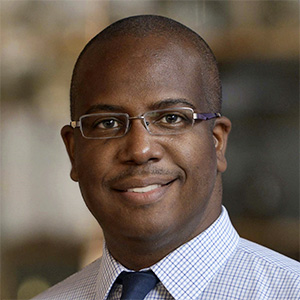
- Clinical Implementation Unit
Investigators
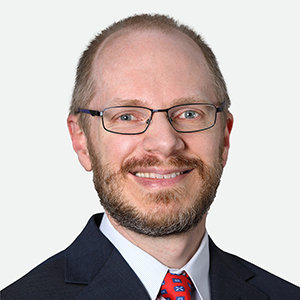
- Adjunct Investigator
Associate Investigators
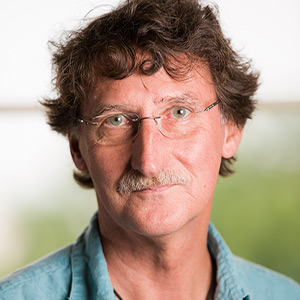
- Associate Investigator
- Molecular Genetics Section
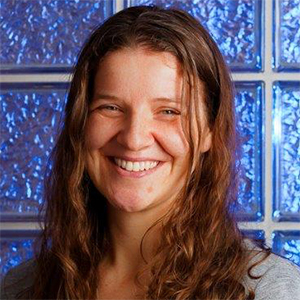
Adjunct Investigators
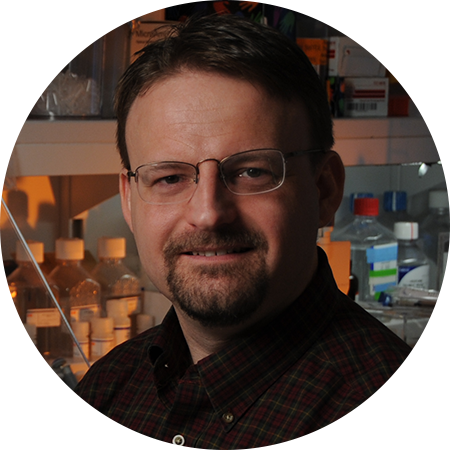
Staff Clinicians
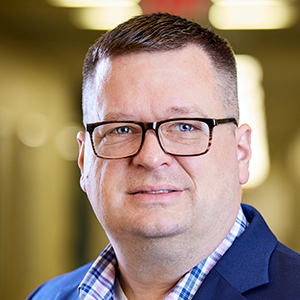
- Staff Clinician
Research Assistants and Coordinators
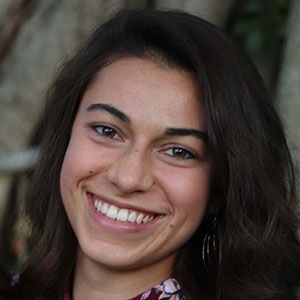
- Clinical Research Coordinator
Featured Content
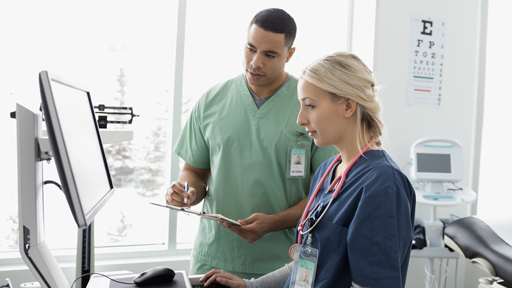
Last updated: March 20, 2024

New Center for Precision Health Aims to Transform Population Health, Patient Care
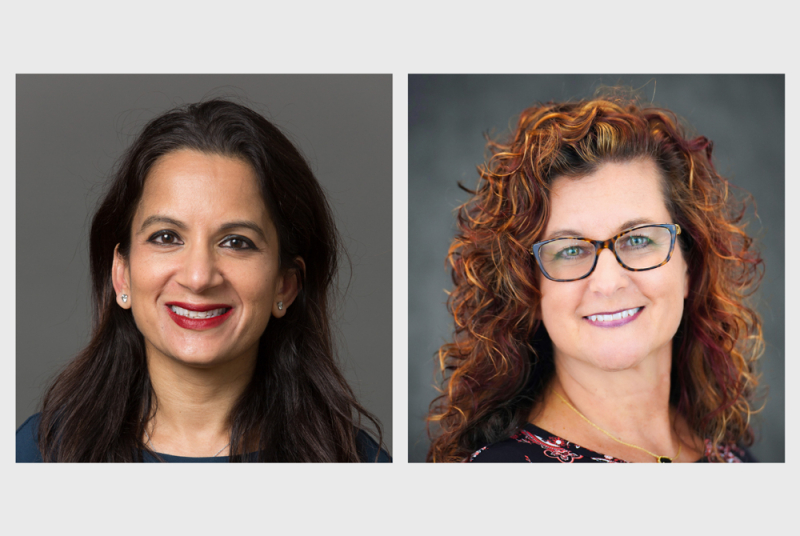
The Duke Clinical and Translational Science Institute (CTSI) is pleased to announce the launch of the Center for Precision Health (CPH) , a collaboration that will harness the power of genomic, biomarker, and health data to transform patient care and population health.
The Duke University School of Medicine has a rich history of translational discovery science leveraging genetics, genomics, and other omic technologies coupled with data science and informatics. With continuing advances in genomics, biomarker technology, and computational biology alongside improvements in electronic health records and machine learning, the CPH will work to bridge discovery science with personalized patient care.
“This collaborative effort will bring together researchers from across Duke that will enable us to improve patient care and help move more discoveries in basic and translational research into clinical care,” said Mary E. Klotman, MD , executive vice president for health affairs and dean of the School of Medicine. “The Center for Precision Health is poised to become a powerhouse for genetic and genomic discovery, outreach, and education. I’m excited to see what the future holds.”
Svati H. Shah, MD, MHS , Ursula Geller Distinguished Professor of Medicine, has been named director of the CPH. Julie Eckstrand, RPh, will serve as executive director.
“The Center for Precision Health brings together all the essential components needed to transform how we view and use data to care for our patients and improve the health of our communities,” said Duke CTSI Director Susanna Naggie, MD, MHS . “The work of this center aligns with the strategic priorities of our institute and the health system overall.”
The Center for Precision Health brings together all the essential components needed to transform how we view and use data to care for our patients and improve the health of our communities. The work of this center aligns with the strategic priorities of our institute and the health system overall.
This new center will fuel clinical, translational, and basic science and serve as a foundational network for coalescing the Duke scientific community. The CPH aims to expand genetic discovery and catalyze clinical genetics care, conduct clinically impactful translational research, perform cutting-edge implementation science, engage the community and build trust, and educate the precision health workforce of tomorrow.
The CPH has five programs to enable these goals: population genetics, translational discovery, implementation science, community engagement and ethics, and precision health education. The center will also serve as the scientific and operational home for OneDukeGen , a genomic medicine research study and biorepository that will perform genetic sequencing on 150,000 Duke Health patients and research study participants and will return clinically actionable genetic test results so they may make informed decisions about their health. The CPH will also house the Duke Kannapolis clinical research site and the MURDOCK Study population-based cohort .
The center will have strong ties to the Duke School of Medicine Precision Genomics Collaboratory and Duke AI Health and will collaborate closely with basic science and clinical departments within the school and with the Duke Health System.
“We are so excited about launching the Center for Precision Health,” Shah said. “This will unite many different aspects of precision health, with a focus on research that has impact for Duke patients. This will necessitate multi-disciplinary teams and highly collaborative science.”
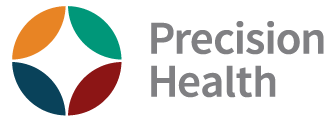
Leading the Biomedical Revolution in Precision Health
Precision Health reimagines medicine to focus on predicting, preventing, and curing disease precisely. Marrying two seemingly different approaches — high-tech and high-touch — this Stanford Medicine vision tailors health care to the unique biology and life circumstances of each individual, with an emphasis on catching disease before it strikes. Precision Health represents a fundamental shift to more proactive and personalized care that empowers people to lead healthy lives.

2020 Health Trends Report spotlights the rise of the data-driven physician
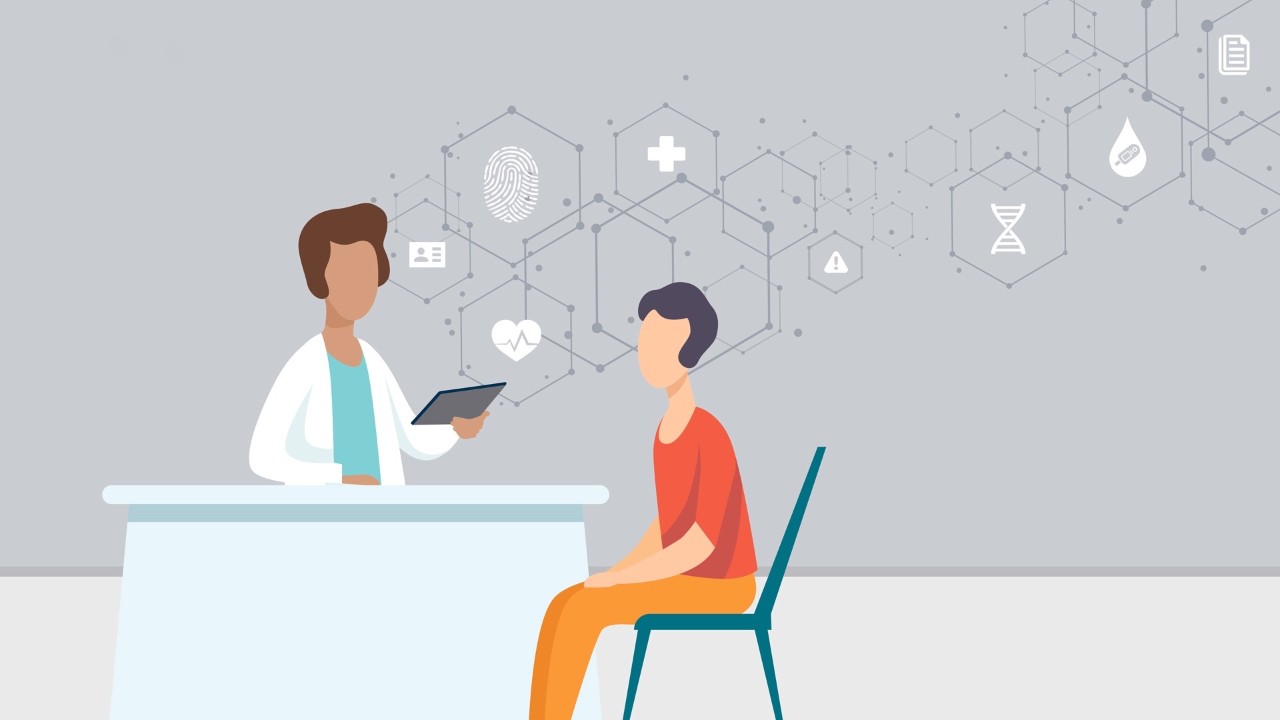
Humanwide: Bringing Precision Health to Life
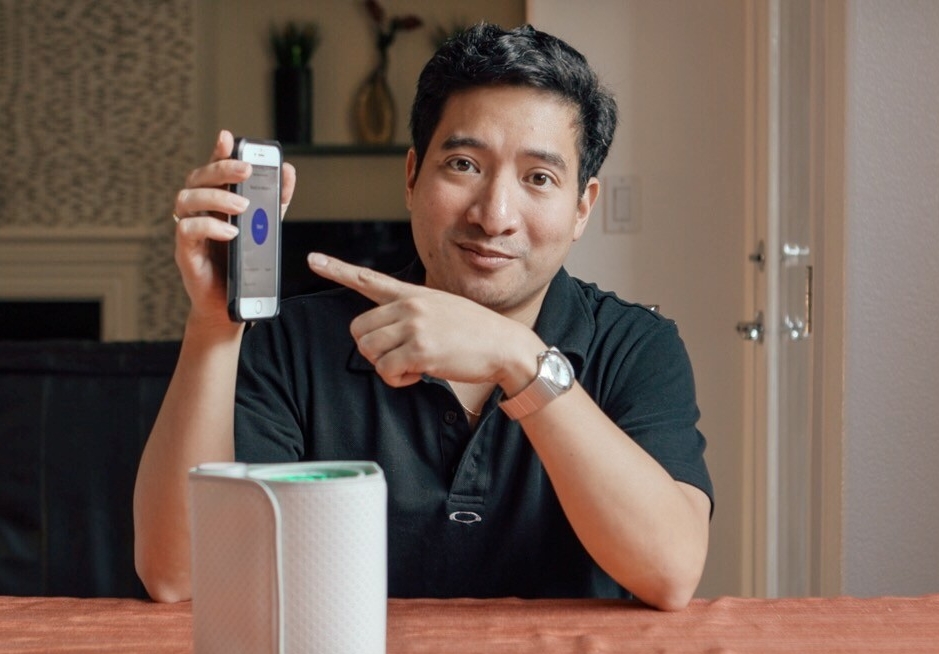
Read an excerpt from Discovering Precision Health
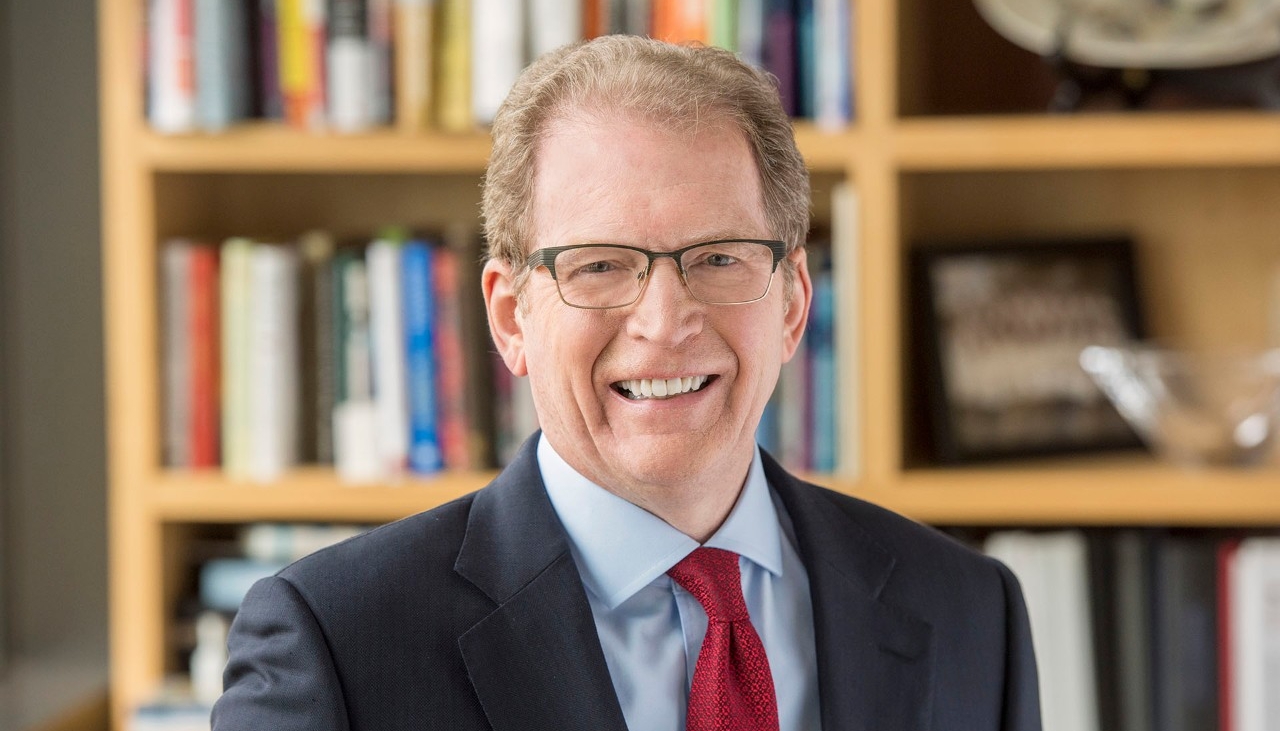
The new Stanford Hospital blends technology, innovation to improve care

How DNA testing can personalize patient care
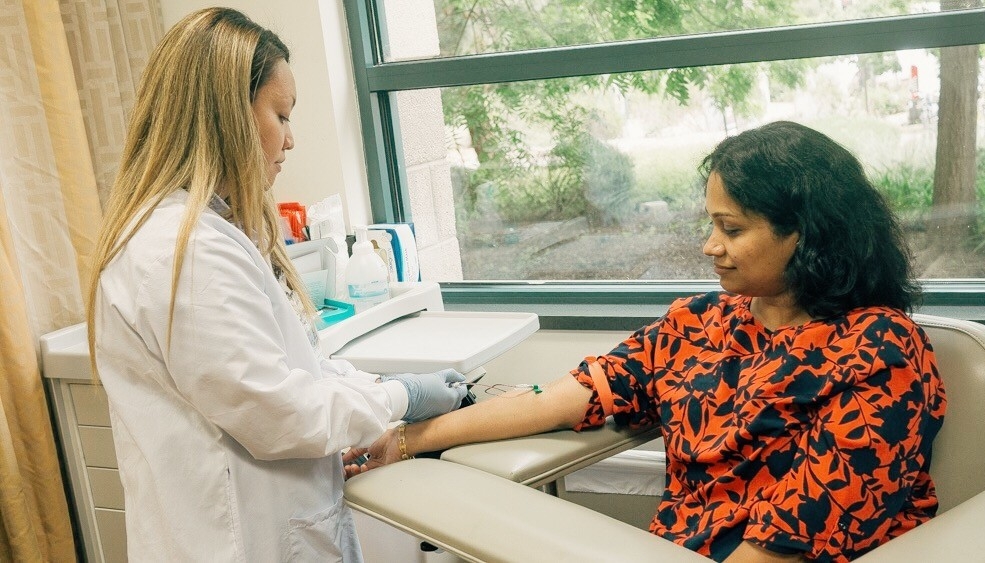
‘Ageotypes’ provide window into how individuals age
Michael Snyder is the senior author of a study that identified specific biological pathways along which individuals age over time.

