share this!
April 15, 2024
This article has been reviewed according to Science X's editorial process and policies . Editors have highlighted the following attributes while ensuring the content's credibility:
fact-checked
peer-reviewed publication
trusted source
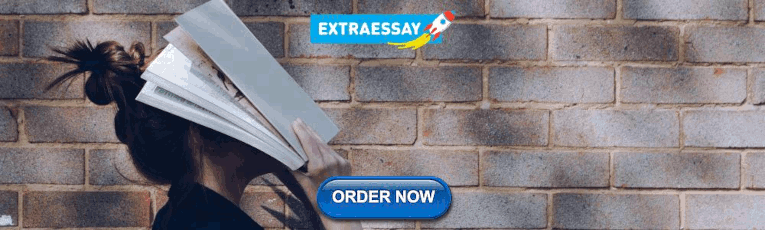
Switch to green wastewater infrastructure could reduce emissions and provide huge savings, new research finds
by Colorado State University
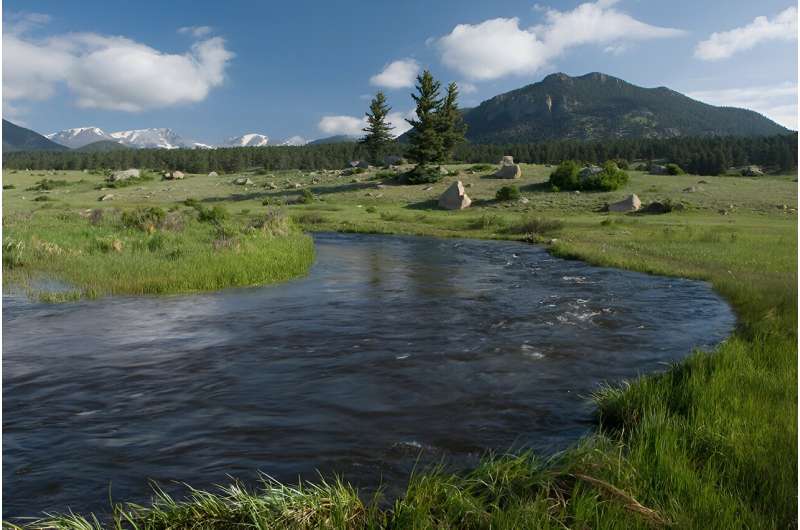
University researchers have shown that a transition to green wastewater-treatment approaches in the U.S. that leverages the potential of carbon-financing could save a staggering $15.6 billion and just under 30 million metric tons of CO 2 -equivalent emissions over 40 years.
The comprehensive findings from Colorado State University were highlighted in Nature Communications Earth & Environment in a first-of-its-kind study. The work from the Walter Scott, Jr. College of Engineering explores the potential economic tradeoffs of switching to green infrastructure and technology solutions that go beyond existing gray- water treatment practices.
Built off data collected at over 22,000 facilities, the report provides comprehensive baseline metrics and explores the relationship among emissions, costs and treatment capabilities for utility operators and decision-makers.
Braden Limb is the first author on the paper and a Ph.D. student in the Department of Systems Engineering. He also serves as a research associate in the Department of Mechanical Engineering. He said the findings are a key initial step to categorize and understand potential green solutions for wastewater.
"These findings draw a line in the sand that shows what the potential for adopting green approaches in this space is—both in terms of money saved and total emissions reduced," he said. "It is a starting point to understand what routes are available to us now and how financing strategies can elevate water treatment from a somewhat local issue into something that is addressed globally through market incentives."
The research was completed in partnership with the University of Colorado Boulder and Brigham Young University. Findings center around both point-source water treatment and non-point sources of water pollution.
Traditional point-source water treatment facilities such as sewage plants remove problem nutrients like nitrogen and phosphorus before releasing water back into circulation. This gray-infrastructure system—as it is known—is monitored by the Environmental Protection Agency.
However, regulation standards may tighten in the future, and facilities would need more power, and in turn more emissions, to reach newly allowable thresholds. Existing facilities already account for 2% of all energy use in the U.S. and 45 million metric tons of CO 2 emissions, said Limb.
Another significant source of freshwater contamination in the U.S. comes from non-point source activity such as fertilizer runoff from agriculture entering rivers. Other non-point sources of pollution can come from wildfires—aided by climate change —or urban development, for example.
Limb said that rather than building more gray-infrastructure treatment facilities to address those increasing sources, the paper explores green approaches financed through carbon markets that can tackle both types simultaneously.
"There could be a switch to nature-based solutions such as constructing wetlands or reforestation instead of building yet another treatment facility," he said. "Those options could sequester over 4.2 million carbon dioxide emissions per year over a 40-year time horizon and have other complementary benefits we should be aiming for, such as cheaper overall costs."
Carbon financing is a mechanism aimed at mitigating climate change by incentivizing activities that reduce emissions or sequester them from the atmosphere. Companies voluntarily buy "credits" on an open market that represent a reduction or removal of carbon from the atmosphere that can be accomplished in a variety of ways. That credit offsets the institution's emissions from operations as it aims to reach sustainability goals.
These trades incentivize development of sustainable activities and can also provide a source of fresh money to further develop or scale up new approaches.
While there are similar financing markets for water, the problem is initially more localized than it is for air quality and carbon. That dynamic has limited the value of water market trades in the past. The paper suggests that these existing markets could be improved, and that the carbon markets could also be leveraged to change some of the financial incentives farmers have around water treatment and impacts from their activity.
The researchers found that using the markets could generate $679 million annually in revenue, representing an opportunity to further motivate green infrastructure solutions within water quality trading programs to meet regulated standards.
Mechanical Engineering Professor Jason Quinn is a co-author on the study. He said the findings have some limitations, but that this was an important first step to model both the problem and opportunity available now. He said the results in the paper have supported new research at CSU with the National Science Foundation to further develop the needed carbon credit methodology with stakeholders.
"This is the first time we are considering air and water quality simultaneously—water is local and carbon is global," he said. "But by bringing these market mechanisms together we can capitalize on a window of opportunity to accelerate the improvement of America's rivers as we transition to a renewable energy and restored watershed future."
Journal information: Nature Communications Earth & Environment
Provided by Colorado State University
Explore further
Feedback to editors

Astrophysics research advances understanding of how gamma-ray bursts produce light
27 minutes ago

Simulated microgravity affects sleep and physiological rhythms, study finds
50 minutes ago

A million years without a megaslide: Study goes deep into the Gulf of Alaska to investigate why

AI weather forecasts can capture destructive path of major storms, new study shows

New 2D material manipulates light with remarkable precision and minimal loss

Investigating the porosity of sedimentary rock with neutrons

Kissing bugs, vector for Chagas disease, successfully gene edited for first time

Novel graphene oxide spray coating advances antiviral protection of face masks

New super-resolution microscopy approach visualizes internal cell structures and clusters via selective plane activation
2 hours ago

Steering toward quantum simulation at scale
Relevant physicsforums posts, unlocking the secrets of prof. verschure's rosetta stones.
11 hours ago
Tidal friction and global warming
Apr 20, 2024
Large eruption at Ruang volcano, Indonesia
Apr 19, 2024
Iceland warming up again - quakes swarming
Apr 18, 2024
M 4.8 - Whitehouse Station, New Jersey, US
Apr 6, 2024
Major Earthquakes - 7.4 (7.2) Mag and 6.4 Mag near Hualien, Taiwan
Apr 5, 2024
More from Earth Sciences
Related Stories

Why water must be at the heart of climate action
Mar 26, 2024

Study shows making cities greener doesn't just capture carbon—it reduces it
Sep 1, 2023

Tapping the potential of wastewater for a sustainable future
Oct 11, 2022

Modeling study: Linking carbon markets with an allowance exchange rate yields environmental, economic dividends
May 31, 2023

Blending hydrogen with natural gas could help fuel energy transition
Feb 8, 2023

Taking a global look at dry and alternative water cooling of power plants
Aug 9, 2023
Recommended for you

Mangrove blue carbon at higher risk of microplastic pollution
5 hours ago

Feedback loop that is melting ice shelves in West Antarctica revealed
4 hours ago

The Italian central Apennines are a source of CO₂, study finds

Weather prediction models can also forecast satellite displacements

Warming of Antarctic deep-sea waters contribute to sea level rise in North Atlantic, study finds
Let us know if there is a problem with our content.
Use this form if you have come across a typo, inaccuracy or would like to send an edit request for the content on this page. For general inquiries, please use our contact form . For general feedback, use the public comments section below (please adhere to guidelines ).
Please select the most appropriate category to facilitate processing of your request
Thank you for taking time to provide your feedback to the editors.
Your feedback is important to us. However, we do not guarantee individual replies due to the high volume of messages.
E-mail the story
Your email address is used only to let the recipient know who sent the email. Neither your address nor the recipient's address will be used for any other purpose. The information you enter will appear in your e-mail message and is not retained by Phys.org in any form.
Newsletter sign up
Get weekly and/or daily updates delivered to your inbox. You can unsubscribe at any time and we'll never share your details to third parties.
More information Privacy policy
Donate and enjoy an ad-free experience
We keep our content available to everyone. Consider supporting Science X's mission by getting a premium account.
E-mail newsletter