Precision Health News
- February 20, 2024 Stanford Medicine study identifies distinct brain organization patterns in women and men
- October 3, 2023 Neurooncologist Reena Thomas receives CIRM award of nearly $12 million for immunotherapy research
- August 24, 2023 Stanford Medicine first to try out novel tumor-targeting radiation therapy machine
More Precision Health News
Follow Stanford Medicine
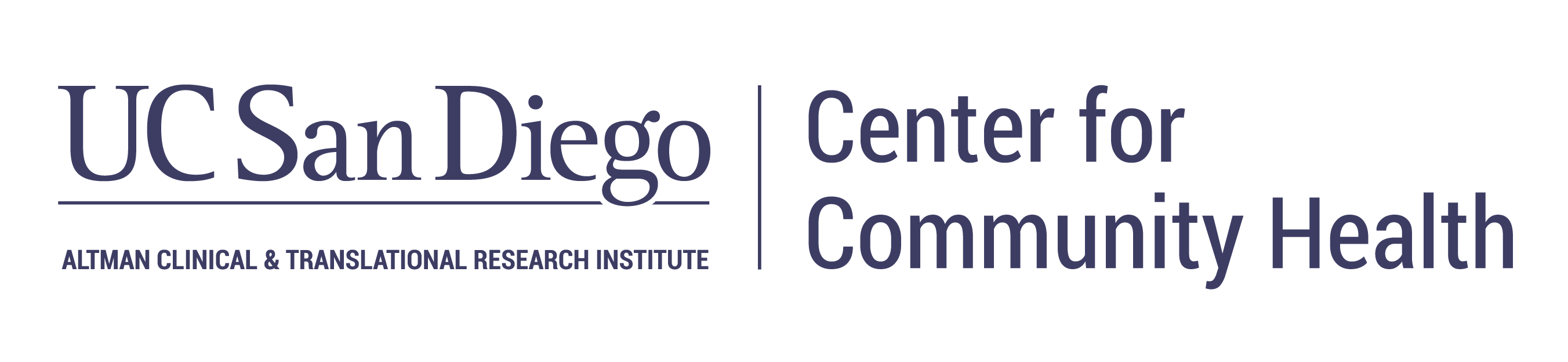
Precision health is a unified approach to match a full range of promotion, prevention, diagnostic, and treatment interventions to fundamental and actionable determinants of health; to not just address symptoms, but to directly target genetic, biological, environmental, and social and behavioral determinants of health. Precision health approaches use advanced computing tools to achieve these goals. Events of 2020, including the COVID-19 pandemic, brought into the vernacular the concept of precision public health, which concerns the use of data and technology to improve public health equity, policy, and practice.
Join our mailing list
Access our newsletter archive .
Join the Live Well @ Work newsletter
Privacy Policy
You are using an out of date browser that can't display the contents of this site. Please upgrade your browser to improve your experience.

An official website of the United States government
Here's how you know
Official websites use .gov A .gov website belongs to an official government organization in the United States.
Secure .gov websites use HTTPS A lock ( Lock Locked padlock ) or https:// means you’ve safely connected to the .gov website. Share sensitive information only on official, secure websites.

Nutrition for Precision Health, powered by the All of Us Research Program
Program snapshot.
The goal of the NIH Common Fund’s Nutrition for Precision Health, powered by the All of Us Research Program , is to develop algorithms that predict individual responses to food and dietary patterns. Nutrition plays an integral role in human development and in the prevention and treatment of disease. However, there's no such thing as a perfect, one-size-fits-all diet. The NPH program will build on recent advances in biomedical science including artificial intelligence (AI), microbiome research, as well as the infrastructure and large, diverse participant group of the All of Us Research Program . These advances provide unprecedented opportunities to generate new data to provide insight into personalized nutrition also referred to as precision nutrition.
In addition, the first ever Strategic Plan for NIH Nutrition Research emphasized opportunities to improve our understanding of how individual human biology and molecular pathways influence relationships among diet and environmental, social, and behavioral factors to influence health. Designed to implement aspects of the Strategic Plan, the Nutrition for Precision Health program will conduct a study nested in the All of Us Research Program to explore how individuals respond to different diets. The NPH study is the first ancillary study to leverage the All of Us infrastructure to answer scientific questions important to participants like understanding more about the role of nutrition in health. High-quality nutrition studies such as the NPH study will help individuals and their health care providers create healthy, precise, and effective diet plans.
The objectives of the study are:
- To examine individual differences observed in response to different diets by studying the interactions between diet, genes, proteins, microbiome, metabolism and other individual contextual factors
- To use artificial intelligence (AI) to develop algorithms to predict individual responses to foods and dietary patterns
- To validate algorithms for clinical application
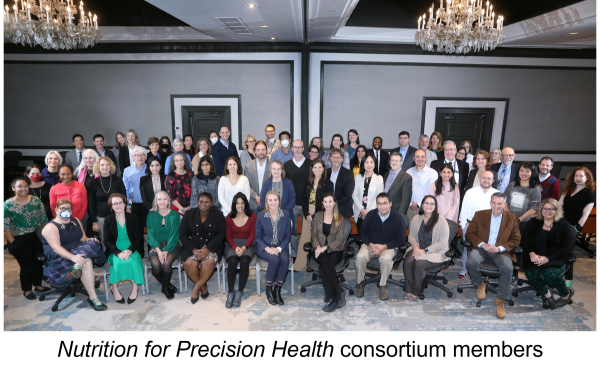
The Nutrition for Precision Health program includes several integrated components:
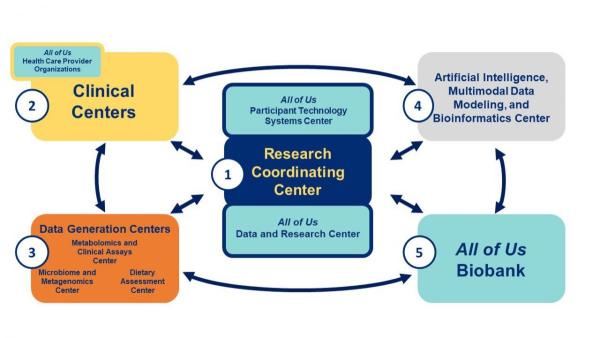
Nutrition for Precision Health, powered by the All of Us Research Program is a service mark of the U.S. Department of Health and Human Services (HHS).
NPH Center and Site Locations
Click the image below to view the interactive location map, detailing the NPH Centers and Sites.
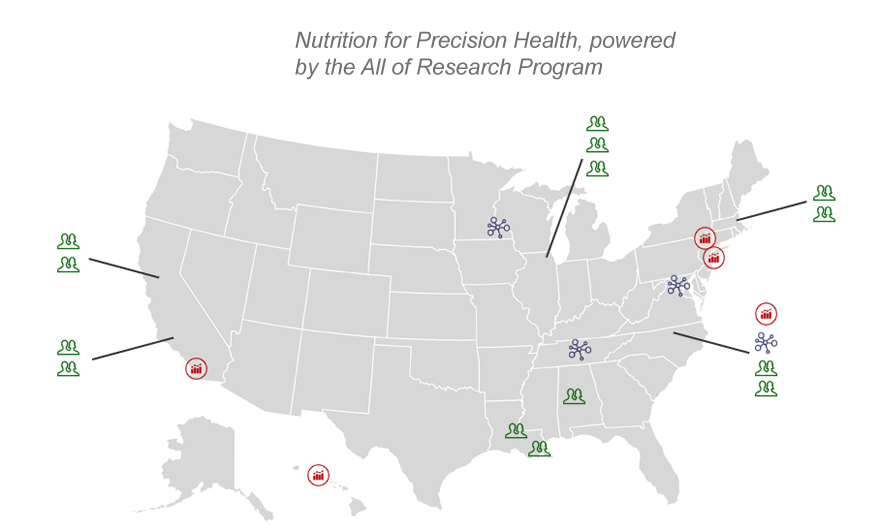
Stay Connected Sign Up for the NPH listserv
Join our listserv to receive updated and announcement about the program.
Enrollment is Now Open for NPH
Participate in a study to help researchers predict how our bodies respond differently to food. More information can be found here .
Request for Applications NEW!
The AIMINGS AI for Precision Nutrition Pilot Program, part of the NPH Consortium , released a new funding opportunity . As the AI Center for the NPH consortium, AIMINGS (Artificial Intelligence, Modeling, and Informatics for Nutrition Guidance and Systems) is developing AI, machine learning (ML), Big Data methods, and other data science approaches to better understand and improve precision nutrition .
This call for proposals seeks research projects that develop and utilize AI and other computer-aided approaches to facilitate precision nutrition.
This is a great opportunity for investigators to receive funding for a project that can eventually enhance into a larger project or an R01 application. Awardees will be connected with a community of researchers working on AI for precision nutrition, a new and emerging field.
Proposals are welcome from applicants anywhere in the US from diverse backgrounds and at varying career levels. Applicants who are in the early stages of their careers and investigators that are underrepresented in the scientific workforce are strongly encouraged to apply. Applicants cannot be current members of the NPH consortium.
Interested applicants are encouraged to attend the applicant webinar prior to submitting. The webinar will be held on July 20th between 3:30pm and 4:30pm ET. Questions should be emailed to [email protected] by July 13th as the AIMINGS team will do their best to cover submitted questions during the webinar. Registration is required and can be found here .
The deadline to apply to the RFA is October 12, 2023.
Announcements
NIH launches largest precision nutrition research effort of its kind.
Science News
Could Precision Nutrition Be a Game Changer for Health?
Diabetes Discoveries & Practice Blog Will Precision Nutrition Help Patients Prevent or Treat Diabetes?
Healio interview with NPH's leadership: NIH will help guide precision nutrition research.
Precision nutrition: Tailored diet assessments to become a mainstay in medical care by 2030.
Press Releases
NIH awards $170 million for precision nutrition study
Program Background
On September 11, 2020 the NIH Council of Councils approved the concept for a new NIH Common Fund program “Nutrition for Precision Health, powered by the All of Us Research Program .” This program will be refined by NIH for potential implementation in Fiscal Year 2022. The archived videocast of the Council of Councils meeting is publicly available and can be viewed here (Nutrition for Precision Health discussion begins at 1:54:00 ). Slides and a brief write-up are also available.
This page last reviewed on March 19, 2024
- Coronavirus Updates
- Education at MUSC
- Adult Patient Care
- Children's Health
- Hollings Cancer Center
- Research at MUSC
- MUSC Catalyst News
- Precision Health
MUSC shines spotlight on precision health, which research leader calls a top priority
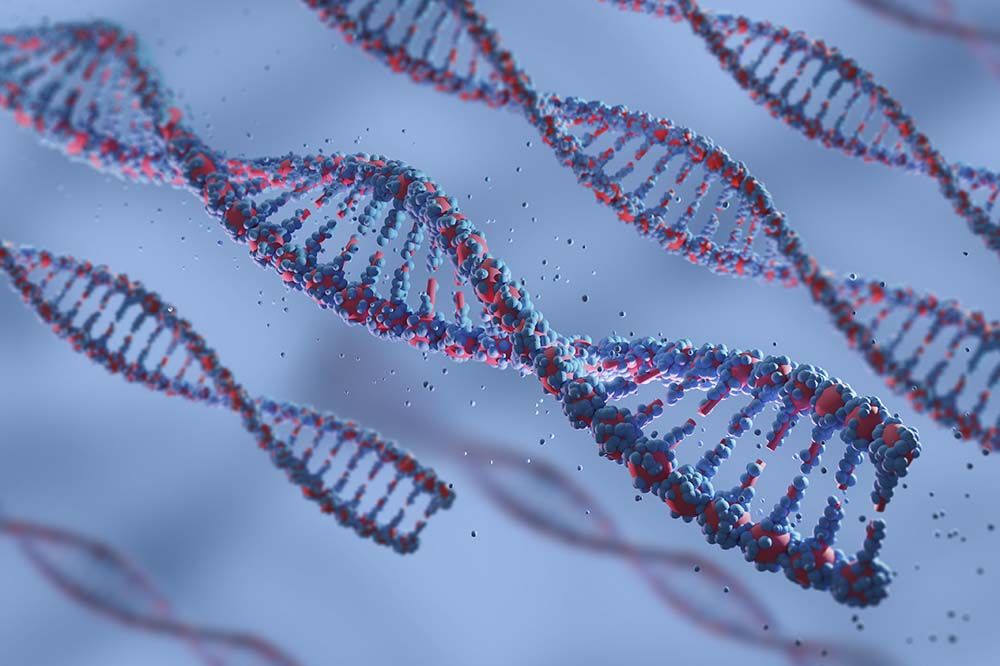
The Medical University of South Carolina is putting precision health front and center, bringing together experts to talk about what it is, how it’s being used and what the future may hold. MUSC recently held a Precision Health Research Symposium, led by Lori L. McMahon, Ph.D. , vice president for Research and a professor of Neuroscience.
“The landscape of medicine and health care is undergoing significant transformation with precision health as a top priority. This evolution is a result of our deepening understanding of genomics and data science, which has opened the door for more precise, individualized health care,” McMahon said.
The event included speakers from MUSC, the University of Alabama-Birmingham, the HudsonAlpha Institute of Biotechnology, Helix and the Greenwood Genetic Center. They talked about the roles of data, genomics, research and systems in precision health.
Precision health through data
Matt Might, Ph.D., director of the Hugh Kaul Precision Medicine Institute at UAB, made a bold prediction: Historians will see data, a key aspect of precision health, as the biggest treatment of this century.
“I see precision medicine, really, as this field that delivers data as a drug. To do it right, precision medicine really needs computation. There's no way around managing the massive amounts of data from genomes to images without heavy intervention from computation.”
Computation is key, he said, in addition to other help from technology, including artificial intelligence. Mightgave a real-life example of how AI can improve patient care. It involved a 19-year-old woman named Kelsey who was suffering from intractable cyclic vomiting syndrome. “I think she was 5 feet, 4 inches tall, but weighed 75 pounds. She was severely malnourished at this point, suffering dozens of vomiting episodes per day,” Might said.
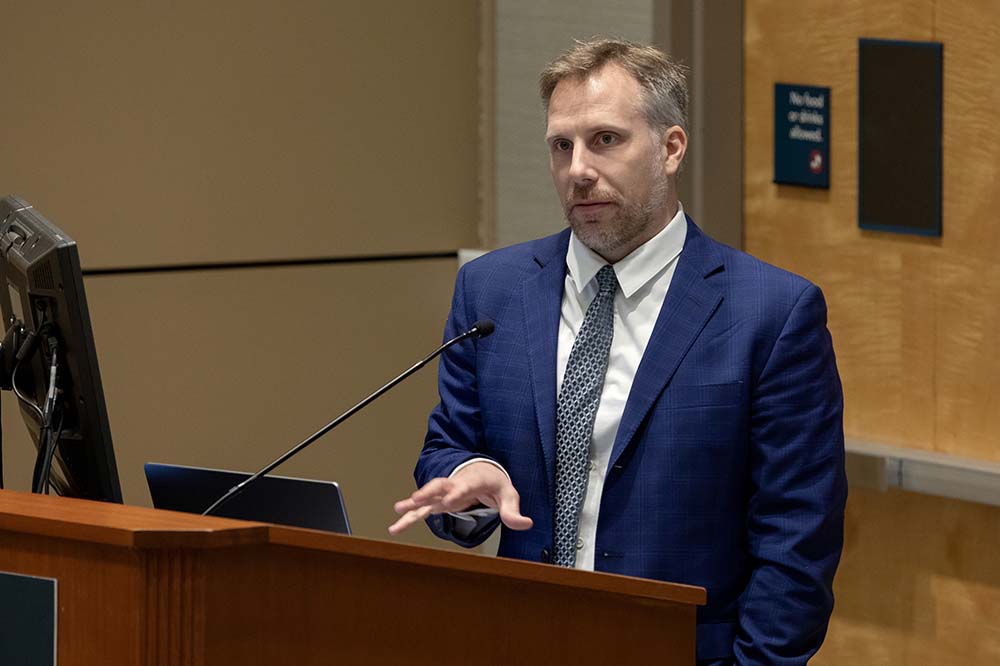
“Her medical team said, ‘Look, we've tried everything that we know about in this case, and nothing seems to be working.’ But everything they tried totaled 25 things. To be fair, 25 things is a lot. But it's not absolutely everything.”
So Might and his team, experts in precision medicine, put their technological skills to work. They used artificial intelligence to scan millions of medical research articles about nausea and unearthed a treatment that hadn’t been tried with Kelsey: nasally inhaled isopropyl alcohol.
To their relief, it worked. Kelsey’s life changed dramatically.
“If you fast forward eight months, not only has she not vomited since, but she was able to use this again to successfully arrest every episode of nausea. She was up to 125 pounds. And she was feeling well enough to get married,” Might said.
Precision health through genomics
Not every precision health story ends with a wedding but some do include life-changing diagnoses, treatments and more. And they begin, in some cases, with the use of genomics.
Genomics is the study of a person’s genes and how they interact with each other and the environment. Bruce Korf, M.D., knows a lot about that. He serves as associate dean for Genomic Medicine at the UAB School of Medicine and chief genomics officer.
Korf’s talk at MUSC focused on how genetic information can help patients, particularly people with rare diseases. Doctors refer patients to his team, which looks at their cases and when needed, performs genetic testing. That sometimes leads to a diagnosis that wasn’t obvious before. That, in turn, can lead to the right treatments.
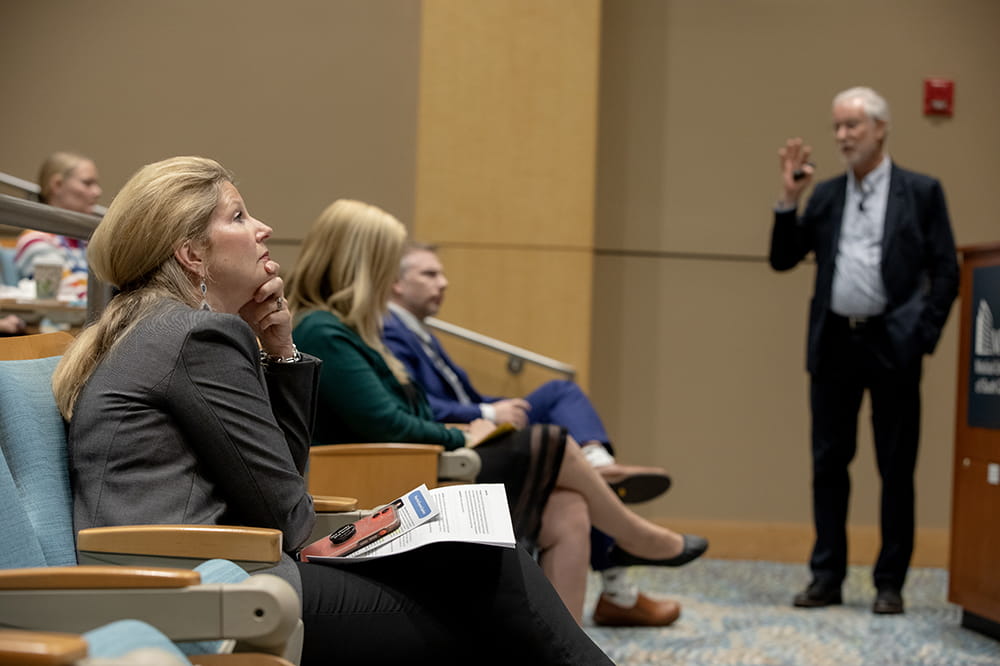
And Korf sees an even greater role for genetic testing in the future – despite mixed public perceptions about it. “How do you get clinicians to use genetic and genomic testing in day-to-day practice? Some clinics are really eager to adopt genetic testing, while others are more hesitant,” he said.
As technological innovation, clinical utility, implementation and education continue to evolve, Korf said genomics and precision health will become more routine. “I think if you went to sleep and woke up in 20 years, you would not recognize the practice of medicine.”
And that could have big benefits, not only for people with rare diseases but also more common ones such as Parkinson’s and Alzheimer’s. Speaker Richard Myers, Ph.D., chief scientific officer, faculty investigator and president emeritus of the HudsonAlpha Institute for Biotechnology, talked about studying the genetics of neurodegenerative diseases. “It’s slow going but really important,” he said.
Also important, in his view: encouraging the public to participate in programs such as In Our DNA SC , a statewide effort to improve access to personalized health care and create a genetic and research database. Alabama has its own version . Both rely on the public’s willingness to get involved.
“Why do people participate in a population-facing component? They want to contribute to research and have an interest in their own future health. They want to help their children and grandchildren,” Myers said.
Precision health’s role in research
That idea of looking toward the future, a future with new health care options for a range of ailments, informs the work of researchers using precision health.
Several speakers talked about that, including Dan Judge, M.D., a professor in the College of Medicine at MUSC and the leader of In Our DNA SC . In Our DNA SC’s genetic and research database will help scientists for years to come. “We need to go from a disease-first to a gene-first approach. Let genetics lead the diagnosis,” Judge said.
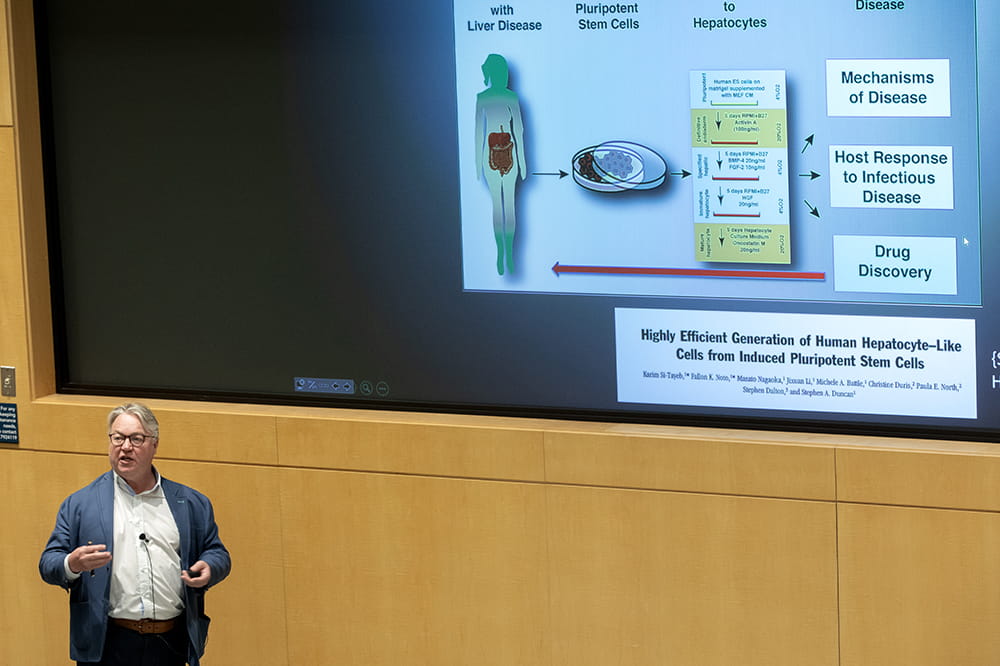
Other scientists weighed in on the role of precision health in research by highlighting how they’re using it, including:
- Alain Greige, an M.D.-Ph.D. student of Neuroscience at MUSC. He’s working with Christopher Cowan, Ph.D., chair of the Department of Neuroscience, on an RNA-based approach to treating neurodevelopmental disorders.
- Steve Duncan, Ph.D., chair of Regenerative Medicine and Cell Biology at MUSC. He’s looking at possible treatments for a genetic disorder that causes high cholesterol levels.
- Saverio Gentile, Ph.D., associate professor in the Department of Cell and Molecular Pharmacology and Experimental Therapeutics at MUSC. He’s looking for new ways to target and treat cancer.
- John Wrangle, M.D., associate professor in the College of Medicine who treats patients in Hollings Cancer Center. He gave a broader take on the role of precision health in cancer research.
Precision health systems
These research projects wouldn’t be possible without a systematic approach. And as the name suggests, precision health requires precision when it comes to the systems needed to implement it.
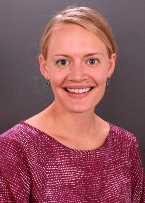
Caitlin Allen, Ph.D. , an assistant professor in the Department of Public Health Sciences at MUSC and the director of Outreach and Implementation Research for In Our DNA SC, was among the speakers talking about getting systems in place to leverage precision health on a large scale, with attention paid to including everyone.
And representatives of Helix, a population genomics company and MUSC partner for In Our DNA SC, talked about standardizing the process of screening for health conditions. Nicole Washington, Ph.D., director of Research and Clinical Informatics at Helix, talked about a new approach. “The use of genomics in health care today is inefficient and inadequate. We need to change the model of how it’s done.”
Here's one way she suggested doing that when it comes to genomic screening. “Stop treating it like a one-off. We’re proposing going back into that sequence as much as possible for a patient’s lifetime. Sequence once, query often.”
Finally, representatives of the Greenwood Genetic Center, Rich Steet, Ph.D., and Heather Flanagan-Steet, Ph.D., discussed integrating research into clinical genetics care. Their South Carolina nonprofit provides genetic services, diagnostic testing, educational programs, resources and research into medical genetics. The partnership between GGC and MUSC combines the expertise of both organizations to expand services across the state and further their capability to improve prevention, diagnosis and treatment of diseases.
Precision health going forward
So what’s next? A lot, according to host McMahon. Precision health is an important part of the present and future of health care at MUSC and beyond.
“Harnessing the information in our DNA for research has the power to drive discoveries further and faster than ever before. With ever-advancing technologies, we are more equipped than ever to explore the intricacies of the human genome with unprecedented depth and precision,” she said.
“By studying the genetic variations among individuals and populations, we can uncover crucial insights into the causes and progression of diseases and ultimately inform potential treatment strategies now and for generations to come.”
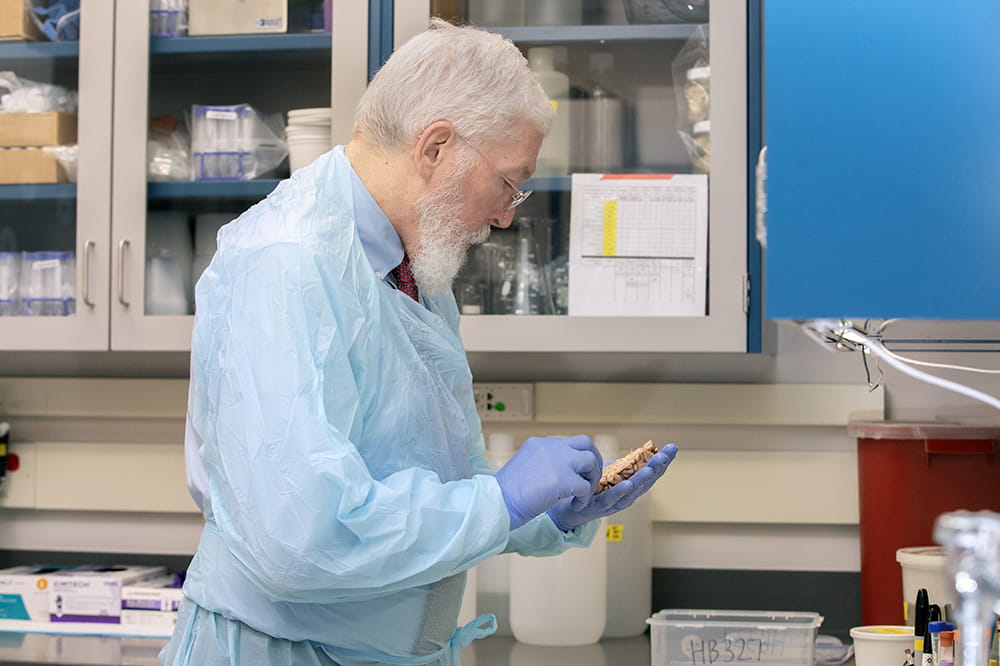
Dementia Developments
As MUSC works with Clemson and USC on new Alzheimer’s Disease Research Center, scientist sees issue through professional and personal lenses.
Get the Latest MUSC News
Get more stories about what's happening at MUSC, delivered straight to your inbox.
About the Author
Helen Adams
Categories: Research
General Inquiries
Call today to schedule an appointment or fill out an online request form. If requested before 2 p.m. you will receive a response today.
713-798-1000
Monday - Friday 8 a.m. - 5 p.m.
Request Now
Request non-urgent appointments
Find a Physician
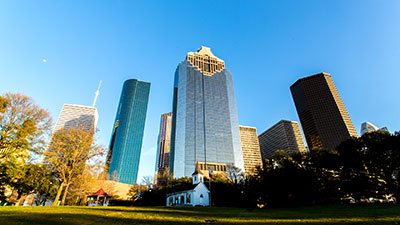
America's fourth largest city is a great place to live, work and play. Find out why. Get to Know Houston
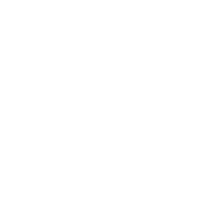
Give Careers Intranet
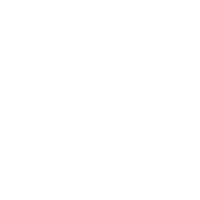
Center for Medical Ethics and Health Policy
- Meet Our Team
- Health Policy Program
- Clinical Ethics
- Health Equity and Community Outreach
- In The News
- Baylor College of Medicine
- Academic Centers
- Medical Ethics and Health Policy
- Ethical, Legal and Social Implications (ELSI) & Genetics/Genomics
The Precision Medicine Study on Alzheimer’s and Related Dementias
Project description.