Switch to green wastewater infrastructure could reduce emissions and provide huge savings according to new research
University researchers have shown that a transition to green wastewater-treatment approaches in the U.S. that leverages the potential of carbon-financing could save a staggering $15.6 billion and just under 30 million tonnes of CO 2 -equivalent emissions over 40 years.
The comprehensive findings from Colorado State University were highlighted in Nature Communications Earth and Environment in a first-of-its-kind study. The work from the Walter Scott, Jr. College of Engineering explores the potential economic tradeoffs of switching to green infrastructure and technology solutions that go beyond existing grey-water treatment practices. Built off data collected at over 22,000 facilities, the report provides comprehensive baseline metrics and explores the relationship among emissions, costs and treatment capabilities for utility operators and decision makers.
Braden Limb is the first author on the paper and a Ph.D. student in the Department of Systems Engineering. He also serves as a research associate in the Department of Mechanical Engineering. He said the findings are a key initial step to categorize and understand potential green solutions for wastewater.
"These findings draw a line in the sand that shows what the potential for adopting green approaches in this space is -- both in terms of money saved and total emissions reduced," he said. "It is a starting point to understand what routes are available to us now and how financing strategies can elevate water treatment from a somewhat local issue into something that is addressed globally through market incentives."
The research was completed in partnership with the University of Colorado Boulder and Brigham Young University. Findings center around both point-source water treatment and non-point sources of water pollution.
Traditional point-source water treatment facilities such as sewage plants remove problem nutrients like nitrogen and phosphorus before releasing water back into circulation. This grey-infrastructure system -- as it is known -- is monitored by the Environmental Protection Agency. However, regulation standards may tighten in the future, and facilities would need more power, and in turn more emissions, to reach newly allowable thresholds. Existing facilities already account for 2% of all energy use in the U.S. and 45 million tonnes of CO 2 emissions, said Limb.
Another significant source of freshwater contamination in the U.S. comes from non-point source activity such as fertilizer runoff from agriculture entering rivers. Other non-point sources of pollution can come from wildfires -- aided by climate change -- or urban development, for example.
Limb said that rather than building more grey-infrastructure treatment facilities to address those increasing sources, the paper explores green approaches financed through carbon markets that can tackle both types simultaneously.
"There could be a switch to nature-based solutions such as constructing wetlands or reforestation instead of building yet another treatment facility," he said. "Those options could sequester over 4.2 million carbon dioxide emissions per year over a 40-year time horizon and have other complementary benefits we should be aiming for, such as cheaper overall costs."
Carbon financing is a mechanism aimed at mitigating climate change by incentivizing activities that reduce emissions or sequester them from the atmosphere. Companies voluntarily buy "credits" on an open market that represent a reduction or removal of carbon from the atmosphere that can be accomplished in a variety of ways. That credit offsets the institution's emissions from operations as it aims to reach sustainability goals.
These trades incentivize development of sustainable activities and can also provide a source of fresh money to further develop or scale up new approaches.
While there are similar financing markets for water, the problem is initially more localized than it is for air quality and carbon. That dynamic has limited the value of water market trades in the past. The paper suggests that these existing markets could be improved, and that the carbon markets could also be leveraged to change some of the financial incentives farmers have around water treatment and impacts from their activity.
The researchers found that using the markets could generate $679 million annually in revenue, representing an opportunity to further motivate green infrastructure solutions within water quality trading programs to meet regulated standards.
Mechanical Engineering Professor Jason Quinn is a co-author on the study. He said the findings have some limitations, but that this was an important first step to model both the problem and opportunity available now. He said the results in the paper have supported new research at CSU with the National Science Foundation to further develop the needed carbon credit methodology with stakeholders.
"This is the first time we are considering air and water quality simultaneously -- water is local and carbon is global," he said. "But by bringing these market mechanisms together we can capitalize on a window of opportunity to accelerate the improvement of America's rivers as we transition to a renewable energy and restored watershed future."
- Nature of Water
- Energy Policy
- Energy Technology
- Engineering
- Sustainability
- Environmental Issues
- Environmental Science
- Carbon dioxide sink
- Geologic temperature record
- Climate engineering
- Carbon dioxide
- Commercial fishing
- Automobile emissions control
Story Source:
Materials provided by Colorado State University . Original written by Josh Rhoten. Note: Content may be edited for style and length.
Journal Reference :
- Braden J. Limb, Jason C. Quinn, Alex Johnson, Robert B. Sowby, Evan Thomas. The potential of carbon markets to accelerate green infrastructure based water quality trading . Communications Earth & Environment , 2024; 5 (1) DOI: 10.1038/s43247-024-01359-x
Cite This Page :
Explore More
- Flare Erupting Around a Black Hole
- Two Species Interbreeding Created New Butterfly
- Warming Antarctic Deep-Sea and Sea Level Rise
- Octopus Inspires New Suction Mechanism for ...
- Cities Sinking: Urban Populations at Risk
- Puzzle Solved About Ancient Galaxy
- How 3D Printers Can Give Robots a Soft Touch
- Combo of Multiple Health Stressors Harming Bees
- Methane Emission On a Cold Brown Dwarf
- Remarkable Memories of Mountain Chickadees
Trending Topics
Strange & offbeat.
Thank you for visiting nature.com. You are using a browser version with limited support for CSS. To obtain the best experience, we recommend you use a more up to date browser (or turn off compatibility mode in Internet Explorer). In the meantime, to ensure continued support, we are displaying the site without styles and JavaScript.
- View all journals
- My Account Login
- Explore content
- About the journal
- Publish with us
- Sign up for alerts
- Review Article
- Open access
- Published: 12 April 2022
Recent developments in hazardous pollutants removal from wastewater and water reuse within a circular economy
- Menatalla Ahmed 1 ,
- Musthafa O. Mavukkandy ORCID: orcid.org/0000-0003-3540-5748 1 ,
- Adewale Giwa ORCID: orcid.org/0000-0002-3509-7477 1 ,
- Maria Elektorowicz 2 ,
- Evina Katsou 3 ,
- Olfa Khelifi 4 ,
- Vincenzo Naddeo ORCID: orcid.org/0000-0002-3395-3276 5 &
- Shadi W. Hasan ORCID: orcid.org/0000-0002-7269-094X 1
npj Clean Water volume 5 , Article number: 12 ( 2022 ) Cite this article
18k Accesses
107 Citations
3 Altmetric
Metrics details
- Biogeochemistry
- Pollution remediation
Recent advances in wastewater treatment processes have resulted in high removal efficiencies for various hazardous pollutants. Nevertheless, some technologies are more suitable for targeting specific contaminants than others. We comprehensively reviewed the recent advances in removing hazardous pollutants from industrial wastewater through membrane technologies, adsorption, Fenton-based processes, advanced oxidation processes (AOP), and hybrid systems such as electrically-enhanced membrane bioreactors (eMBRs), and integrated eMBR-adsorption system. Each technology’s key features are compared, and recent modifications to the conventional treatment approaches and limitations of advanced treatment systems are highlighted. The removal of emerging contaminants such as pharmaceuticals from wastewater is also discussed.
Similar content being viewed by others
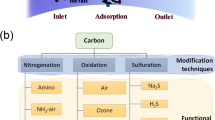
Removal of heavy metal ions from wastewater: a comprehensive and critical review
Naef A. A. Qasem, Ramy H. Mohammed & Dahiru U. Lawal
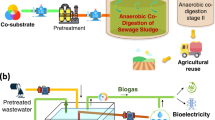
Pathways to a net-zero-carbon water sector through energy-extracting wastewater technologies
Aishwarya Rani, Seth W. Snyder, … Shu-Yuan Pan
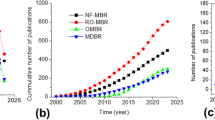
Strategies for mitigating challenges associated with trace organic compound removal by high-retention membrane bioreactors (HR-MBRs)
Oranso T. Mahlangu, Thabo I. Nkambule, … Faisal I. Hai
Introduction