The Precision Medicine for Alzheimer’s Disease and Related Dementias Project is a joint project conducted in collaboration with the Center for Alzheimer’s and Neurodegenerative Disease at Baylor College of Medicine. This project aims to integrate precision medicine (cognitive testing, genomic testing, and neuroimaging) into Alzheimer’s research and care to increase early detection of ADRD and improve clinical outcomes. In this project, at-risk patient-participants enroll together with study partners (potential caregivers) to receive cognitive testing, genetic testing, and neuroimaging (MRI, PET), and a comprehensive profile of their risk of developing ADRD. In conjunction with CAND, the Center for Medical Ethics and Health policy is assessing the impact of precision medicine testing and disclosure on both patient-participants and their potential caregivers in order to facilitate ethical and effective implementation of precision medicine disclosure.
The AD Project is enrolling 250 patient-participants and their study partners in the Houston area. The diverse settings of the study allow us to evaluate the impact of precision medicine on a diverse community, including both English and Spanish-speaking populations.
We are exploring impact across a variety of dimensions including:
- Attitudes towards different testing modalities, and the perceived relative importance of different test results for future planning.
- Psychological impact of testing, including both patient-participant and study partner worry and self-efficacy around test results, as well as the effects of disclosure of patient-participant results on study partner burden.
- How results are actually used to improve patient-participant health behaviors for the purposes of improving ADRD outcomes.
Patient-participants and study partners complete pre-disclosure surveys to assess their expectations around results prior to receiving them. At follow-up, both patient-participants and study partners provide information about their understanding of and reaction to their results. Study partners also provide information about their caregiver burden and gains in dementia caregiving.
Supported by : a private foundation
Project Team
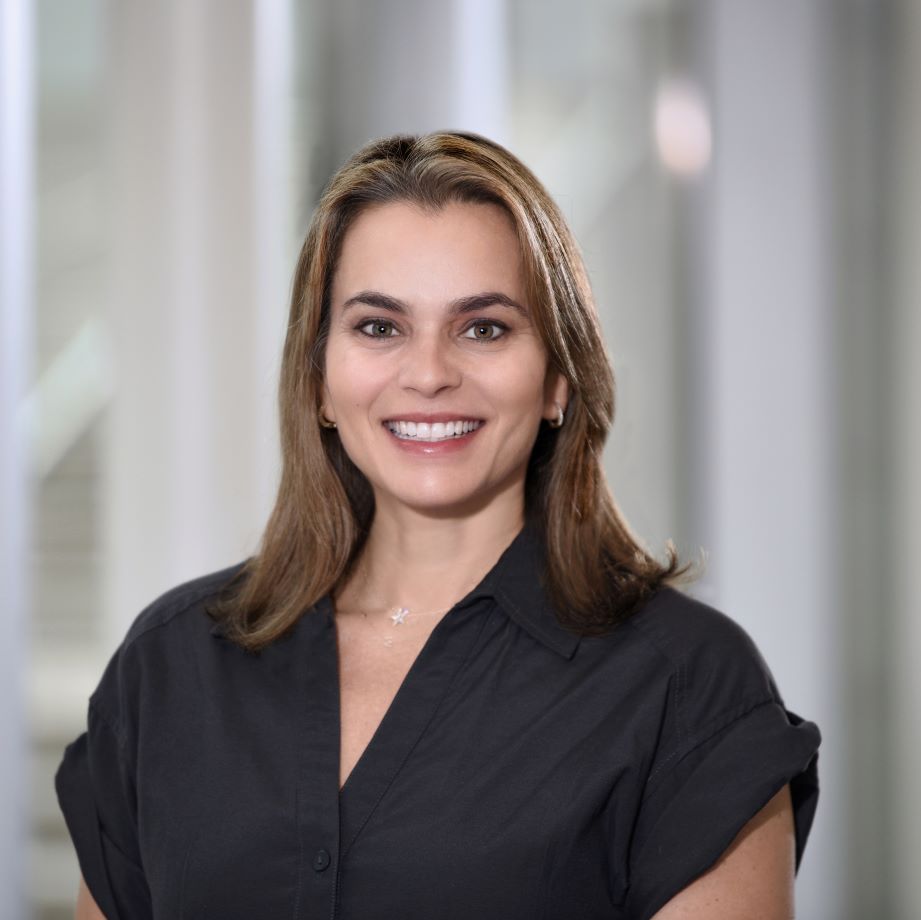
Publications
Stevens Smith et al., Research participants' perspectives on precision diagnostics for Alzheimer's disease , Journal of Alzheimer’s Disease
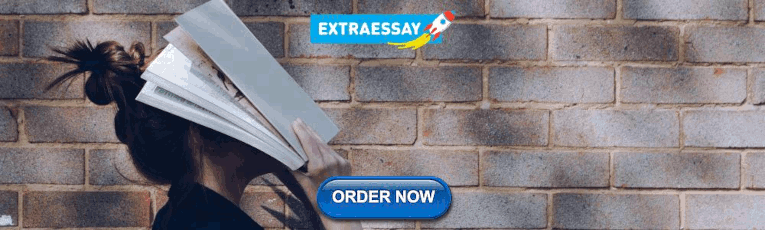
Presentation
Levchenko et al., Impact of Precision Medicine on Patient and Caregiver Perceptions of Alzheimer’s Disease. American Society for Bioethics and Humanities Annual Conference. October 2023.
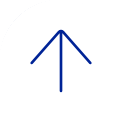

- News Releases
NIH launches largest precision nutrition research effort of its kind
Enrollment opens at 14 sites nationwide, including Pennington Biomedical, to drive advances in precision nutrition
Pennington Biomedical Research Center
image: A participant has his in person visit at Pennington Biomedical for the Nutrition for Precision Health study. view more
Credit: Madison Page/PBRC
The National Institutes of Health is now enrolling participants in a landmark initiative to advance nutrition research. Nutrition for Precision Health, powered by the All of Us Research Program , or NPH, is working with 14 sites across the United States – including Pennington Biomedical Research Center and LSU Health Sciences New Orleans in Louisiana – to engage 10,000 participants from diverse backgrounds and learn more about how our bodies respond differently to food.
“ Nutrition for Precision Health brings us a step closer to precision medicine. The study will generate a massive dataset, a wealth of biospecimens and the algorithms that will lead to personalized dietary prescriptions that can promote health, prevent heart attacks or strokes, and importantly, address health disparities,” said Pennington Biomedical Executive Director John Kirwan, Ph.D.
NPH will use artificial intelligence-based approaches to analyze information provided by participants in order to develop algorithms that predict responses to dietary patterns. The study’s findings may one day allow healthcare providers to offer more customized nutritional guidance to improve overall health.
“Poor diet is one of the leading causes of preventable disease and death around the world. If everyone followed the healthy eating guidelines that we have available now, we still may not achieve optimal health because our bodies respond differently to food,” said Holly Nicastro, Ph.D., MPH, coordinator of NPH. “Through this study, we are looking to better understand differences in individual responses and pave the way for more tailored guidelines in the future.”
Nutrition is important for the prevention and treatment of most chronic conditions and diseases, including hypertension, diabetes, and stroke. However, current dietary recommendations do not consider individual biological differences in how people respond to foods or ways and timing of eating. The goal of precision nutrition is to move from a “one-size-fits-most” approach to more specific recommendations that are based on each individual’s unique characteristics and environments.
“Food lies at the epicenter of health and disease. But clinical nutrition is still limited to a one-size-fits-all-approach that far too often fails a large segment of the population,” said Eric Ravussin, Ph.D., Associate Executive Director for Clinical Science at Pennington Biomedical Research Center.
NPH will study how a range of factors, including genes, lifestyle, health history, the gut microbiome (the community of microorganisms that live in the human gastrointestinal tract), and social determinants of health (the conditions in which people live, work, and age that affect health), influence a person’s response to diet.
To participate in NPH, individuals must be aged 18 or over and must enroll in or already be enrolled in NIH’s All of Us Research Program. All of Us is an effort that aims to engage at least 1 million participants in building a health database that reflects the diversity of the U.S., to help speed up medical research and enable individualized prevention, treatment, and care options.
The NPH study consists of three components. All study participants will participate in the first component, while a subset will take part in the other two components. In the first component of the study, participants will be asked to complete surveys, report their daily diets, and provide blood, urine, and stool samples for lab tests, including microbiome analysis. In the second component, a subset of participants will be given diets selected by researchers. In the third component, participants will also be given diets selected by researchers but will be requested to reside in a research center while on the diets.
Participants from all three components of the study will participate in meal challenge tests that measure biological changes after they consume a standardized study-provided meal or drink. Participants will receive interpreted information from the study on their health, including body fat percentage, microbiome makeup, metabolism, and diet composition.
“What we need is precision, the ability to prescribe diets that account for the factors unique to each person, such as their genetics, metabolism, physiology, behavior, even the microorganisms in their body,” said Leanne Redman, Ph.D., Associate Executive Director for Scientific Education at Pennington Biomedical.
NPH will link participants’ data from the study to information obtained through the All of Us Research Program, including genetics information and data from electronic health records and additional surveys. The study will leverage advances in AI to analyze this vast amount of data from participants to develop algorithms predicting how a person will respond to a particular food or diet based on various factors. All of this data will ultimately be accessible through All of Us ’ data platform, the Researcher Workbench , to support many other studies on health and disease. Strict safeguards are in place to keep the data secure and protect participant privacy.
“Nutrition is perhaps one of the most powerful medicines we have available, but is among the least understood,” said Geoffrey Ginsburg, M.D., Ph.D., All of Us ’ chief medical and scientific officer. “By tapping into the All of Us infrastructure and platform, NPH will be set apart from other nutrition studies by its scale and diversity. The value of NPH will be amplified by the research community as new data types are made broadly available in the Researcher Workbench to explore and advance our understanding of nutrition and health.”
NIH funded six clinical centers to conduct the study at enrollment sites in various regions of the country. These centers will implement the study modules and engage diverse communities to participate in NPH.
NPH is led by multiple institutes and centers within NIH, including the NIH Common Fund; All of Us Research Program; Office of Nutrition Research; National Institute of Diabetes and Digestive and Kidney Diseases; National Heart, Lung, and Blood Institute; Eunice Kennedy Shriver National Institute of Child Health and Human Development; National Cancer Institute; and National Center for Advancing Translational Sciences.
Currently, the NPH study materials are available only in English. Spanish-language materials will be available at a future date.
To learn more about NPH and how to join the study, please visit https://nutritionforprecisionhealth.org/ . To enroll at Pennington Biomedical, visit https://www.pbrc.edu/nph .
The work described here is supported by National Institutes of Health award # 1 UG1 HD107696-01.
“All of Us” and “ Nutrition for Precision Health, powered by the All of Us Research Program ” are service marks of the U.S. Department of Health & Human Services.
About the NIH Common Fund
The NIH Common Fund encourages collaboration and supports a series of exceptionally high-impact, trans-NIH programs. Common Fund programs are managed by the Office of Strategic Coordination in the Division of Program Coordination, Planning, and Strategic Initiatives within the NIH Office of the Director in partnership with the NIH Institutes, Centers, and Offices. More information is available at the Common Fund website: https://commonfund.nih.gov .
About the All of Us Research Program
The mission of the All of Us Research Program is to accelerate health research and medical breakthroughs, enabling individualized prevention, treatment, and care for all of us. The program will partner with one million or more people across the United States to build the most diverse biomedical data resource of its kind, to help researchers gain better insights into the biological, environmental, and behavioral factors that influence health. For more information, visit www.ResearchAllofUs.org , www.joinallofus.org , and https://www.allofus.nih.gov/ .
About the National Institutes of Health
NIH, the nation's medical research agency, includes 27 Institutes and Centers and is a component of the U.S. Department of Health and Human Services. NIH is the primary federal agency conducting and supporting basic, clinical, and translational medical research, and is investigating the causes, treatments, and cures for both common and rare diseases. For more information about NIH and its programs, visit www.nih.gov .
About the Pennington Biomedical Research Center
The Pennington Biomedical Research Center is at the forefront of medical discovery as it relates to understanding the triggers of obesity, diabetes, cardiovascular disease, cancer and dementia. The Center architected the national “Obecity, USA” awareness and advocacy campaign to help solve the obesity epidemic by 2040. The Center conducts basic, clinical, and population research, and is affiliated with LSU.
The research enterprise at Pennington Biomedical includes over 480 employees within a network of 40 clinics and research laboratories, and 13 highly specialized core service facilities. Its scientists and physician/scientists are supported by research trainees, lab technicians, nurses, dietitians, and other support personnel. Pennington Biomedical a state-of-the-art research facility on a 222-acre campus in Baton Rouge.
For more information, see www.pbrc.edu .
Disclaimer: AAAS and EurekAlert! are not responsible for the accuracy of news releases posted to EurekAlert! by contributing institutions or for the use of any information through the EurekAlert system.
Additional Multimedia
Electronic Video Consent to Power Precision Health Research: A Pilot Cohort Study
Affiliations.
- 1 UCLA Center for SMART Health, Clinical and Translational Science Institute, David Geffen School of Medicine at UCLA, Los Angeles, CA, United States.
- 2 David Geffen School of Medicine at UCLA, Los Angeles, CA, United States.
- 3 Mayo Clinic, Rochester, MN, United States.
- 4 Embedded Clinical Research and Innovation Unit, CTSI Office of Clinical Research, Los Angeles, CA, US
- 5 Institute for Precision Health, David Geffen School of Medicine at UCLA, Los Angeles, CA, United States.
- PMID: 34313247
- PMCID: PMC8459215
- DOI: 10.2196/29123
Background: Developing innovative, efficient, and institutionally scalable biospecimen consent for remnant tissue that meets the National Institutes of Health consent guidelines for genomic and molecular analysis is essential for precision medicine efforts in cancer.
Objective: This study aims to pilot-test an electronic video consent that individuals could complete largely on their own.
Methods: The University of California, Los Angeles developed a video consenting approach designed to be comprehensive yet fast (around 5 minutes) for providing universal consent for remnant biospecimen collection for research. The approach was piloted in 175 patients who were coming in for routine services in laboratory medicine, radiology, oncology, and hospital admissions. The pilot yielded 164 completed postconsent surveys. The pilot assessed the usefulness, ease, and trustworthiness of the video consent. In addition, we explored drivers for opting in or opting out.
Results: The pilot demonstrated that the electronic video consent was well received by patients, with high scores for usefulness, ease, and trustworthiness even among patients that opted out of participation. The revised more animated video pilot test in phase 2 was better received in terms of ease of use (P=.005) and the ability to understand the information (P<.001). There were significant differences between those who opted in and opted out in their beliefs concerning the usefulness of tissue, trusting researchers, the importance of contributing to science, and privacy risk (P<.001). The results showed that "I trust researchers to use leftover biological specimens to promote the public's health" and "Sharing a biological sample for research is safe because of the privacy protections in place" discriminated opt-in statuses were the strongest predictors (both areas under the curve were 0.88). Privacy concerns seemed universal in individuals who opted out.
Conclusions: Efforts to better educate the community may be needed to help overcome some of the barriers in engaging individuals to participate in precision health initiatives.
Keywords: barrier; biobanking; cancer; consent; education; efficient; electronic consent; engagement; innovation; participation; pilot study; precision; precision medicine; privacy; video.
©Arash Naeim, Sarah Dry, David Elashoff, Zhuoer Xie, Antonia Petruse, Clara Magyar, Lilliana Johansen, Gabriela Werre, Clara Lajonchere, Neil Wenger. Originally published in JMIR Formative Research (https://formative.jmir.org), 08.09.2021.
Grants and funding
- UL1 TR001881/TR/NCATS NIH HHS/United States
NIH VideoCasting
CIT can broadcast your seminar, conference or meeting live to a world-wide audience over the Internet as a real-time streaming video. The event can be recorded and made available for viewers to watch at their convenience as an on-demand video or a downloadable file. CIT can also broadcast NIH-only or HHS-only content.
Clinical Center Grand Rounds: Great Teachers Distinguished Clinical Research Scholar and Educator in Residence Lecture - Genetics & Genomics of Neuropsychiatric Disorders
The event has not started yet...

Loading video...
Wednesday, April 10, 2024, 12:00:00 PM, 1 hour. viewers
Videocast send live feedback.
Clinical Center Grand Rounds: Great Teachers Distinguished Clinical Research Scholar and Educator in Residence Lecture - Genetics & Genomics of Neuropsychiatric Disorders

Research Data Analyst 1
🔍 school of medicine, stanford, california, united states.
The Stanford University Center for Precision Mental Health and Wellness is seeking a motivated and well-trained research data analyst to manage and analyze high dimensional neuroimaging and behavioral datasets acquired in precision mental health studies. Especially sought are candidates with expertise in functional imaging data analysis in human subjects studies.
The position will be based within Center’s Personalized and Translational Neuroscience lab. Studies in the lab use brain imaging approaches to improve our understanding and treatment of mental health conditions such as depression and anxiety. These studies are funded through the NIH and philanthropy.
The Research Data Analyst (RDA) will be tasked with managing incoming data, implementing data quality controls, visualizing data and generating and implementing analysis models for neuroimaging and behavioral data and their integration. Data are acquired at multiple time points, including pre- and post-treatment. The RDA will also be responsible for sharing data through NIH data coordinating centers. The tasks are supported by an existing database and server infrastructure, an large existing datasets.
The RDA will work in close collaboration with an interdisciplinary team. There is a need for flexibility to work across different scripting and software environments and display agility in learning. Members of the Center possess deep domain experience in their chosen fields and an ability to communicate across fields.
The position will be fully in person.
Interested candidates should include:
- A cover letter addressing requirements and why you are motivated to apply to this position and contribute to research in precision mental health and how your education and experience relate to the position as described above.
- Resume or CV
- At least three references to [email protected] . The position is open until filled. However, because of funding timelines and project milestones, we are seeking to fill this position as soon as possible.
The supervising PI for this position is Dr. Leanne Williams, https://profiles.stanford.edu/leanne-williams .
Learn more about the Lab and Center: http://med.stanford.edu/pmhw . The position will be based within a collaborative team that values cooperation, fairness, efficiency, and conscientiousness.
Specific Tasks include:
- Collect, manage and clean large datasets.
- Employ new and existing tools to interpret, analyze, and visualize multivariate relationships in human subjects data.
- Create databases and reports, develop algorithms and statistical models, and perform statistical analyses appropriate to data and reporting requirements.
- Use system reports and analyses to identify potentially problematic data, make corrections, and determine root cause for data problems from input errors or inadequate field edits, and suggest possible solutions.
- Develop reports, charts, graphs and tables for use by investigators and for publication and presentation.
- Analyze data processes in documentation.
- Collaborate with faculty and research staff on data collection and analysis methods.
- Provide documentation based on audit and reporting criteria to investigators and research staff.
- Communicate with grant agencies in regard to data sharing centers.
* - Other duties may also be assigned
DESIRED QUALIFICATIONS:
- Familiarity with fMRI data analysis techniques, such as using SPM, Freesurfer
- Experience with complex human subjects data management
- Knowledge of statistical and machine learning techniques for data analysis
- Experience working in research or academic setting
- Familiarity with data visualization tools
EDUCATION & EXPERIENCE (REQUIRED):
- Bachelor's degree or a combination of education and relevant experience. Experience in a quantitative discipline relevant to the domain of the research in the lab, such as psychology, neuroscience, or statistics.
KNOWLEDGE, SKILLS AND ABILITIES (REQUIRED):
- Motivation to contribute to advances in precision mental health
- Proficiency in statistical analysis software (e.g., SPSS, R, Python)
- Experience working with complex datasets
- Substantial experience with MS Office and analytical programs
- Strong writing, problem solving and analytical skills
- Ability to prioritize workload
- Ability to manage multiple projects and deadlines
PHYSICAL REQUIREMENTS*:
- Sitting in place at computer for long periods of time with extensive keyboarding/dexterity.
- Occasionally use a telephone.
- Rarely writing by hand.
- - Consistent with its obligations under the law, the University will provide reasonable accommodation to any employee with a disability who requires accommodation to perform the essential functions of his or her job.
WORKING CONDITIONS:
- Some work may be performed in a laboratory or field setting.
- The work arrangement for this position will be on-site and will be based on the Stanford main campus.
The expected pay range for this position is $64,480 to $97,000 per annum. Stanford University provides pay ranges representing its good faith estimate of what the university reasonably expects to pay for a position. The pay offered to a selected candidate will be determined based on factors such as (but not limited to) the scope and responsibilities of the position, the qualifications of the selected candidate, departmental budget availability, internal equity, geographic location and external market pay for comparable jobs.
* - Stanford is an equal employment opportunity and affirmative action employer. All qualified applicants will receive consideration for employment without regard to race, color, religion, sex, sexual orientation, gender identity, national origin, disability, protected veteran status, or any other characteristic protected by law.
- Schedule: Full-time
- Job Code: 4751
- Employee Status: Regular
- Requisition ID: 102819
- Work Arrangement : On Site
My Submissions
Track your opportunities.
Similar Listings
School of Medicine: Porter Drive, Stanford, California, United States
📁 Information Analytics
Post Date: Dec 14, 2023
School of Medicine, Stanford, California, United States
Post Date: 4 days ago
Post Date: Jan 11, 2024
Global Impact We believe in having a global impact
Climate and sustainability.
Stanford's deep commitment to sustainability practices has earned us a Platinum rating and inspired a new school aimed at tackling climate change.
Medical Innovations
Stanford's Innovative Medicines Accelerator is currently focused entirely on helping faculty generate and test new medicines that can slow the spread of COVID-19.
From Google and PayPal to Netflix and Snapchat, Stanford has housed some of the most celebrated innovations in Silicon Valley.
Advancing Education
Through rigorous research, model training programs and partnerships with educators worldwide, Stanford is pursuing equitable, accessible and effective learning for all.
Working Here We believe you matter as much as the work
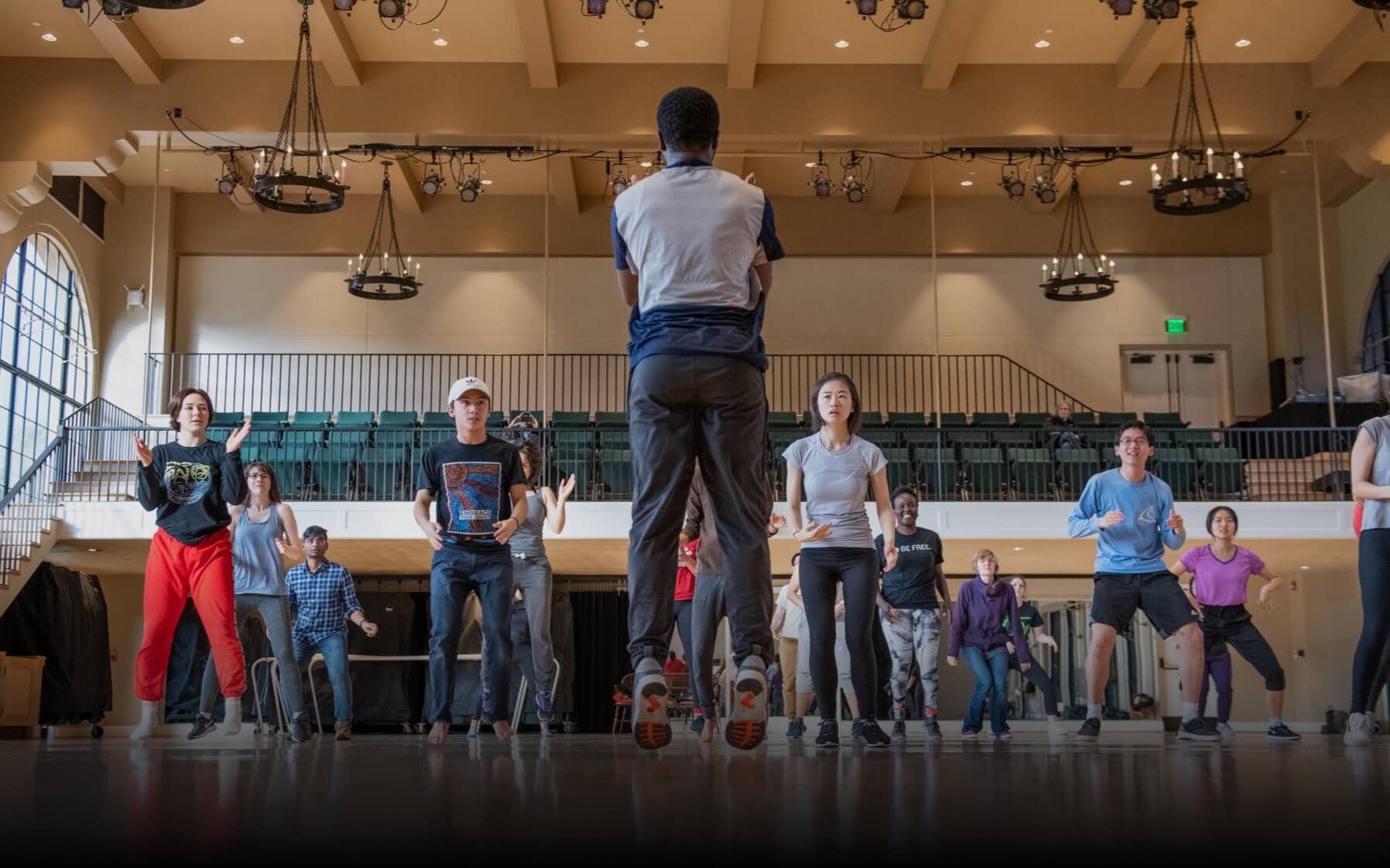
I love that Stanford is supportive of learning, and as an education institution, that pursuit of knowledge extends to staff members through professional development, wellness, financial planning and staff affinity groups.
School of Engineering
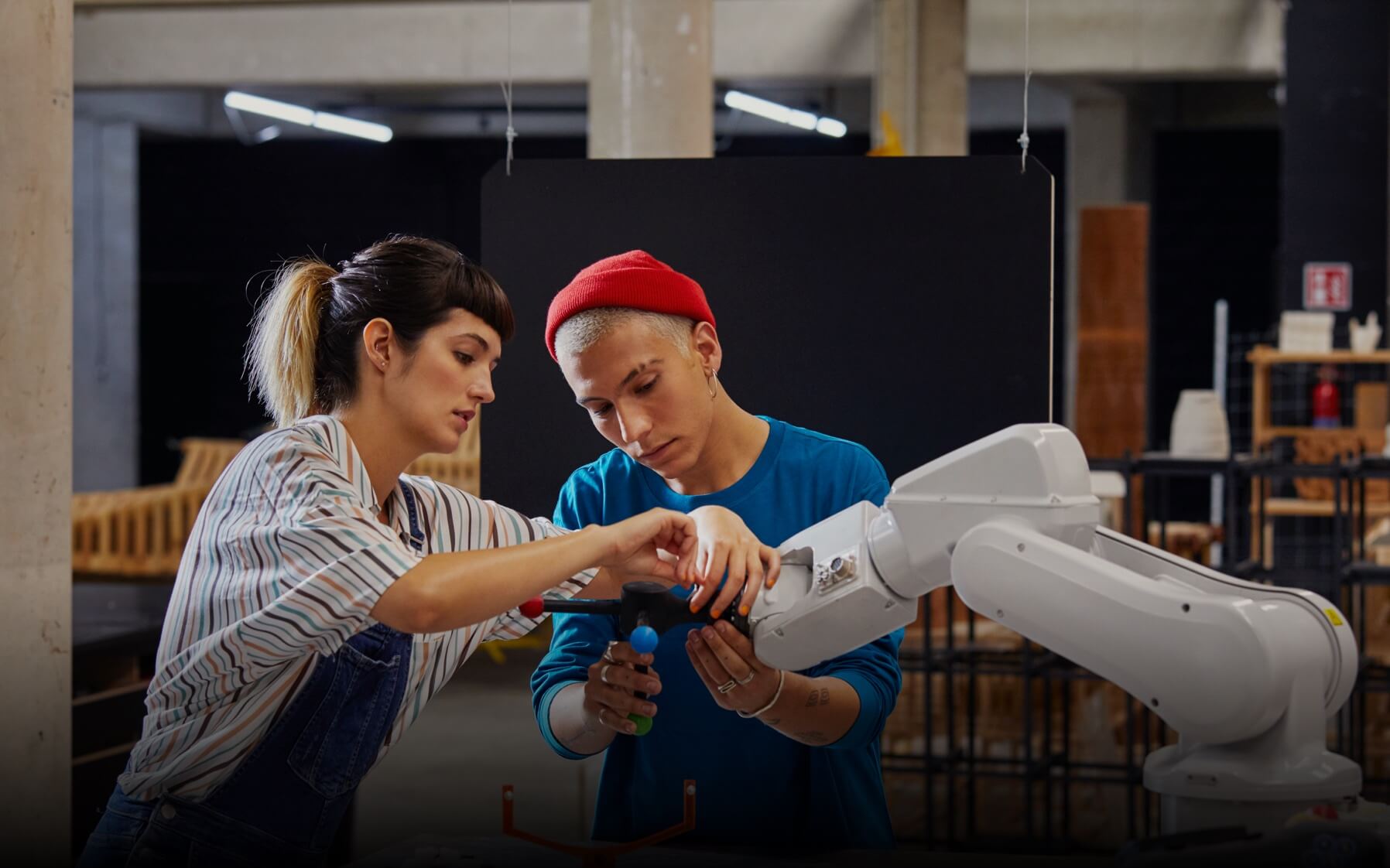
I get to apply my real-world experiences in a setting that welcomes diversity in thinking and offers support in applying new methods. In my short time at Stanford, I've been able to streamline processes that provide better and faster information to our students.
Phillip Cheng
Office of the Vice Provost for Student Affairs
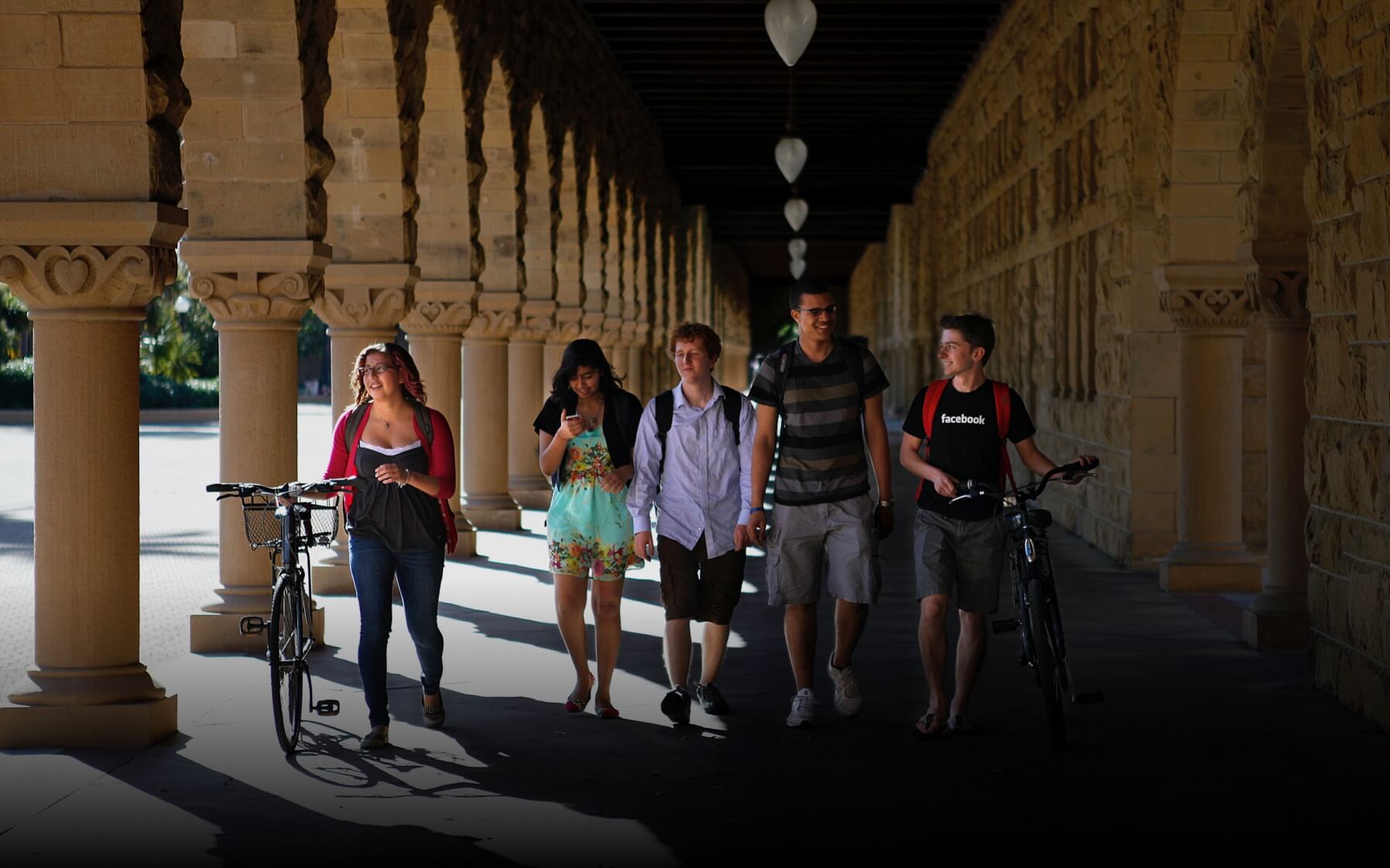
Besides its contributions to science, health, and medicine, Stanford is also the home of pioneers across disciplines. Joining Stanford has been a great way to contribute to our society by supporting emerging leaders.