Industries are significant water consumers. On a global scale, they consume ~22% of the total water produced, whereas, in high-income counties, it can reach up to 60%. It is estimated that by 2050, manufacturing industries alone could increase their water usage by 400% 1 . Aqueous discard from the use of water in various industrial steps such as cooling tower, heating by the boiler, purification, etc. may contain numerous suspended or dissolved contaminants, and these effluents are referred to as industrial wastewater 2 . Industries such as chemical and petrochemical, paper and pulp, food processing, tannery, and other manufacturing industries constitute the primary sources of industrial wastewater 3 . These wastewaters usually have high organic strength (1-200 g/L), non-neutral pH, different temperatures, salinity, turbidity, and high heavy metal content 4 . Wastewater from leather manufacturing, food processing and preservation, textile processing, and petroleum refining may have high salt concentration 5 . Wastewater composition varies depending on the chemicals used in the upstream processes and the nature of treatment it has undergone; thus classifying industrial wastewater into specific categories is challenging 2 .
Ideally, industrial wastewater should undergo proper treatment and subsequent disposal into the environment or reuse for landscaping and housekeeping 2 . However, the regulatory control of industrial wastewater is region-specific, with many countries are having little or no effective legal frameworks supported by regulatory institutions 6 . According to UNESCO, 70% of industrial effluents in developing countries are dumped untreated 7 . However, as more countries are tightening their regulatory frameworks, industries are facing challenges in meeting the stringent water discharge and reuse requirements 8 .
Conventional treatments for removing heavy metals from wastewater include chemical precipitation, flotation, and ion exchange. However, these processes have several drawbacks, such as low removal efficiency, high energy consumption, and generation of toxic sludge, that limit their widespread application 9 . Recently, various alternative treatment methods have been investigated to improve the quality of the treated effluent. They include adsorption using low-cost materials, membrane separation, electro-technologies, and photocatalytic processes. Adsorption and membrane separation are widely used for treating wastewater contaminated with high heavy metals concentrations. Photocatalytic methods are effective in removing organic matter and recovering metals and hence are expected to be more prevalent in the future 10 . To the best of authors’ knowledge, no comprehensive and critical review in the literature discusses the latest innovations in the removal of hazardous pollutants from industrial wastewater. Therefore, this work is aimed at reviewing the recent advances in the removal of hazardous pollutants from industrial wastewaters. Membrane-based technologies, adsorption, Fenton-based process, advanced oxidation and photocatalytic processes, and hybrid systems are critically reviewed and discussed.
Membrane technologies
Microfiltration (mf), ultrafiltration (uf), nanofiltration (nf), and reverse osmosis (ro).
Membrane processes are increasingly being implemented for treating industrial and municipal wastewater because of their simplicity, modularity, and better energy efficiency 11 . Based on the pore size of the employed membrane, these processes can be broadly classified into microfiltration (MF), ultrafiltration (UF) 8 , 12 , nanofiltration (NF) 8 , 13 , and reverse osmosis (RO) 13 . MF, UF, and NF membranes are used for the removal of contaminants with a size range of 100–1000, 5–100, and 1–5 nm, respectively. There are several recent studies reporting treatment of industrial wastewater using membrane processes. For example, RO and NF were effective in removing Cu 2+ and Cd 2+ from synthetic wastewater 14 . RO achieved 98% and 99% removals for Cu 2+ and Cd 2+ , respectively, whereas NF achieved ~90% for both Cu 2+ and Cd 2+ . Another study reported 99.5% removal of Cu 2+ and Ni 2+ using RO 15 . Galambos et al. compared the chemical oxygen demand (COD) removal efficiency of RO and NF in treating the food industry wastewater; two samples of wastewater were used in this study with an initial COD of 9500 and 1160 ppm 16 . It was found that the permeate from RO has a COD of less than 125 mgO 2 L −1 and hence can be discharged into natural waters. The study did not investigate the energy requirements associated with the treatment processes.
Polymer-supported ultrafiltration (PSU) has been investigated for treating synthetic wastewater containing heavy metals. Barakat and Schmidt added carboxyl methyl cellulose (CMC) as a water-soluble polymer for complexing the cationic forms of heavy metals such as Cu 2+ , Ni 2+ , and Cr 3+ prior to filtration 17 . The results revealed that a high pH (≥7) enhanced the formation of CMC-heavy metal complexes, precipitation and size exclusive removal of metal hydroxides. In many cases, adjusting the solution pH is found to be an important step in heavy metal rejection as high pH can induce the precipitation of heavy metal hydroxides, which can then be filtered out through UF membranes. Juang and Shiau [20] investigated the removal of Cu 2+ and Zn 2+ from synthetic wastewater using chitosan-enhanced membrane filtration 18 . The presence of chitosan in the feed solution enhanced the metal removal by 6–10 times, which is attributed to the presence of the amino group in chitosan that served as a site for metal binding. Diethylaminoethyl cellulose 19 and polyethyleneimine (PEI) 20 are other examples of water-soluble metal-binding polymers used for heavy metal removal. Heavy metal removal can also be enhanced by the addition of minerals into the membrane reactor 21 . For example, the addition of 10 g L −1 vermiculite at pH=8 resulted in >99% removal of Pb 2+ , Ni 2+ , Cu 2+ and Zn 2+ 21 . Consequently, the integrated process of sorption-UF with suitable pH adjustment (i.e. >7) can be applied as a pre-treatment stage to remove heavy metals from industrial wastewater, such as metal plating 21 , 22 .
Adsorbents can be used to increase the heavy metal removal efficiency in membrane processes. For instance, Malamis et al. (2010) used adsorbents such as bentonite, vermiculite, and zeolite (clinoptilolite) for removing Cu 2+ from activated sludge permeate containing 317 mg L –1 Cu 2+ 23 . Adsorption using 10 and 20 g L –1 bentonite or vermiculite (pH: 5.5) followed by the ultrafiltration achieved a removal efficiency from 93.8–96.8 to 99.4% of Cu 2+ , respectively. The ultrafiltration alone was able to remove only 59.4–78.3% of Cu 2+ , indicating the superior performance of adsorbent-enhanced UF systems. The addition of zeolite and bentonite reduced the fouling, whereas vermiculite did not show any antifouling properties. As a follow-up study, Katsou et al. investigated the removal of Ni 2+ via a combined adsorption and UF system 24 . In a batch UF unit with an initial Ni 2+ concentration of 320 mg L –1 at a pH of 6, 65.3, and 80% removal efficiencies were obtained with 15 g L –1 each of bentonite and vermiculite respectively 24 . Membrane processes have also been used to treat saline industrial wastewaters containing high organic content. For instance, suspended solids (SS) and colloidal COD were reduced from seafood-processing wastewater by initial concentration via a UF system, followed by recycling of proteins to be used in fish meal production 25 . Turano et al. combined MF and centrifugation to remove up to 80% of the SS and 90% of the COD from olive oil mill effluent 26 .
It is worth noting that although RO is the most efficient in removing heavy metals from wastewater, it is not widely applied for this purpose when compared to other membrane processes such as MF, UF or NF, as it requires high energy for operation 11 . Also, the aerobic condition can promote the formation of metal oxides that can prematurely foul the RO membranes, necessitating frequent membrane replacements. However, if the wastewater also requires demineralization in addition to the removal of heavy metals, then RO might be a better technology to opt for.
Membrane bioreactors (MBRs)
Membrane bioreactors (MBRs) combine biological processes and membrane filtration to achieve better treated effluent quality by exploiting the dual benefits of membrane separation and activated sludge processes (ASPs). In the case of high strength industrial wastewater, operating parameters such as HRT, SRT, and MLSS should be optimized, and suitable pretreatment or neutralization should be provided to preserve the microbial community and prolong the membrane life 2 .
Metal sorption on activated sludge takes place as follows:
Cell wall and cell membrane contain various cations like Na + , Κ + , Mg 2+, and Ca 2+, and these ions will be exchanged with other dissolved metals in the MBR mixed liquor.
Interaction between metals and the sorption sites on cell surface induces complexation and/or microprecipitation.
The removal of metals in MBRs depends on a variety of parameters 27 , 28 such as: (i) operating parameters like dissolved oxygen (DO) levels, mixed liquor SS (MLSS), and sludge retention time (SRT), (ii) physicochemical parameters such as metal type, species, and initial concentration, the type and concentration of ligands, the presence and concentration of competing cations, (iii) biochemical parameters such as the concentration and content of extracellular polymeric substances (EPS), ligands produced through bacterial activity, products from cell lysis, and bacterial species that favor metal uptake.
Aerobic MBR utilizes biological treatment with aerobic microorganisms that prosper in presence of oxygen. MBRs have been used for removing various pharmaceutical compounds with varying efficiencies. Although longer SRTs were usually associated with higher removal efficiencies, contradictory results were obtained for diclofenac and ethinylestradiol. It was concluded that antiepileptic compounds like carbamazepine were resistant to removal by MBR, whereas other compounds such as Bisphenol-A, the natural estrogens, ibuprofen, and bezafibrate were removed sufficiently. At a temperature of 10 °C, 80% removal of bisphenol-A was observed at SRT higher than 10 days. Also, the natural estrogens 17β-estradiol (E2), estrone (E1), and estriol (E3) were nearly removed completely. Similarly, the removal efficiency of more than 95% was observed for ibuprofen and bezafibrate 29 . GE Corp. has developed an effective MBR system named Zee-Weed MBR to treat wastewaters from the pharmaceuticals industry 30 . However, it should be noted that MBR processes, like any other biological process, is not specifically designed for removing pharmaceutical compounds. The level of biodegradation will thus depend on how recalcitrant these compounds are and whether they can be sorbed to activated sludge or remain in the liquid phase.
MBRs have been used for removing the oil, grease, and other organics from petrochemical-contaminated wastewater containing various compounds with typical 10,000–20,000 mg L –1 SS, 2000–4000 mg L –1 COD, and up to 1000 mg L –1 total Kjeldahl nitrogen (TKN) 30 . In another study, MBR reported 99.9% removal of fuel and lubricant oil at hydraulic retention time (HRT) of 13.3 h. It was observed that the quality of the treated effluent met industrial process water standards 31 . A study conducted on a full-scale MBR plant showed a 90% removal of COD and complete removal of grease, oil, and phenolics 32 , whereas, industrial site of Porto-Marghera has a huge petrochemical MBR plant, whose permeate is being discharged into Lagoon of Venice 33 .
Sometimes, industrial wastewater might contain compounds that are toxic to microorganisms, which necessitates pre-treatment before biological treatment 3 . Katsou et al. investigated a submerged MBR for treating heavy metals from a synthetic wastewater effluent 21 . The municipal wastewater was added with 3.9–14.7 mg L –1 Pb 2+ , 3.4–9.1 mg L –1 Cu 2+ , 3.2–12.1 mg L –1 Zn 2+ , and 4.3–14.7 mg L –1 Ni 2+ and a hollow fiber membrane with a nominal pore size of 0.04 µm was employed. The MBR was operated at an HRT of 10.3 h and SRT of 15 days. A UF pre-treatment ensured the complete removal of total suspended solids (TSS). COD removal for the municipal wastewater was 95–97%, but it decreased to 83–91% upon the addition of heavy metals into the municipal wastewater. Comparing mixed liquor volatile suspended solids (MLVSS) before and after heavy metal addition showed that there was a 13% reduction in biomass due to the inhibition. Likewise, complete nitrification was observed in the case of municipal wastewater, but the addition of metals reduced it to 22–54% 21 .
When compared to traditional ASPs, MBR equipped with a UF membrane resulted in a 40–50% increase in the heavy metal removal efficiency 34 . Heavy metal removal mainly depends on the SRT, pH, and MLSS. High SRTs and MLSS, usually result in better heavy metal removal efficiency 35 . Metal ions are sorbed to the sludge particles attached to the UF membrane, which has resulted in the removal of Cu 2+ (59.4–78.3%) and Ni 2+ (23–50%) from industrial wastewater 23 . A submerged MBR with a hollow fiber membrane has also been found to significantly remove Cu 2+ and Cr 3+ from industrial wastewater 36 . Table 1 illustrates the literature data on MBR performance in industrial wastewater treatment for the removal of organics and nutrients from various industrial effluents.
There are several strategies for reducing the membrane fouling in MBRs. For instance, Deowan et al. coated a polyethersulfone (PES) UF membrane with an antifouling material and tested using synthetic textile dye wastewater 13 . The PBM MBR module showed around 10% higher dye removal when compared to commercial membranes and better fouling-resistance due to the antimicrobial properties of polymerizable cationic surfactant acryloyloxyundecyltriethylammonium bromide (AUTEAB).
Anaerobic membrane bioreactors (AnMBRs) are used to treat industrial wastewaters characterized by high organic matter. Anaerobic processes offer great sustainability benefits as they produce little sludge due to low biomass yield and consume less energy because of the absence of aeration. Moreover, anaerobic processes generate biogas that can be used as an alternative energy source 3 . Given the high organic loading of industrial wastewater, a comparably small amount of greenhouse gases is emitted to the atmosphere 37 . AnMBR system allows operation at high MLSS up to 15 g L –1 and can be run at long SRT of more than 20 days, and thus refractory and recalcitrant organics can be removed with high efficiency 38 . AnMBR can also retain specific microbial communities that can treat particular contaminants in wastewater 39 .
AnMBR has been employed for treating different types of wastewaters—from paper and pulp, food processing, winery, textile, pharmaceutical, oil, and petrochemical industries. For instance, the food processing industry produces wastewater that is non-toxic and readily biodegradable with high organic loading rate (OLR, 1000–85,000 mg COD L –1 ). AnMBR achieved a COD removal of >97% with OLRs higher than 12 kg COD m –3 d –1 4 . It was reported that 15 full-scale AnMBR plants have been operating around the world for food industry treatment 40 .
Bioaugmentation involves supplementing the microbial community with particular microorganisms to improve biodegradation of recalcitrant molecules. The added microbes should be compatible and competitive with the existing microbial communities in the system to avoid any damaging effects. This method is more environment-friendly and affordable when compared to other extra physico-chemical processes. There are several studies on improving the degradation performance via bioaugmentation. For example, Saravanane and Sundararaman investigated the treatment of pharmaceutical wastewater with a high concentration of Cephalosporin derivative using an AnMBR with a submerged flat sheet membrane 41 . They found an enhanced removal efficiency upon bioaugmentation. In another study, Qu et al. bio-augmented an MBR with Sphingomonas xenophaga QYY strain for treating wastewater containing anthraquinone dyes intermediates to achieve an enhanced color and COD removals 42 . Thus, bioaugmentation or post-treatment may be needed with MBRs/AnMBRs to ensure the enhanced degradation of pollutants from industrial wastewaters.
Quorum sensing (QS) refers to the bacterial communication using chemical signals like N-acyl homoserine lactones (AHL), and subsequent regulation of gene expression in bacterial communities as a response to the population density 43 . Several studies showed a high correlation between AHL signals and biofilm growth 44 , 45 , 46 , 47 . When reaches a threshold level, QS activates the transcription of specific genes and induce the secretion of exocellular enzymes, extracellular polymeric substances (EPSs), soluble microbial products (SMP), antibiotics, virulence, and bioluminescence. Quorum quenching (QQ) refers to the interference with QS via inhibiting or degrading signals, and interfering or blocking signal receptors for mitigating biofouling in MBRs 48 , 49 . Thus the use of antimicrobial compounds can be minimized, and the risk of developing anti-microbial resistance can be avoided 48 . For instance, Kim et al. reduced the biofouling by exploiting the double-benefits of improved friction and QQ using porous alginate beads trapped with QQ bacteria 50 . The increased friction between loose beads and the static membranes helped to loosen the biofilm on the membranes, whereas QQ bacteria helped reduce the formation of EPS. Another study reported an improved antifouling by encapsulating QQ bacteria on a polymer membrane layer 51 .
In general, the use of MBRs is useful for treating industrial wastewater. However, further research is needed to optimize the process and overcome the limitations. For instance, membrane fouling due to the deposition and growth of microbes on membrane surface or pores causes a gradual increase in trans-membrane pressure (TMP) and a decline in water flux. This necessitates frequent cleaning and membrane replacements, leading to increased operation and maintenance costs 52 , 53 . Furthermore, the exact nature of the interactions between membrane surface and foulants cannot be easily identified. Also, most of the published research investigated the efficiency of the bench and pilot-scale systems. Limitations of pH, temperature, pressure, and some corrosive chemicals constrain the use of MBR, especially in large-scale operations 2 . Leakage of contaminants through the membranes, due to the gradual degradation of membranes poses another challenge. Although QQ is promising in ensuring the long-term operation of MBR with minimal fouling, further work should be conducted to evaluate MBR performance with QQ bacteria in treating industrial wastewater in the pilot and full-scale. The electricity consumption of MBRs is usually higher than most of treatment processes. Due to the high membrane aeration rates required to manage fouling and clogging, MBR energy consumption was three times higher even than that of conventional activated sludge (CAS) systems combined with advanced treatment techniques 54 . Recently, more efforts have been focusing on reducing the energy consumption associated with MBR. The electrical consumption was reported to be in the range 1.43 kWh m –3 to 4.23 kWh m –3 55 , Energy consumption at 985 Japanese municipal WWTPs were analyzed and it was reported that CAS systems consumed between 0.3 kWh m –3 and 1.9 kWh m –3 56 . A balanced comparison of MBR and CAS (or other) systems is only possible, however, when similar effluent quality is produced.
Adsorption and ion exchange