Denisha Clark
School of Medicine
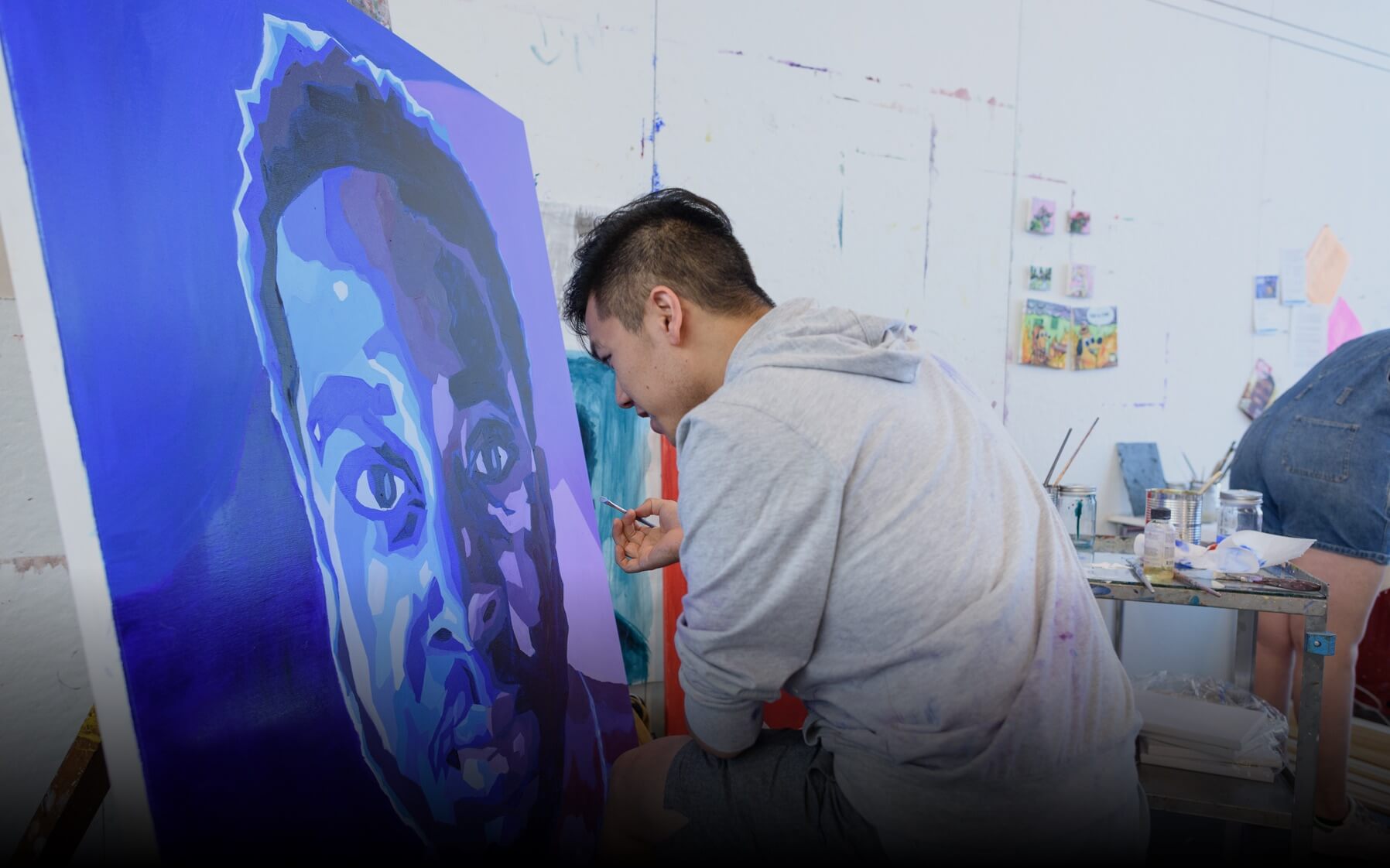
I like working in a place where ideas matter. Working at Stanford means being part of a vibrant, international culture in addition to getting to do meaningful work.
Office of the President and Provost
Getting Started We believe that you can love your job
Join Stanford in shaping a better tomorrow for your community, humanity and the planet we call home.
- 4.2 Review Ratings
- 81% Recommend to a Friend
View All Jobs

An official website of the United States government
The .gov means it’s official. Federal government websites often end in .gov or .mil. Before sharing sensitive information, make sure you’re on a federal government site.
The site is secure. The https:// ensures that you are connecting to the official website and that any information you provide is encrypted and transmitted securely.
- Publications
- Account settings
Preview improvements coming to the PMC website in October 2024. Learn More or Try it out now .
- Advanced Search
- Journal List
- PMC10147599
A precision environmental health approach to prevention of human disease
Andrea baccarelli.
1 Department of Environmental Health Sciences, Columbia University Mailman School of Public Health, New York, NY USA
Dana C. Dolinoy
2 Department of Environmental Health Sciences, University of Michigan School of Public Health, Ann Arbor, MI USA
Cheryl Lyn Walker
3 Center for Precision Environmental Health, Baylor College of Medicine, Houston, TX USA
Human health is determined by the interaction of our environment with the genome, epigenome, and microbiome, which shape the transcriptomic, proteomic, and metabolomic landscape of cells and tissues. Precision environmental health is an emerging field leveraging environmental and system-level (‘omic) data to understand underlying environmental causes of disease, identify biomarkers of exposure and response, and develop new prevention and intervention strategies. In this article we provide real-life illustrations of the utility of precision environmental health approaches, identify current challenges in the field, and outline new opportunities to promote health through a precision environmental health framework.
Precision environmental health leverages environmental and system-level data to understand underlying environmental causes of disease, identify biomarkers of exposure, and develop new prevention and intervention strategies. In this Perspective, the authors provide real-life illustrations of the utility of precision environmental health approaches and identify current challenges in the field.
Introduction
Our health is affected by the world around us—the climate in which we live, the air we breathe, the water we drink, and the food we eat all impact our health 1 . The broadest interpretation of our environment encompasses all aspects of the world that surrounds us, including our built, socioeconomic, chemical, physiological, and psychosocial environments 2 , 3 . These diverse components influence how all body systems function, constituting major, and often modifiable, determinants of human health 4 .
Environmental exposures contribute to an estimated 70–90% of the burden of human disease 5 . This relationship between the environment and health is the focus of environmental health science (EHS) research, which seeks to understand how the environment influences human health and promotes healthier lives. In the past, EHS research largely focused on defining and mitigating environmental hazards 6 . Modern EHS research also encompasses beneficial exposures such as essential trace elements, beneficial microbiota, and social support systems. Traditionally, EHS research was translated into action through strategies to reduce harmful exposures, for instance, through policy or technological advances. However, these traditional approaches have limited capacity to consider everyone’s unique combination of type, level, and timing of exposures that, combined with their genetic and patho- and physiological states, are likely to produce distinct health outcomes in each of us. Precision environmental health seeks to tackle this challenge by increasing our understanding of exposures over the lifecourse and determinants of individualized response to these exposures. Identification of individuals at greatest risk for, or who are disproportionately impacted by, diseases linked to environmental exposures is key to enabling precise, targeted, and effective prevention and intervention strategies (Box 1 ).
The concept of precision environmental health is based on an appreciation that the impact environmental exposures have on health differs among individuals and across populations and time 7 , as well as across life stages 8 . Large interindividual differences exist in genomic, epigenomic, and other molecular and lifestyle factors that contribute to health and risk of disease. Specific life stages, such as during embryo and fetal development, early postnatal life, or aging, have been proposed as discrete windows with higher vulnerability 8 . Environmental exposures themselves are unevenly distributed across populations due to geographic, socioeconomic, and other demographic factors 9 , 10 , with patterns of concern influenced by environmental injustice and inequity. However, achieving the promise of precision environmental health will require new knowledge, computational approaches, and technologies to move into clinical and public health practice (Box 2 ). All of these will be needed to evolve our understanding of the relationships between environmental exposures and health and translate that understanding into effective, targeted, and precise real-life applications 11 – 13 .
Box 1 The future of precision environmental health
Imagine a future when you could take a simple blood test to determine if you have been exposed to chemicals in the environment that can harm your health. Your healthcare provider would then offer solutions to ameliorate that exposure and/or prevent its adverse health effects. Or imagine a personal monitoring device that can reveal how you are responding to fluctuations in your environmental exposures caused by climate change. While climate change is global, each of us may be impacted differently, for example, by temperature extremes or inhalation exposures from drought-provoked fires, and will have unique responses. Precision environmental health offers the promise to understand and identify these types of individualized responses and provide precision interventions to improve health and prevent disease.
Box 2 The promise of precision environmental health
Precision environmental health seeks to promote health and reduce or eliminate the adverse health effects of environmental exposures while maximizing positive health influences 1 . Current clinical and public health approaches to achieve this goal have not yet fully utilized system-level data-omic approaches to characterize environmental exposures and design strategies to mitigate risk at the individual level 9 . These exposures, sometimes referred to as the “exposome” contribute to an individual’s allostatic load, or cumulative burden of chronic stress and life events. A precision environmental health approach leverages genomics, epigenomics, and other ‘omics, as well as knowledge of the many environmental exposures encountered across the lifecourse. Combining these types of omic-level data holds promise for precise, individualized health promotion and prevention of diseases linked to environmental exposures.
Precision environmental health toolbox
While precision medicine originated primarily from genomics 14 , precision environmental health increasingly focuses on other targets. Genetics has a significant impact on health, but the genome remains largely static throughout an individual’s lifetime. In contrast, other substrates, such as the epigenome or microbiome, are both a target and a determinant of response to environmental exposures. Often environmental exposures leave imprints on these substrates, like fingerprints, providing opportunities to capture previous exposures and predict future risks of disease 15 . This has led to an appreciation of the need to capture data from multiple ‘omic layers using an integrated, data-driven approach to precisely assess how environmental exposures impact each individual, identify adverse or beneficial environmental factors, and develop precisely targeted interventions to improve health and prevent disease. These ‘omic layers include:
Genomics and epigenomics
The Human Genome Project identified ~3 billion human bases and 20,000 human genes, including ~10 million single nucleotide polymorphisms (SNPs). Genomic technologies, along with mechanistic genome editing platforms such as CRISPR 16 , can now determine which genetic variables are most responsible for an individual’s response to their environment. Precision environmental health research continues to leverage these advances and incorporate genomics into individualized assessments of susceptibility to environmental exposures. For example, genetic susceptibility factors that contribute to disease risk have clear utility as susceptibility biomarkers 17 .
However, the genome is static and non-modifiable, and therefore has little utility for use as a record of previous environmental exposures in non-diseased tissue or as a target for interventions to reduce the risk of environmental disease. In contrast, the epigenome is potentially modifiable and thus has become a focus in precision environmental health for its potential as a reporter and biomarker of past exposures, as well as a target for interventions 18 , 19 . Literally meaning “above the genome”, the epigenome encompasses heritable modifications, such as DNA and histone modifications and changes in chromatin conformation, that affect gene expression without altering the underlying DNA sequence 20 . Traditional molecular epidemiology has viewed how the environment affects health through the lens of the genome–environment interactions (GxE) 21 , or how an individual’s genetics influences their response to the environment. For example, individual differences in metabolizing enzyme polymorphisms that alter chemicals’ toxicity can, in the case of dietary carcinogens, change their potency to influence the risk of developing cancer 22 , 23 . Now, the field is moving beyond GxE to study interactions between the environment and other system-level substrates, such as the epigenome. Hundreds of in vitro, animal, and human studies over the past decade have now established the importance of epigenome x environment (ExE) interactions. An example of an ExE interaction is exposure to lead and DNA methylation. Data on lead levels from pregnant mothers and DNA methylation from their infants from the Early-Life Exposure in Mexico to Environmental Toxicants (ELEMENT) project show that environmental lead exposures are associated with infant DNA methylation changes in LINE-1 transposable elements 24 . While ELEMENT data do not link those epigenetic changes to childhood outcomes, a separate study does—childhood obesity data from the Healthy Families Study showed that LINE-1 methylation associates with obesity status during childhood 25 .
Transcriptomics and epitranscriptomics
Transcriptomic studies using next-generation sequencing can comprehensively profile protein-coding (mRNAs) as well as non-coding RNAs (e.g., microRNAs, long non-coding RNAs, tRNAs), which play an equally important role in the cell as coding RNAs. Most recently, epitranscriptomics 26 —a study of the regulation and function of post-transcriptional RNA modifications—has emerged as an important link between environmental exposures and disease 27 – 31 . Studies in various model systems show that environmental stressors can change RNA modifications and reprogram regulatory RNAs 32 . Additional research on the impact of environmental exposures on RNA processing and modifications is needed to understand the mechanisms by which exposures perturb RNA, and to link this biology to environmental causes of human disease. In this context, exosomes with targeted RNA cargos 33 , may also provide new approaches to assessing exposure and response, and as tools for interventions 34 , 35 . Exosome cargos include non-coding RNAs that are responsive to environmental stressors 36 , 37 . Exosomes and their contents can be engineered to deliver specific cargoes to target tissues, including sense or antisense RNAs that can reverse deficits or counteract overexpressed RNAs in those tissues 38 .
Proteomics is the analysis of many, and potentially all, proteins in a specimen 39 . The development of proteomic biomarkers and the ability to detect early protein-level changes in response to environmental exposures holds the potential for the prevention of environmentally induced adverse health effects 40 . For example, proteomic studies have discovered 16 proteins, many related to cardiovascular disease and function, associated with low-level mercury or lead exposure 41 , and protein biomarkers have been identified for fluoride exposure in children 42 . Shotgun proteomic methods [e.g., gel electrophoresis liquid chromatography–tandem mass spectrometry (GeLC-MS/MS)] can identify proteins that are depleted or increased in response to environmental exposures 43 . Additionally, LC-MS/MS-based methods to characterize posttranslational modifications (PTMs) can also be biomarkers if specific PTMs are significantly associated with exposure or toxicity 44 . However, implementing these technologies requires specialized expertise, and often, expensive instrumentation, and targeted proteomics is much further advanced than our current ability to identify all proteins in a specimen. This is further exacerbated by the importance of proteoforms, which is the specific combination of PTMs on a single protein molecule, which provide a level of resolution and information not yet routinely available.