Adsorption refers to the transfer of gas or liquid molecules into a solid sorbent surface and holding them via physical and/or chemical intermolecular interactions 10 . Ion exchange refers to the transfer of ions between an electrolyte and a complex or between two electrolytes solutions. In many textbooks, ion exchange is grouped under adsorption, and henceforth in this article, the term adsorption also covers the ion exchange as well. Adsorbents can be natural (e.g., charcoal, clays, minerals such as bentonite and vermiculite, zeolites, and ores) or synthetic (produced from agricultural products and wastes, industrial or urban wastes, sewage sludge, metal oxides, and polymeric adsorbents) 57 , 58 , 59 . The adsorption has been effective in removing dyes, and organic pollutants and metals from various industrial wastewater effluents 60 . It is pivotal to determine the thermodynamic parameters such as standard free energy change (ΔG°), to predict the feasibility of the process. For instance, if ΔG°< 0, the process is both spontaneous and feasible and vice versa 60 . Recently, adsorption via activated carbon (AC), low-cost industrial products, and biosorption has been investigated and are discussed below.
Adsorption on activated carbon (AC)
Activated carbon (AC) for industrial wastewater treatment is usually applied in two forms – powdered (PAC) and granular (GAC). Adsorption unit can either be used before biological treatment for removing toxic compounds or be placed after the physio-chemical treatment steps for ensuring the complete removal of micropollutants. AC can be used to remove organics such as pesticides, phenols, pharmaceuticals, organic halogens, non-biodegradable compounds, dyes, and inorganics such as Hg 2+ , Pb 2+ , Cd 2+ , Cu 2+ , and Ni 2+ , 61 , 62 . AC is also an efficient media for microbial growth, and biologically activated carbon (BAC) has been developed for the inactivation of biological pollutants within a short period. Attaching biomass to AC can remove contaminants by both adsorption and biodegradation 62 . However, AC has some disadvantages, such as expensive thermal/chemical regeneration methods and the loss of a significant fraction of adsorbent during regeneration 63 . Moreover, the adsorption mechanism on AC depends on various factors such as dispersive, electrostatic and chemical interactions; intrinsic properties of the solute and adsorbent and hence the interaction between the pollutants and AC is difficult to predict 64 . Although AC has a high adsorption capacity, it can maintain it only until the adsorption sites become exhausted with pollutants. Other absorbents such as polymeric absorbents are also used when recycling of valuable chemicals is desired 58 , 62 .
The adsorption of phenols by commercial PAC showed that the removal efficiency increases up to an optimum dosage, beyond which the improvement was negligible 65 . It can be observed that the GAC is usually used for removing natural organic matter, synthetic organic compounds, and heavy metals 61 . Zhang et al. used GAC for removing algal odorants like dimethyl trisulfide and ß-cyclocitral 66 . Adsorption isotherms were investigated for the process and found that Freundlich isotherm was fitting the best. The ΔG° for adsorption of ß-cyclocitral and dimethyl trisulfide were –4.24 and –3.61 kJ mol −1 at 298 K, respectively 66 .
Cyanide, for instance, was found to be better removed by biologically activated GAC compared to virgin GAC. Also, biodegradable anthraquinone dyes were removed more efficiently because of the elevated substrate concentration at the granular surface found in BAC systems 62 . Table 2 shows a summary of the adsorptive removal of heavy metals from industrial wastewater by AC.
Not only AC is an effective method to remove heavy metals, it has also been used for the removal of other pollutants. AC was used to remove crystal violet dye and had a maximum adsorption capacity of 84.11 mg/g and a removal efficiency of 85–90% 67 .
Adsorption on natural materials
Adsorption on natural materials such as zeolites has been gaining more interest. Adsorption of metal ions on the zeolite active sites produce inner and outer-sphere complexes. The interposition of at least one water molecule between the bound ion and the functional group of the adsorbent results in the formation of outer-sphere complexes. In contrast, inner-sphere complexes will be formed when there is no interposition of water molecule(s). A coordinate bond will be formed between the heavy metals and the surface functional groups 68 , 69 . For example, Clinoptilolite, a type of zeolite, has shown high selectivity to Pb 2+ , Cd 2+ , Zn 2+ , and Cu 2+ 10 . Also, it was found that polymeric materials can be used to increase the efficiency of natural clay to remove heavy metals by modifying the natural clay into a composite named clay-polymer composite 70 . For example, clay-poly(methoxyethyl)acrylamide (PMEA) composite has been synthesized to study its capacity to adsorb Pb 2+ ions. Bentonite, another type of clay has also exhibited high removal (>99%) of heavy metals removals like Cu 2+ , Co 2+ , Ni 2+ , Zn 2+ , and Pb 2+ ions. The adsorption affinities of the metal species were as in the below order: Co 2+ >Cu 2+ >Ni 2+ = Zn 2+ >Pb 2+ 71 . Another study also reported the complete (100%) removal of Pb 2+ from aqueous solutions using 20 g L –1 of bentonite 72 .
Natural phosphates (NP) constitute another category of raw adsorbents 73 . NPs are abundant, cheap, and non-hazardous to the environment, and can be used for heavy metals removal. It was reported that NP has a monolayer adsorption capacity of 26 mg g –1 for Cd 2+ at a pH of 5.0 74 . Another study reported a room temperature adsorption capacity of 200 mg Pb 2 + /g of rock phosphate (low-grade) when the initial aqueous Pb 2+ concentration was 50 mg L –1 . Adsorption of Pb 2+ on PO 4 3- followed a pseudo-first-order rate and Langmuir isotherm 75 . Nano-hydroxyapatite (nHA) is a less-soluble, abundant and stable phosphate that has a high sorption capacity for heavy metals. It has been used in the adsorptive removal of Pb 2+ and Cd 2+ from polluted soils 73 . Applying nHA can reduce water-soluble Pb 2+ by 72% and Cd 2+ by 90%, bioaccessible Pb 2+ by 12.5–27.5% and Cd 2+ by 17.7–34.6% 73 . It was also reported that nHA had a maximum adsorption capacity of 2500 mg of Sn 2+ g –1 . The process was endothermic and followed Langmuir isotherm 76 .
NPs have also been used to remove emerging and persistent organic contaminants. The adsorption of a reactive dye, Reactive Yellow 84, by hydroxyapatite (HAP) has been found to follow Langmuir isotherm with a monolayer adsorption capacity of 50.3 mg g –1 at a pH of 5.0. Adsorption of this dye is endothermic (enthalpy of adsorption is 2.2 kJ mol –1 ) and has a low temperature dependency 77 . Nanocrystalline HAP has been used to remove nitrobenzene 78 . The authors achieved a 52.4% removal with an adsorbent dosage of 5 g L –1 , whereas the removal was increased up to 95% when the dosage was 25 g L –1 78 . However, the adsorption capacity of nitrobenzene on nanocrystalline HAP is relatively low compared to adsorption on AC (100–300 mg g –1 ). This was attributed to the smaller surface area of nanocrystalline HAP (42.3 m 2 g −1 ) when compared to AC (1000 m 2 g –1 ) 78 . The effectiveness of mesoporous silica-alumina (MSA) on the removal of hydrocarbons from industrial wastewater has been investigated 79 . The dehydroxylated silicon content is the main factor in the adsorption process. Water is adsorbed on the MSA surface by interacting with silanol groups; aromatic hydrocarbons such as benzene and toluene interact with electron-poor acid sites. However, the presence of areas rich in hydrophilic Al enclosed in an active silica matrix can create spots that can deter the efficient removal of hydrophobic hydrocarbons.
Adsorption on industrial by-products
Industrial by-products such as carbonaceous wastes, agricultural by-products, mineral-derived sources, etc. can be used as low-cost adsorbents for industrial wastewater treatment 80 . For instance, steelmaking slag comprised of hydroxides of iron and calcium is used as a low-cost adsorbent 81 , 82 , 83 , 84 . Fe(OH) 3 and Fe(OH) 2 present in the slag provide adsorption sites for ions such as As 3+ , and Cr 3+ whereas Ca(OH) 2 increases the solution pH and enhances the heavy metal precipitation 83 . The removal of Cd 2+ by steel industry slag has also been investigated. The optimum adsorbent concentration was found to be 10 g L –1 for treating 100 mg L –1 of Cd 2+ solution at pH 4.0, and the removal rate could reach up to 99.1%. The removal is attributed to the chemisorption, including chemical precipitation and coordination reactions 84 .
Other examples of low-cost industrial by-product adsorbents are fly ashes 85 , 86 , 87 , 88 , 89 , waste Fe, hydrous TiO 2, and other waste products which can be fine-tuned chemically to enhance pollutant removal 10 . Fly ash can be chemically modified with NaOH and CH 3 (CH 2 )15N(Br)(CH 3 ) 3 for Cd 2+ and Cu 2+ removal 89 . The mechanisms of adsorption on industrial by-products differ from one material to another. Adsorption on hydrothermally modified fly ash, for instance, is based on electrostatic attraction. Other mechanisms depend on ion exchange and the structure of surfaces 11 . In general, using low-cost adsorbents (such as the ones obtained as by-products or from natural resources) has introduced an alternative for industrial wastewater treatment systems. The comparative advantages of low-cost adsorbents are their relatively low prices and abundance since they are natural materials or by-products from agricultural and industrial activities. Some of these adsorbents exhibit a high selectivity for specific contaminants. Required wastewater-pretreatment and fine-tuning the adsorbent materials are some limitations. Moreover, in some cases, the heavy metals in the slag may leach out and cause secondary contamination, and hence using those industrial by-products as adsorbents has to be done carefully 83 .