Advances in understanding the role of microorganisms in health and disease brought attention to the microbiome as a mediator of environmental health and as a potential target for interventions 45 , 46 . Indeed, the microbiome is a key interface between exogenous and endogenous exposures and health. It can be modified by environmental exposures, affect health, and even be used in interventions to prevent environmental disease 47 . Some microbes alter environmental substances and make them more toxic, while others make environmental substances less harmful. The microbiome, because it can be modified by simple interventions, including nutritional interventions or probiotics, holds special promise in identifying new approaches to prevent the harmful and amplifying beneficial effects of environmental exposures, for example, to promote cardiovascular health 50 . The interplay of environmental exposures, the microbiome, and the epithelial barrier has also been proposed to underlie the observed increase in allergic and autoimmune disease 48 , 49 . Metagenomic analyses are key to unlocking environment x microbiome interactions: ribosomal 16 S sequencing can provide information on changes in bacterial composition in a sample, whereas whole genome shotgun (WGS) sequencing provides genome coverage of all organisms in a sample and greater taxonomic (read: functional) resolution.
Despite the wide availability of metagenomic sequencing, more knowledge is needed about crosstalk between the microbiome and other “omic” layers, for example, the metabolome, epigenome, and microbiome. Short-chain fatty acid (SCFA) metabolites, such as acetate and butyrate, are exclusively derived from microbial fermentation of dietary fiber 50 . SCFAs provide the acyl groups needed for several epigenetic histone modifications and are the acyl donors required for the activity of histone acetyltransferases 51 – 56 . Thus, SCFA production by the microbiome is required for normal epigenomic programming, and when deficient or perturbed by environmental exposures, has been shown to cause reprogramming of the epigenome in multiple tissues 57 , 58 Investigating the microbiome-metabolome interplay is critical to toxicology and environmental health. As an example, Lu et al. showed that C57BL/6 mice treated with arsenic have concurrent changes in both the gut microbiome and urine metabolomics 59 . Gao et al. provided a prototype of human multi-omic studies that included high-resolution untargeted exposome-scale analysis of chemicals and metabolites in plasma and gut microbiome analysis, as well as genomic and transcriptomic analyses 11 . This study demonstrated that both time and location impacted all the omic levels investigated and their highly dynamic interplay 11 .
Metabolomics
Metabolic homeostasis is intricately regulated and sensitive to environmental conditions, and metabolites can provide insight into the body’s dynamic response to diet, exercise, pharmaceutical use, and exposure to chemical stressors 60 . Metabolic profiling can, for example, provide signatures for and identify responses to environmental exposures as varied as air pollution, persistent organic pollutants, proximity to industrial operations, metals, perfluorinated substances, plasticizers, and climate-related variables 61 – 63 . An extensive array of metabolic measurements (e.g., targeted metabolomics panels of citric acid cycle intermediates, amino acids, acylcarnitines, free fatty acids, targeted lipidomics, multiplexed hormone analysis) and untargeted metabolomics are possible. However, not all chemical metabolites are persistent, and metabolic perturbations in response to environmental exposures are often labile, and both can be challenging to capture. However, metabolic analyses are becoming more readily available in institutional core facilities and commercial laboratories, and through for-profit ventures, facilitating their increasing use in EHS and precision environmental health research.
The “exposome” represents a framework to study environmental drivers of health and disease 64 . The ultimate goal of exposomic science is to accurately define the totality of an individual’s chemical and non-chemical exposures (e.g., toxicants, diet, physical activity, and psychosocial stressors) over the lifecourse, including prenatally 64 . Rapid developments in analytical chemistry and other technologies provide highly sensitive, specific information on a wide range of external and exogenous exposures. For example, newer mass spectrometry technology enables untargeted screening of hundreds of chemicals at once in biological samples 65 . Also, the increasing abundance of environmental geospatial data, including air pollution, noise, and the natural, built, and social environments, allows for comprehensive characterization of external exposome factors 66 . However, the field continues to face numerous challenges in operationalizing the exposome, as the ideal goal to measure all exposures continuously throughout someone’s lifetime is still elusive.
Data science approaches
Advancing risk stratification and disease prevention using genomic, epigenomic, exposomic, proteomic, and metabolomic data will require data science approaches that integrate large datasets across multi-omic platforms. In this regard, artificial intelligence, which includes machine learning (ML) and natural language processing, can identify patterns in complex multidimensional datasets that include measurements of environmental exposures, and then apply that knowledge to identify individuals or populations most at-risk for adverse outcomes. ML is particularly well suited to unlocking knowledge and detailed information from semi-structured or unstructured data for precision environmental health, because time-dependent and high-throughput genome-scaled data types, such as epigenomic, transcriptomic, proteomic, or metabolomic data, are similar in structure. Some approaches are completely data-driven, only making the general assumption that the data will identify subgroups of individuals with characteristics of interest. For instance, ML approaches can help to differentiate cases and controls or to identify individuals with higher exposures and/or at increased risk of developing a certain disease. Hence, ML may reveal patterns that can inform personalized approaches that might not be discoverable using traditional statistical techniques 67 .
Because precision environmental health adds another dimension—the environment—to already high-dimensional data, robust data science approaches become even more critical to tease out the impacts of environmental exposures and develop approaches for risk stratification. As environmental exposures can affect biological processes at many different levels that contribute to disease, integrating multi-omic inputs in precision environmental health research can yield more comprehensive and accurate insights into how exposures impact health, for example, by moving beyond phenotype to endotype 68 , 69 (Box 3 ). Approaches that allow for modeling the interactions among different “omic” data, such as the “interactome”, which uses a more multi-axial systems approach to identify the relevant networks that underlie environmental-driven diseases 70 , may provide additional advantages. Incorporating mechanistic understandings of how exposures contribute to disease, their associated molecular responses (endotypes), and “omic-based” determinants of disease risk, will enable the identification of target populations and individuals who will most benefit from targeted interventions—enabling a precision approach to disease prevention.
Box 3 Moving beyond phenotype to endotype
Disease phenotypes are identifiable using a common rubric of key attributes such as clinical parameters and physiological characteristics. However, phenotype can be driven by different molecular mechanisms with disparate etiologies. Precision environmental health, by linking, for example, underlying individual risk factors and exposures contributing to disease pathogenesis with specific molecular (or other) endotypes, can provide an additional level of understanding of disease etiology, mechanisms of pathogenesis, and, potentially, new prevention and intervention strategies.
Hallmarks of precision environmental health research
Precision environmental health has demonstrated its utility for the development and delivery of interventions and/or prevention of disease tailored to the individual in four key areas (Fig. 1 ).

Precision Environmental Health seeks to prevent disease by understanding risk and tailoring interventions at the level of the individual. To reach this goal, precision environmental health research seeks to understand mechanisms by which environmental exposures cause disease and the basis for interindividual differences in susceptibility. This information will contribute to the attainment of this goal through the development of more precise biomarkers of exposure and risk and the development of advanced risk prediction models that incorporate “omic” data.
Elucidating the complex mechanisms by which environmental exposures cause disease
Cells respond in diverse ways to environmental exposures 12 . Some of these responses, such as increased expression of metabolic genes to rapidly metabolize chemicals, directly reduce the impact of external exposures 5 , 21 – 23 . However, these adaptive responses also may cause adverse effects 12 . For instance, our body responds to a peak of air pollution exposure by mounting inflammatory responses locally in the lung and systemically to help clear air pollutants 71 . However, high repeated exposure and chronic exposures that induce a sustained inflammatory response can cause increased oxidative damage, a contributing factor to multiple diseases, including chronic lung disease, atherosclerosis and cardiovascular disease, accelerated cognitive aging, and dementia 3 , 72 – 74 . These responses are driven by a cascade of molecular, biochemical, and cellular changes that can be captured by various ‘omics approaches 12 . For instance, genetic polymorphisms may determine the robustness of an individual’s inflammatory response to air pollution, and how inflammatory genes are activated in response to air pollution can be influenced by an individual’s epigenome, which in turn controls changes in the transcriptome and the production of specific inflammatory proteins 75 . This single example illustrates how knowledge gained from genomic, epigenomic, transcriptomic, and proteomic data can help characterize responses to environmental exposures.
Other examples where the application of “omics” technologies are providing new insights into how environmental exposures contribute to the human disease include ExE interactions. Reprogramming of the epigenome by environmental exposure can persist long after the actual environmental exposure, sometimes even across multiple generations, and result in changes in the transcriptome, proteome, and metabolome 76 , 77 that can drive adverse health outcomes. Therefore, while a variety of omics are influenced by the environment and mediate disease 78 , ExE interactions hold special promise for understanding how past exposures, especially those occurring early in life, influence the risk of disease later in adult life.
Studies in vitro and model organisms can also contribute mechanistic information linking exposures and adverse effects. While clinical sciences typically rely on randomized controlled trials to define the effects of treatment, comparable exposure–outcome trials are usually not ethically feasible 79 . For example, if observational data link prenatal pesticide exposure to adolescent cognitive deficits, one cannot design an ethical trial to expose pregnant women to that pesticide to determine if the relationship is causal. An alternative is to perform human intervention trials to remove the exposure 80 , 81 , a converse approach that can still reveal relevant information about the exposure–response relationship. However, some exposures are ubiquitous, and derived from multiple, widespread sources, making them challenging to control through simple interventions. Yet without causal data, how can observational data provide sufficient evidence to limit an exposure?.
A possible path toward deriving mechanistic links and causality stems from sourcing correlation and mechanistic/causation data from separate complementary approaches rather than relying on just one type of study to supply all the answers. While human observational studies can provide rich data on the responses or targets of real-world environmental exposures, in vitro and in vivo experiments can provide similar insights from more defined, controlled exposures. Carefully controlled experiments in cell and animal models enable investigators to probe more deeply into the mechanisms by which exposures influence responses, which may not be feasible in population studies. By combining analyses from these parallel approaches, common molecular targets can be cross-referenced across population and laboratory studies, yielding a powerful strategy to mechanistically understand how real-world exposures influence health outcomes (Fig. 2 ).

While human cohort studies can examine associations between environmental exposures and health outcomes, only surrogate tissues are available to assess molecular mechanisms involved in complex biological functions. Animal models can provide additional mechanistic insight by allowing for multi-tissue and multi-age comparisons. Animal models and in vitro cell culture studies can provide both mechanistic insights and a basis for moving beyond correlation to causality via reverse translation. Red people mean “unaffected”; blue people mean “susceptible”. Created with BioRender.com.
Predicting the risk caused by environmental exposures
Understanding the basis of interindividual differences in susceptibility.
Current approaches in environmental health do not adequately incorporate emerging knowledge about how environmental exposures can alter individual disease risk 82 . For example, while the entire population of a large metropolitan area is exposed to the city’s air pollution, a relatively small proportion of individuals will develop diseases associated with air pollution exposure, such as cardiovascular disease, respiratory disease, or cancer 71 , 72 . Yet, we currently have limited means to ascertain individual risk or subpopulations disproportionately and adversely affected by air pollution exposure 83 . To lessen the burden of disease in response to this, and other adverse environmental exposures, we must identify those at greatest risk well before clinical effects are detectable to maximize preventive efforts.
Developing biomarkers of exposure and disease risk
Laboratory advances in exposome assessment have been powered by high-resolution mass spectrometry platforms that can measure many hundreds of exogenous chemicals, in addition to endogenous metabolites, in biological samples (e.g., blood, saliva, and urine) 84 . Operationalizing the exposome will be a major step toward fulfilling the promise of precision environmental health. While data continue to reveal the importance of the exposome, capturing exposures across the lifecourse is challenging 85 . Environmental exposures vary across time and tissues and, in most cases, must be sampled longitudinally (e.g., multiple times in a single day, across life stages) to accurately measure an individual’s exposure. Geospatial methods developed to characterize the external exposome factors are one approach to meet this challenge, and can model place-based characteristics of the environment over space and time to ideally estimate exposures such as air pollution, built environment, temperature, and noise, at any location and time. The growing capacity for personal monitors of environmental exposure (chemicals, pollen, extreme weather events, etc.) to collect real-time exposure data can provide opportunities for “personalized” decision-making (e.g., avoid an area with higher exposure, increase/decrease medication dose, contact healthcare providers, etc.). Other tools, such as the use of “digital twins”, already being adopted in precision medicine 86 , may prove equally useful in integrating the exposome into precision environmental health. Further, when capturing the exposome is not feasible, the evaluation of persistent epigenetic alterations may be possible as a proxy for exposures, and provide useful information for personalized decision-making. However, tools and applications are still limited and often constrained by the scarcity of data needed for evidence-based decision-making.
To this end, the Exposome-Explorer is a major advancement that offers the first database dedicated to biomarkers of exposure to environmental risk factors, including dietary factors, pollutants, and contaminants measured in population studies 87 , 88 . Exposome-Explorer has collected detailed information on the nature of biomarkers, their concentrations in various human biospecimens, the study population and the analytical techniques used for measurement, correlations with external exposure measurements, and data on biological reproducibility over time. This information can be used in precision environmental health research to compare the performance and field of application of various biomarkers and to identify the specific biomarkers or panels of biomarkers that are most useful for biomonitoring or disease etiology studies. Further, technologies that can measure and assess response to exposures at the molecular, protein, and metabolite levels are providing new opportunities to develop biomarkers of response to the same exposures. Combining knowledge of the mechanisms by which environmental exposures cause disease with exposure assessment and individual susceptibility factors can provide biomarkers of disease risk that can be applied to individuals and across populations.
Epigenetic biomarkers have also proven useful, as alterations caused by environmental exposures may persist long after the exposure ends, in some instances for a lifetime. Hence, epigenomic profiling can also provide both a record of past exposures and biomarkers for the risk of adverse health effects from environmental exposures at the level of the individual. One hurdle for incorporation of ExE studies in molecular epidemiology is the reliance of these studies on cells present in non-invasive biological samples, such as saliva, urine, stools, and meconium or biospecimens that can be obtained through minimally-invasive collection of blood, sputum, buccal and nasal epithelium from the respiratory tract, placenta and umbilical cord tissues, and breast milk 89 – 91 . Since the genome of cells present in specimens is identical to that of other cells across the human body, cells in those samples prove good surrogates for genetic studies. However, the epigenome is highly tissue- and even cell-type-specific 92 , 93 . Whether epigenetic alterations that occur in surrogate tissues in response to environmental exposures provide correlative information on the impact of these exposures on the epigenome of target tissues for the disease remains unclear.
To help assess the relationship between epigenomic alterations induced by the environment in target and surrogate tissues, the National Institute of Environmental Health Sciences (NIEHS) recently launched the TaRGET II: Environmental Epigenomic Analysis in Tissue Surrogates Consortium 94 (Box 4 ). TaRGET II explores whether correlative epigenetic signatures induced by environmental exposures occur in both surrogate and target tissues using mouse models. Ultimately, this consortium intends to provide epigenomic data to help design and interpret epidemiology studies following inaccessible target tissues with available surrogate tissues. The success of these TaRGET studies will be key to developing future practical epigenetic applications for precision environmental health.
Epigenomic alterations in response to the environment not only have the potential to serve as reporters of past environmental exposures, but, as the epigenome is modifiable, opens the possibility of targeted epigenetic interventions. Recent developments in techniques that allow selective manipulation of a cell’s epigenome 95 provide opportunities to use epigenetic editing to identify causal linkages for ExE interactions that drive disease and open possibilities for designing epigenetic interventions to prevent and treat environmental disease. However, challenges remain—existing methods for epigenetic editing can be globally indiscriminate and have significant off-target effects, or in the case of exogenous DNA, can be limited by the size of DNA recognition motifs 26 , 96 . The use of Piwi-interacting RNA (piRNA) begins to address these challenges 97 , 98 . piRNA is an endogenous mechanism for silencing genes and transposons at the transcriptional level, and can act permanently and acutely, unlike silencing using miRNA/siRNA, which act at the translational level and require repeated treatment.