Biosorption
Biosorption involves concentrating pollutants, particularly heavy metals, by binding them with inactive microbial biomass mainly via adsorption and chelations 10 , 90 , 91 , 92 . Although this is attributed to the metabolism-independent binding of heavy metals to the cell walls, the actual mechanisms are yet to be understood 10 , 90 . Several mechanisms, such as chemisorption (ion exchange and chelation), complexation, and physical adsorption, are proposed 90 . For example, a study on the removal mechanism of Cu 2+ , Ni 2+ , Zn 2+ , Pb 2+ and Cr 3+ by Penicillium chrysogenum attributed ion exchange as the principal removal mechanism 93 . The uptake of Pb 2+ by R. glutinis is also attributed to the transfer of ions and biomass released phosphate induced precipitation. Also, the uptake of Pb 2+ by Aspergillus parasiticus cell wall takes place by ion exchange and complexation processes 94 . Cu 2+ ions are also bioadsobed by Fucus serratus by ion exchange. When Ca 2+ ions are released from the surface of the biomass, a bond between Cu 2+ and functional groups forms 94 , 95 . Also, when Ni 2+ is bioadsorbed by Lathyrus sativus, dative bonds will be formed between Ni 2+ and nitrogen in the ammonia functional group of the biomass species 96 . Chelation and ion-exchange are proposed to be the two main mechanisms of chemisorption of Cu 2+ by walnut and hazelnut shells 96 . Many other biomass sources such as peanut and hazelnut shells 10 , 97 , green alga 98 , orange peel 99 , Rhizopus sp . biomass 100 , jackfruit 10 , maize cob or husk 10 , and their chemical modification or thermal conversion to AC have been used during biosorption 10 .
Weak Van der Waals forces between the heavy metal ions and adsorbent surfaces constitute the primary removal mechanism in physisorption. An example of physical adsorption is Cd 2+ adsorption by olive cake, which achieved a 66% removal at 28 °C and pH of 6 101 . Another study on the removal of Zn 2+ , Pb 2+ , Fe 2+ , and Cu 2+ using dried red seaweed Kappaphycus sp . also indicated the removal of metals vis physisorption 102 . Malamis et al. (2011) applied activated sludge coupled with UF to enhance the removal of Ni 2+ , Cu 2+ , Pb 2+ and Ni 2+ 103 . The highest removal of Pb 2+ was found at pH=4 and Zn 2+ , Ni 2+ , and Cu 2+ at pH = 6. Table 3 illustrates data obtained from the literature of several biosorption cases.
Biosorption has been reported to remove pollutants other than heavy metals. For instance, modified lemon leaf was used to remove cationic dye, and was found to have an adsorption maximum capacity of 36.10 mg g –1 and yielded 70% removal efficiency 104 . Another example was the utilization of modified biogas residue to remove nitrate and phosphate. The maximum adsorption capacity was reported to be 64.12 mg g –1 and 34.40 mg g –1 for nitrate and phosphate, respectively 105 .
Several modified biopolymers have been developed for heavy metal removal applications, which include natural rubber, Lyocell fiber, and chitosan-based adsorbents 11 . Biopolymers are widely used in industries due to their availability, environmental safety, and ability to reduce heavy metals to parts per billion 10 . Such materials do have certain drawbacks, however. For example, the biopolymer chitson in its natural form has low specific selectivity for heavy metals and low adsorption capacity for complex polluted wastewater 106 . Nevertheless, good sorption capacity for transition metals has been demonstrated for chitosan with a high content of hydroxyl and amine groups, but little to no sorption capacity for alkaine or alkaine earth elements 106 .
Heavy metal removal by biopolymers can be enhanced by modifying its chemical and physical properties 10 , 11 , 107 . For instance, chemical and physical methods can be used on chitosan to improve its removal efficiency. Chitsoan can be modified physically by preparing the polymer in different forms. Other forms of chitosan include water-soluble and water-insoluble chitosan, such as flakes nanoparticles and beads 11 , 106 . Modified chitosan beads were suggested for the diffusion of various metal ions and specifically Cu 2+ ions through spherical chitosan-tripolyphosphate (TPP) chelating resins, which are prepared using an in-liquid ionotropic crosslinking method 108 . Additionally, the study of Liu et al. [149] suggests that non-porous glass beads can be used to create hybrid materials by immobilizing chitosan on their surface 109 . Chemically modified chitosan is also beneficial for wide heavy metal sorption applications. The most highlighted modifications of chitosan are the grafting chitosan and the cross-linked chitosan 11 . Polysaccharide-based-materials have also been developed as modified biopolymer adsorbent, which is derived from chitin, chitosan, and starch for the removal of heavy metals from wastewater 10 .
Future research should aim at developing new and low-cost adsorption materials with high treatment efficiencies as for most adsorption processes, the cost of the adsorbent constitutes up to 70% of the total cost 110 . Also, most of the studies focus on determining the maximum adsorption capacity of an adsorbent, which assumes a fixed-bed adsorption system. This might not be the case in industry, and more studies should aim at investigating the maximum capacity in real-life processes. The performance of fixed-bed adsorbers is different when compared to agitated batch adsorbers for instance. Another very important aspect that is often not focused on is desorption and regeneration. Since the world is more concerned with sustainability and environment nowadays, more studies should focus on adsorbents regeneration and reuse.
Advanced oxidation processes (AOPs)
Advanced oxidation processes (AOPs) are chemical treatments involving the generation of hydroxyl radicals (OH • ) that can efficiently oxidize recalcitrant pollutants 111 . OH • are characterized by their high standard oxidation potential (up to 2.80 V) and their high reaction rate in comparison to common oxidants like chlorine, oxygen, ozone, H 2 O 2 , or potassium permanganate. Hence, high rate constants can be achieved during the reaction of OH • with both inorganic and organic solutes 112 . AOPs, in general, employ the efficacies of different oxidants to degrade hazardous pollutants by converting them from their reduced forms to their final harmless oxidized forms. This conversion mineralizes and degrades the contaminants to harmless substances for overcoming the environmental impacts due to the disposal of the primary pollutants to the aquatic ecosystem. Although these systems use different oxidants, they all tend to share the same radical production 113 . These processes have a high potential to purify water from pollutants that are hard to be removed by biological methods 114 . AOPs include two main stages: The formation of strong OH • /oxidants and the interaction of these radicals with the targeted pollutants to convert them to carbon and water in the best-case scenario. When two OH • interact, H 2 O 2 is formed, as shown in below equation:
The comparative advantages of AOPs are (i) high disinfection strength: Several AOPs are used because of their great disinfection properties 115 , and (ii) standalone destruction of organic contaminants. If methyl tert-butyl ether, for example, was removed by stripping, additional processes such as catalytic oxidation would be needed for effective treatment. However, AOPs destroy the organic contamination directly without the need for other chemical processes 116 .
However, the generation of undesirable oxidation by-products affect AOPs. If these oxidation by-products have slow reaction rates, there would be a delay in mineralization, leading to unwanted accumulation. Also, inorganic substances are formed during some AOPs, such as bromide conversion to bromate during ozonation. These inorganic compounds interfere with AOPs and inhibit the oxidation reactions. These compounds scavenge the OH • , which are meant to remove and destroy the concerned contaminants 116 . Therefore, there is need to address the issue of radical scavengers and the likelihood of producing unwanted intermediate derivatives from the oxidized forms of pollutants. pH influences the acid-base equilibrium involving OH • formation and the radical’s concentration.
Two critical parameters should be taken into considerations while designing and constructing an efficient AOP system. Firstly, the dosage of chemicals as it will increase the cost and may give the possibility of by-product formation. Secondly, reactor configuration and contact time, which is often determined when implementing a pilot study rather than a lab-scale, should also be considered 116 . The quality of industrial wastewater and other operating conditions also affect the efficiency of degradation of concerned pollutants. It is known that most of the organic substances would react instantly with the introduced radicals 116 . Turbidity also acts as an influencer to the system performance because the more turbid the industrial wastewater, the lower is the penetration of UV source to the water. Additionally, Fe 2+ and Cu 2+ or other heavy metals in wastewater may also cause the formation of Fe or Cu organic complexes thus results in fouling for the system 116 . The recently employed AOPs are the Fenton-based processes, electrokinetic treatment, and degradation with metal oxides.
Fenton-based processes
Fenton processes are catalytic processes that generate hydroxyl radicals (OH • ) from H 2 O 2 upon the addition of Fe 2+ 112 . OH • are produced from the oxidation of Fe 2+ to Fe 3+ as in below equation 111 :
However, the Fenton process produces Fe sludge waste because Fe 3+ precipitates as FeO(OH) 112 . Thus, the typical Fenton process can be improved by coupling it with electricity to have an electro-Fenton process, or with UV or solar light to have a photo-Fenton process, to reduce Fe 3+ back to Fe 2+ and reduce the amount of waste sludge 111 . The UV produces more OH • by photolysis and activates photo-decarboxylation of ferric carboxylates 117 . Table 4 summarizes the application of Fenton treatment for the removal of various types of contaminants. Recent advances in the Fenton-based processes such as Fenton, photo-Fenton, and electro-Fenton are elaborated in the next subsections.
FeSO 4 is an adequate salt for ferrous generation, and it showed a TOC removal efficiency of 94% after 2 h 118 . FeSO 4 can be generated by adding Fe catalyst to H 2 SO 4 . Fenton process via FeSO 4 was shown to be very useful and efficient in terms of oxidation and degradation of TOC 118 . Organophosphorus pesticides removal from wastewater has been evaluated using the Fenton process under various reaction conditions at room pressure and temperature. The optimum condition was determined by several parameters such as pH, stirring time, and dosage of FeSO 4 and H 2 O 2 . COD removal efficiencies for an actual triazophs wastewater treatment plant and a bench-scale experiments were 85.4% and 96.3%, respectively. Complete oxidation of phosphorus and nitrogen content was observed through which eutrophication is minimized 119 . The efficiency of treating wastewater obtained from a synthetic fiber factory that uses acrylic polymer has also been investigated. The more H 2 O 2 was added, the more the effectiveness of degrading the unwanted pollutants. However, an increase in the COD content of the treated effluent was observed at a concentration above 500 mg L –1 of H 2 O 2. Also, pH levels seemed to impact the removal efficiency of color and COD. The pH was carefully selected for the removal of color since a decrease in pH (<6) led to the destabilization and aggregation of particles 120 .
Apart from FeSO 4 , Fe 3 O 4 has also been examined as a reagent that can be combined with H 2 O 2 in a Fenton process for the degradation of phenols 121 , 122 . Many characteristics were taken into consideration, but the one that played a significant role was Fe 2+ and Fe 3+ ratio, which was determined by the chemical analysis. Fenton reaction started slow but accelerated eventually. Phenol degradation was achieved efficiently in Fe 3 O 4 that has a higher structural content of Fe 2+ . The use of magnetite showed positive features such as safe levels of Fe 2+ content in wastewater/water effluent, a magnetic behavior that separates the reagent easily from the treated feed, and easy absorption of UVA radiation, which enhanced the reaction.
Recently, Fenton pilot-scale experiment has been implemented to degrade synthesized C 20 H 18 NO 4 + 123 . The main parameters were optimized using response surface methodology technique. Acidic industrial wastewater sample was collected from a chemical factory, and subsequently, pH was adjusted prior to the oxidation process. Values given by the statistical method were highly efficient and relatively close to the obtained experimental results. The berberine removal efficiency was found to be 35.6% at a pH of 3.5.
The photo-Fenton process has improved the TOC removal efficiency when compared with the Fenton process due to the enhancement of TOC degradation rate by photons 124 . Photo-Fenton process at neutral pH with UV254 is a promising technique in which it degrades all pollutants in a limited time. For this process, Fe is not necessarily needed if the treated water contains at least 1.5 mg L –1 of Fe 2+ or Fe 3+ 125 . This technique is appropriate for large-scale systems and can be used to replace the available conventional solutions such as ozonation. However, a relatively low amount of pollutant degradation would be observed when photo-Fenton is implemented using regular sunlight.
Photo-Fenton technology has also proved to be efficient for the removal of pesticides from the water with high salinities 126 . Additionally, photo-Fenton technology has also been reported as efficient for the removal of pesticides from the water with high salinities. Oxidation rates were much faster from the beginning when the water was more saline. For water with lower salinity, oxidation rates started to increase only after about 60 min of treatment. The effect of conductivity on the mineralization of organic content of saline water was mainly caused by interference by chloride. The photo-Fenton process has also been used for dye removal. Low levels of H 2 O 2 in the process may result in the formation of more toxic products. In some cases, however, the pollutant may be degraded without the creation of any toxic by-products depending on the solubility of the contaminant in water. Therefore, the solubility of pollutant in water has a significant role in the photo-Fenton process. Photo-Fenton is also reported as useful for the full degradation of 4 colorants in wastewater streams from the food and cosmetics industries 127 .
Electro-Fenton is an emerging process where H 2 O 2 is electro-generated at the cathode made with carbon materials. This method is safe because H 2 O 2 is produced in-situ, and the risk of handling H 2 O 2 is reduced. It is also a faster process in degrading many pollutants because of the constant generation of Fe catalyst at the cathode 128 . A simple illustration of the electro-Fenton mechanism is shown in Fig. 1a . Removal of Alizarin Red has been successfully implemented recently using the electro-Fenton technique with a graphite-felt electrode where the cathode was fed with air to reproduce H 2 O 2 . Ferrous ions played the central role in the removal since the oxidant alone has a limited capability. Through this method, Alizarin Red was oxidized to a colorless acid and then to carbon dioxide 129 . 100% color removal has also been achieved through the electro-Fenton process 130 . By using the GAC electrode as the cathode and air in the electro-Fenton process, stability, and efficiency in removing methyl orange has been achieved 130 . It was, however, observed that the electro-generation of H 2 O 2 was limited at longer times due to the oxidation to oxygen at the anode, but still, the limited value is much more significant when compared to methods without air bubbling approach. It was also mentioned that oxygen did not contribute to the reduction process since the rate of increase of H 2 O 2 generation followed a linear relationship with the imposed current for the first hour 130 . The use of AC cathode was also studied previously in Taiwan by 131 , where the pollutant adsorption on the cathode was measured, and the highest COD removal reached around 75%.
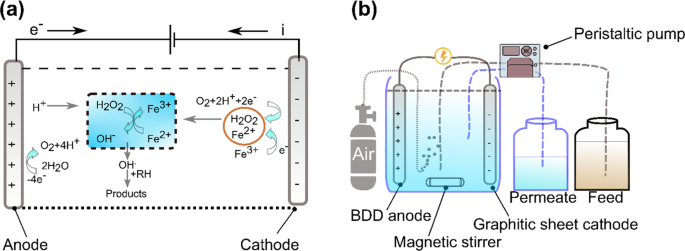
( a ) Illustration of reaction mechanism in electro-Fenton process (inspired from 308 ), and ( b ) The schematic of a continuous electro-Fenton process (inspired from 296 ).
Electro-Fenton process has also been applied for the degradation of surfactants by using graphite-felt cathode 119 . The critical parameter was to apply different electrolytes in the presence of Fe catalyst. The decay kinetics of the surfactant was unaffected by its initial dosage, highly dependent on the applied current, Fe catalyst concentration, and pH; and followed a pseudo-first-order reaction. Another recent application of the graphite cathode electro-Fenton process is the removal of antibiotic residue from industrial wastewater. A highly bioactive antibiotic (tetracycline) was mineralized to carbon dioxide through this method 123 . Meanwhile, the recent development and use of nano-enhanced carbon electrodes in the electro-Fenton process can ensure enhanced stability and pollutant degradation rate. Graphite cathodes have been compared with carbon nanotube (CNT) sponge in an electro-Fenton process 114 . The CNT sponge was used as the cathode of the electro-Fenton process under high electrical power. The CNT sponge showed enhanced stability and a good degradation rate that was estimated to be ten times higher than the one obtained by graphite cathode. Recently, the efficiency of carbon-felt electrode has also been compared with those of alternative non-carbon materials as anode 132 . The materials, such as boron-doped diamond (BDD), Ti with Pt coating, and Ti with TiO 2 coating, were used as electrodes to remove carbamazepine from wastewater. The BDD anode showed the fastest oxidation and mineralization efficiency; and exhibited much better catalytic ability than the others 125 . Also, the BDD anode showed a better performance in mineralizing Atrazine into C 3 H 3 N 3 O 3 as the ultimate end product. This study compared its results with the previous experiment conducted on C 8 H 14 ClN 5 removal with classical Pt anode. Although the classical Pt anode was faster in decaying Atrazine, it was slower in mineralizing it. BDD anode has the potential to produce high amounts of OH • . These formed OH • are physically adsorbed to the anode surface, which enhanced pollutant uptake. The study concluded a full removal of the insecticide at an initial pH of 2. The efficiency of the BDD anode agreed with previous results 132 . A continuous electro-Fenton process with graphite sheet cathode and BDD anode is illustrated in Fig. 1b .