Advanced risk prediction using multi-omic data
Precision environmental health seeks to provide a more accurate and individualized prediction of disease risk 99 . Combining knowledge of the environmental “riskscape”—which captures environmental exposures, the built environment, and demographic risk factors such as sex, race/ethnicity, and socioeconomic status—with knowledge of genetic, epigenetic, and lifestyle determinants of disease holds promise to more precisely assess individual risk and identify the most vulnerable individuals in a population, for example, to understand maternal and child health disparities 100 . Environmental exposures may have moderate to weak effects, and do not act in isolation to cause disease. Rather most exposures occur as mixtures, and, as discussed above, interact with multiple substrates and have “omic” effects on multiple substrates. This in contrast to single-gene disease-causing genetic mutations, which have strong and easy-to-identify effects 101 . However, despite these complexities, because environmental exposures influence everyone 102 , there is massive potential for precision environmental health to advance risk prediction and prevention strategies focused on environmental contributors to disease.
Population genetics has shown that disease risk is seldom determined by defects in only one or two genes. Rather, most common diseases involve multiple low-risk alleles and/or defects in genes that perturb multiple pathways. In genetics, this knowledge prompted the development of polygenic scores to predict disease risk, which combines multiple genetic variations into a single score that can help identify individuals at higher risk of disease. Analogous to polygenic contributions to disease, the adverse impacts of environmental exposures also could be quantified by creating data-driven “risk scores”. Such risk scores could be developed by integrating multi-‘omic inputs, including exposomics, genomics, epigenomics, metabolomics, proteomics, and metagenomic data, to reveal mechanistic information, contribute to the understanding of individual susceptibility to exposures, and inform risk prediction. Stratification based on such risk scores could then be used to identify those who will most benefit from targeted interventions to mitigate their exposures or ameliorate their adverse health effects—enabling a precision approach to disease prevention.
Together, these four hallmarks form the cornerstones for precision environmental health research. However, while research in these areas continues to advance, their application to public health practice is still constrained by the need for further information about their practical utility as well as challenges to large-scale implementation, as discussed below.
Precision environmental health and disease prevention
Here we discuss opportunities for precision environmental health in disease prevention and outstanding challenges to bringing these approaches to fruition. Precision environmental health can provide a targeted approach to prevent disease by focusing exposure mitigation, interventions, and treatments at the level of the individual, and to those most vulnerable. Such strategies can be incorporated into primary, secondary, and tertiary prevention 103 (Fig. 3 ).

Precision environmental health research can contribute to primary prevention (left) by mitigating individual risk, secondary prevention (middle) by slowing the progression of subclinical disease, and tertiary prevention by reducing disease impact (right). Colors are used to indicate diversity within exposed populations. Created with BioRender.com.
Primary prevention
It refers to preventing the initial occurrence of disease, including limiting exposures to reduce the overall disease burden. Rather than an untargeted population-agnostic approach, precision environmental health approaches could discern who is at greatest risk of disease, for example, by establishing biomarkers that inform how specific exposures (or mixtures of exposures), will impact an individual based on their genome, epigenome, and microbiome.
An example of a potential precision environmental health tool for primary prevention comes from ref. 104 , who analyzed data from the Normative Aging Study to identify DNA methylation-based biomarkers that discern individuals with high levels of past lead exposure (Fig. 4 ). DNA methylation has practical advantages as an epigenetic biomarker as it can be measured on biospecimens obtained with a variety of collection methods (e.g., standard blood draw, dried blood spot, saliva kept at room temperature, etc.) and can be applied to archival samples using standard storage methods. In identifying differentially methylated regions associated with lead levels in patella and tibia bones, the authors were able to assess cumulative lead exposure (the elemental half-life of lead extends beyond a decade in bone). Correlating these lead measurements with methylation patterns measured in blood identified a signature that not only served as a surrogate measure of lead levels in bone, but also cumulative lead exposure across time. This signature could serve as a simple biomarker—much easier than obtaining measures of bone lead, which requires highly specialized equipment—to identify individuals for targeted interventions to ameliorate the long-term health effects of lead exposure, which include adverse cardiovascular, neuro-cognitive, and renal system effects.

Epigenome-scale blood methylation data were used to train and test against benchmark bone-Pb data, which reflect long-term Pb exposure and were available in the study. This type of epigenetic biomarker holds great promise to capture and accurately assess long-term, cumulative exposures to environmental and lifestyle factors. Created with BioRender.com.
Such surrogate biomarkers hold great promise for high-throughput screening of potentially at-risk populations and for guiding intervention efforts to those most susceptible; however, more information is needed about the applicability of these biomarkers to other exposures and populations, and the cost-effectiveness of such screening when applied across the population. While DNA methylation is easily assessed from a drop of blood, most DNA methylation platforms are designed for research laboratories and do not yet have the scalability and cost of typical clinical tests. This illustrates that while precision environmental health approaches can have great utility, research, and technological advances are still needed to support their broad adoption.
Secondary prevention
It offers opportunities to mitigate exacerbating effects of environmental exposures once the disease is initiated but still preclinical, by promoting early disease detection and providing targets to impede the environmental promotion of disease progression 105 . A precision environmental health framework could also guide screening efforts to target resources to those with disproportionately higher risk based on their environmental exposures. An example of the applicability of precision environmental health to secondary prevention comes from the Trial to Assess Chelation Therapy 2 (TACT2) study 106 , an ongoing clinical trial examining the outcomes of EDTA chelation therapy combined with oral vitamins and minerals to treat patients with diabetes who have experienced a previous cardiac event. The initial study, TACT1, found a reduction in recurrent cardiac events following a myocardial infarction when EDTA chelation therapy to remove lead and cadmium was combined with oral vitamins, with a particularly profound effect in a subset of the cohort who also had diabetes 107 , 108 . TACT2 is testing replication of these findings in a larger, diabetes-focused cohort and could lead to blood lead and urine cadmium levels being developed as biomarkers to screen cardiac patients who may benefit from EDTA chelation therapy.
Tertiary prevention
It aims to reduce disease symptoms and patient morbidity/mortality to improve an individual’s quality of life even in the context of established clinical disease 109 . Precision environmental health in the setting of tertiary prevention could, for example, help protect patients most susceptible to environmental triggers of morbidity/mortality. Exposure to PM 2.5 (i.e., fine air pollution particles with aerodynamic diameter ≤2.5 μm) exemplifies this point—while PM 2.5 exposure has negative health effects across the population, those effects become particularly dangerous in individuals with asthma, whose airways are inflamed, constricted, and susceptible to insult 83 . Because asthma is an umbrella term comprising various subgroups that require different therapies and management, precision environmental health can help identify asthmatics who may have worse exacerbation from PM 2.5 exposures and might benefit most from interventions. These individuals, for example, might benefit most from avoiding areas with higher ambient exposures or reducing indoor levels in home, work, and school environments 110 .
Box 4 Toxicant exposures and responses by genomic and epigenomic regulators of transcription (TaRGET) consortium 26
Launched by NIEHS, TaRGET I explored how adverse environmental exposures impact the epigenome. TaRGET II established a multi-institution consortium to validate the robustness and feasibility of using surrogate tissues (e.g., peripheral blood lymphocytes) to detect epigenetic reprogramming by early-life exposures in mouse models. The third phase, TaRGET III, will support the translation of epigenomic data from the mouse- and cell-based studies to human population-based studies with available epigenomic data. The fourth phase, TaRGET IV, will support integrated analyses in population-based studies, using several genomic and epigenomic databases to develop more comprehensive analyses.
Precision environmental health holds great promise for enhancing our understanding of how the environment influences human health. Integrating environmental exposures across the lifecourse with large “omic” datasets using data science approaches, a precision environmental health framework has transformative potential for the development of precise and effective disease prevention. However, bringing precision environmental health into the realm of clinical and public health applications will require more action. Clearly, to realize the promise of precision environmental health will require training a workforce with the skills needed to work at the interface of the environment, the “omic” sciences such as genomics and epigenomics, and data science. In addition, the development and application of a precision environmental health framework will require additional ‘omic inputs from population, model organism, and in vitro systems, and technological refinements to translate knowledge and techniques from the laboratory to health practice. Most important, success will depend not only on scientific and technological advances but also on society’s capacity to incorporate advances in precision environmental health into public health strategies and individual health decision-making.
Acknowledgements
The authors would like to thank Rachel Morgan, Jessa Ehlinger, Katelyn Polemi, and Dr. Durga Tripathi for their valuable assistance in referencing this review.
Author contributions
All authors contributed to creating the Perspective’s concept and structure. All authors wrote, edited, and reviewed the text. A.B., D.C.D., and C.L.W. contributed to the original idea, discussions, manuscript writing, and figure design.
Peer review
Peer review information.
Nature Communications thanks Ioana Agache and Cathrine Hoyo for their contribution to the peer review of this work.
Competing interests
The authors declare no competing interests.
Publisher’s note Springer Nature remains neutral with regard to jurisdictional claims in published maps and institutional affiliations.
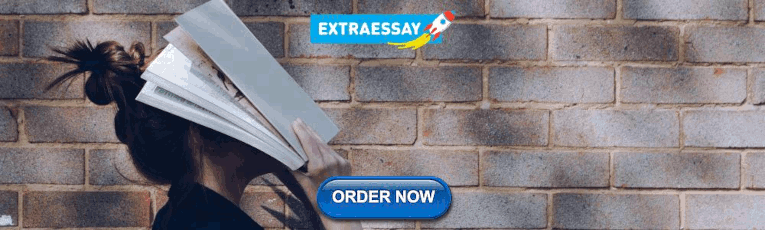
IMAGES
VIDEO
COMMENTS
The Center for Precision Health Research (CPHR) was established in 2021 as a research group within NHGRI's Intramural Research Program to foster the development of cutting edge genomic and informatic approaches to health. The investigators in CPHR study a broad spectrum of disorders, with a range of heritability from common diseases ...
Introduction. Precision health is a nascent field that seeks to maximise population health and well-being while minimising premature disability and death through the continuous monitoring of key health data, generation of actionable health discoveries 1 and recommendation of personalised interventions. 1 2 It is derived from precision medicine, which similarly considers individual variation in ...
UF Health Precision Health Research Center - The Villages® is guided by a community advisory board composed of local residents and is founded on a community-based participatory research, or CBPR, approach that ensures the community has a strong voice in the work it carries out.
Precision health research at UCLA is presently engaged in partnerships focused on the following areas: Cardiovascular Disease. Depression Grand Challenge. Metabolism Theme. UCLA Clinical Neurogenomics Research Center. Center for Autism Research & Treatment. Clinical & Translational Science Institute. Cancer Immunology.
The Duke Clinical and Translational Science Institute (CTSI) is pleased to announce the launch of the Center for Precision Health (CPH), a collaboration that will harness the power of genomic, biomarker, and health data to transform patient care and population health.. The Duke University School of Medicine has a rich history of translational discovery science leveraging genetics, genomics ...
Precision Health reimagines medicine to focus on predicting, preventing, and curing disease precisely. Marrying two seemingly different approaches — high-tech and high-touch — this Stanford Medicine vision tailors health care to the unique biology and life circumstances of each individual, with an emphasis on catching disease before it strikes.
With precision health research, IRBs must be able to evaluate several risk-to-benefit profiles as well as a diverse set of tools, methods, and stakeholders. Clearly, resources are needed to support risk-to-benefit assessments and mitigation strategies, which will assist those planning safe and ethical research as well as those responsible for ...
Abstract. Introduction: Precision health is a nascent field of research that would benefit from clearer operationalisation and distinction from adjacent fields like precision medicine. This clarification is necessary to enable precision health science to tackle some of the most complex and significant health problems that are faced globally.
Princeton Precision Health is a cross-cutting initiative that uses AI and data science to transform human health at all levels: from molecular to social to environmental, with the goal of enacting smart policy and evidence-based clinical practice. We are an interdisciplinary team of researchers from a wide variety of fields, including computer ...
Objective: To determine progress and gaps in global precision health research, examining whether precision health studies integrate multiple types of information for health promotion or restoration. Design: Scoping review. Data sources: Searches in Medline (OVID), PsycINFO (OVID), Embase, Scopus, Web of Science and grey literature (Google Scholar) were carried out in June 2020.
The primary objective of this scoping review is to map the current state of human precision health research, specifically to: Identify how precision health is operationalised in scientific manuscripts and patents. Summarise precision health research study characteristics (eg, study settings, sample characteristics and research designs).
What are Clinical Trials? Precision health is a unified approach to match a full range of promotion, prevention, diagnostic, and treatment interventions to fundamental and actionable determinants of health; to not just address symptoms, but to directly target genetic, biological, environmental, and social and behavioral determinants of health.
The ConNECT Framework (Figure 1 in Alcaraz et al., 2017, Menon et al., 2018 ), is an actionable model that incorporates five broad, synergistic principles for use across the translational continuum as it relates to precision health. This framework grew out the work of the Health Equity Special Interest Group of the Society for Behavioral Medicine.
The University of California San Diego (UCSD) Microbiome and Metagenomics Center (MMC) as part of the Nutrition for Precision Health consortium will provide rapid, robust stool sample processing, high-quality metagenomic and metatranscriptomic data generation, and best-in-class bioinformatic analysis. The data will be used by the Nutrition for Precision Health Consortium to understand the ...
The UF Health - Precision Health Research Center in The Villages brings together a team of scientists, clinicians, learners, innovators in industry, and community partners. What unites us is a passion for improving health and wellness for older adults, and a desire to advance science that facilitates our ability to predict, prevent, and cure ...
The goal of the NIH Common Fund's Nutrition for Precision Health, powered by the All of Us Research Program, is to develop algorithms that predict individual responses to food and dietary patterns. Nutrition plays an integral role in human development and in the prevention and treatment of disease. However, there's no such thing as a perfect ...
The Medical University of South Carolina is putting precision health front and center, bringing together experts to talk about what it is, how it's being used and what the future may hold. MUSC recently held a Precision Health Research Symposium, led by Lori L. McMahon, Ph.D., vice president for Research and a professor of Neuroscience.
In Precision health care, participant and facilitator will be setting a goal and developing a detailed written action plan for achieving glycemic control in reducing HbA1C. ... The APC was funded by the Research Center for Healthcare Industry Innovation, National Taipei University of Nursing and Health Sciences, Taipei City 112, Taiwan ...
Project Description. The Precision Medicine for Alzheimer's Disease and Related Dementias Project is a joint project conducted in collaboration with the Center for Alzheimer's and Neurodegenerative Disease at Baylor College of Medicine. This project aims to integrate precision medicine (cognitive testing, genomic testing, and neuroimaging ...
Nutrition for Precision Health, powered by the All of Us Research Program, or NPH, is working with 14 sites across the United States - including Pennington Biomedical Research Center and LSU ...
Background: Developing innovative, efficient, and institutionally scalable biospecimen consent for remnant tissue that meets the National Institutes of Health consent guidelines for genomic and molecular analysis is essential for precision medicine efforts in cancer. Objective: This study aims to pilot-test an electronic video consent that individuals could complete largely on their own.
Reframing and broadening precision medicine beyond 'omics' (e.g., genomics, proteomics, metabolomics) has been referred to as "precision population health" or "precision public health." These goals of precision population health have been reflected in the US Precision Medicine Initiative, including the one-million-person research ...
Clinical Center Grand Rounds: Great Teachers Distinguished Clinical Research Scholar and Educator in ... Professor of Human Genetics Neurology and Psychiatry Senior Associate Dean and Associate Vice Chancellor of Precision Health Institute for Precision Health University of California Los Angeles For more information go to https ...
The Research Data Analyst (RDA) will be tasked with managing incoming data, implementing data quality controls, visualizing data and generating and implementing analysis models for neuroimaging and behavioral data and their integration. Data are acquired at multiple time points, including pre- and post-treatment.
Precision health is a term that refers to personalized healthcare based on a person's unique genetic, genomic, or omic composition within the context of lifestyle, social, economic, cultural and environmental influences to help individuals achieve well-being and optimal health [ 1, [13], [14], [15] ]. The successful implementation of ...
Precision environmental health can provide a targeted approach to prevent disease by focusing exposure mitigation, interventions, and treatments at the level of the individual, and to those most vulnerable. Such strategies can be incorporated into primary, secondary, and tertiary prevention 103 (Fig. 3 ). Fig. 3.