Furthermore, Tylosin antibiotic was degraded after 15 min of applying electro-Fenton, and it followed pseudo-first-order 124 . The degradation of Tylosin antibiotic showed a possibility to combine biological treatment with electro-Fenton because the biodegradability showed an improvement where the BOD 5 /COD ratio increased from zero to 0.6 after 6 h of electrolysis. After 6 h, the application of electro-Fenton alone gave more energy efficiency than the combination. It was suggested that the mineralization should be optimized by balancing the energy used in case both processes are operating together. In addition, electro-Fenton has been integrated with absorption in order to enhance the kinetics of color removal. The use of Fe-loaded AC as an absorbent, together with electro-Fenton has shown promising results in winery wastewater treatment 133 . Fe-loaded AC implementation alone exhibited 23% color removal after 24 h. However, almost total color removal was achieved at the same time when electro-Fenton was integrated with Fe-loaded AC absorption. The adsorbent was used as a catalyst to optimize the process kinetics so that higher degradation rates can be reached. The adsorbent also showed a better physical capability than Mn and Fe alginate beads. Several conventional electro-Fenton process studies emphasized that the process can ensure a total removal or high removal efficiency at a pH of 3 128 . However, a recent optimization study using Box-Behnken design and response surface methodology showed higher efficiency when the pH was increased up to 5 128 . It was illustrated that if the pH was below 5, then H 2 O 2 cannot be decomposed to the oxidant radical by Fe 2+ . At pH below 5, hydrogen would gain one electron, and this would lead to a reduction in the rate of reaction between the Fe 2+ and H 2 O 2 . Consequently, a reduction in the degradation of pollutants would be observed. The results obtained in several recent studies on Fenton-based processes in terms of the removal of highly hazardous pollutants are shown in Table 5 . An overview illustration of various Fenton processes is presented in Fig. 2
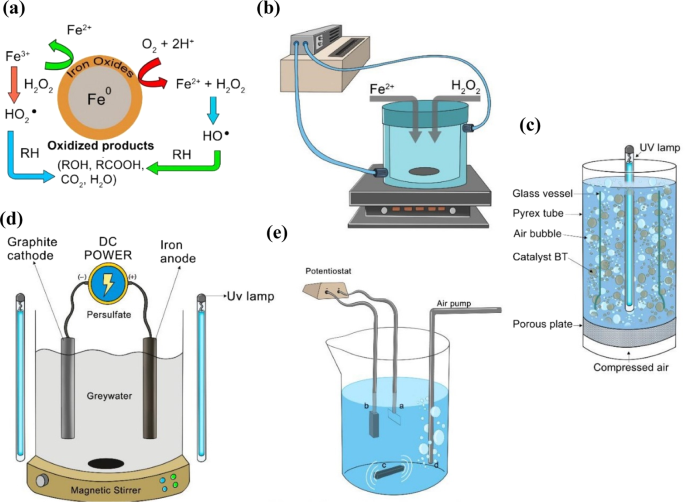
( a ) Illustration of Fenton mechanism, ( b ) Fenton set-up, ( c ) Photo-Fenton set-up, ( d ) Photo-Electro-Fenton device, and ( e ) Electro-Fenton set-up.
Electrokinetic treatment
Electrokinetic treatments such as electrocoagulation (EC), electrochemical oxidation (EO), and electroflotation (EF), electroosmosis, and electrophoresis help in the degradation of various pollutants via electrochemical mechanisms. They are specifically beneficial for the treatment of industrial wastewater, such as textile wastewater. Some of the contaminants that have been removed successfully by electrokinetic treatments include decolorizing reactive dye solutions and phenolic compounds, and heavy metals 134 .
Electrocoagulation (EC) is an electrokinetic process that utilizes the electrical field to form aggregated particles. EC generates coagulates via the decomposition of electrodes. Ion generation occurs at the anode, while hydrogen gas is produced at the cathode, allowing electofloculation to take place since the hydrogen released helps in keeping the flocculated particles float 135 . Colloidal solids and particles, metals, and soluble inorganic pollutants are some of the materials that EC can remove from aqueous media by neutralizing their charges using the charged polymeric metal hydroxide species. Neutralizing suspended solids charges causes the contaminates to agglomerate and then separate from the aqueous phase.
EC has been widely used for treating industrial wastewaters with metallic content 136 . The efficiency of EC depends on the type of wastewater. For example, COD removal by EC in textile wastewater has been found to be from 40 to 70%, 96% for soluble oils, and 80% for paper waste 137 . One of the key factors to consider when using EC for the treatment of industrial wastewater is the electrode material. The effect of three different types of electrode materials, namely Al, stainless steel, and Fe on EC’s efficiency for the removal SO 4 2- from a refinery’s wastewater has been reported 138 . The electrodes used in the study have the same area, contact time, and CD. It was observed that Al electrodes have the highest efficiency, in which, the sulfide reduction was 2.5 times higher than stainless steel and Fe electrodes. This is due to the reaction between Al(OH) 3 and SO 4 2- in the cell, causing sulfate salts to participate at the bottom of the cell. SO 4 2- can also get trapped in porous precipitate and increase extraction efficiency. Additionally, the solubility of Al 2 (SO4) 3 is less than that of ferrous sulfates (400 kg m −3 vs. 600 kg m −3 ). Therefore, the precipitation of Al 2 (SO 4 ) 3 can occur at a faster rate. The electrode with the lowest efficiency among the three materials is stainless steel, due to its resistance to corrosion 138 . However, a high concentration of calcium or magnesium ions can increase stainless steel effectivity drastically 135 . This demonstrates that the type of pollutant should be taken into consideration when choosing an electrode. Wang and Chou [209] reported that using Al as cathode and anode, because of its low hydrogen overvoltage, gives the highest turbidity removal and COD in the treatment of tanning wastewater. At the same time, Fe or steel can be more effective in treating the same wastewater, depending on the targeted pollutant 139 . Another study showed that Al electrodes yield better effluent quality for leather tanning industrial wastewater treatment in comparison with other electrodes 140 . Therefore, in general, Al pairs of electrodes might be more suitable for the removal of organic matter. In contrast, Fe electrodes might provide a higher removal of inorganic pollutants, such as Cr, Ca, and Zn 141 . For instance, Fe electrode is not effective for COD removal, since soluble and miscible organic compounds such as glucose, isopropyl alcohol, phenol, sucrose do not react with Fe 2+ and Fe 3+ . Only a small amount of these organic compounds can be incidentally removed by sorption on the floc. COD value might increase when compounds (usually acids) react with Fe 2+ to form soluble products, which remain in the solution. Additionally, COD can be partially removed when sodium oxalate, other similar salts, and certain acids are present in the wastewater. In addition to protons, EC generates Fe ions which hydrolyze to form Fe(OH) 2 and Fe(OH) 3 . OH − ions are not attracted to sodium ions since Fe ions are more acidic, which causes a very low percentage of CH 3 COO − and similar ions are removed. Acids, such as C 6 H 8 O 7 , C 7 H 6 O 3 , C 4 H 6 O 6 and C 2 H 2 O 4 acids, react with Fe 2+ and Fe 3+ to form soluble and insoluble, respectively. COD can only be effectively removed if the present organic compounds can react with Fe ions to form insoluble compounds, as exhibited by hydroxoaluminum ions 142 . Therefore, when Al and Fe electrodes are compared, Al electrodes show higher COD removal efficiencies, especially at lower pH values. This is because Al has only one oxidation state, namely Al 3+ , which allows a complete reaction of the organic compound to form an insoluble compound 142 .
EC can also be used to treat oil-in-water emulsions 143 , 144 , 145 , 146 , 147 . The coagulants produced in-situ cause the break-up of the emulsion by reducing the surface charge of the droplets. This causes the coalescence of oil droplets, which is then followed by separation either by settling or by dissolved air-flotation. The primary removal mechanism is bridging flocculation or the attachment of absorbing macromolecules to several droplets simultaneously 148 , 149 . In bridging flocculation, electrically charged sites on the surface of the droplet are attracted to species with the opposite charge. Additionally, the adsorption properties of growing metal hydroxides can be utilized for the elimination of oil (Fe and Al hydroxides). It was found that the adsorptive layers of anions and cations of growing hydroxides, in addition to its nucleus, produce positively charged particles that have high adsorption of oil droplets 148 , 149 . Also, destabilization by non-absorbing polymers can happen by depletion flocculation 148 . It has been found that the instability of oil emulsions and the removal of COD can only be attained for values of pH in the range of 5–9.
The aqueous behavior of Al includes the production of polymeric hydroxoaluminum ions, monomeric hydroxoaluminum ions, and Al(OH) 3 precipitates. Monomeric cationic hydroxoaluminum are the predominant species at low range of pH. When the pH is close to neutrality, aluminum hydroxides precipitates are the predominant species. The dissolution of the precipitates into monomeric anionic hydroxoaluminum occurs with an increase in pH 150 . The inability of the monomeric Al ionic species to destabilize oil emulsions can also be attributed to their steric constraints; monomeric Al ionic species have a smaller size in comparison to the size of the droplet; thus, enabling to act over oil droplets. On the other hand, the size of hydroxide precipitates, and polymeric ions can help more than one droplet in the attraction to the coagulant species 151 . When the electrical charge exceeds a certain threshold, de-emulsification occurs, which slightly decreases the removal efficiency. This is because the coagulant at an excess concentration reduces the efficiency, since it increases the concentration of Al(OH) 3 particles, and thus decreases the chance of attracting more than one droplet on the same particle of coagulant.
EC has been used to treat industrial wastewater rich in heavy metals such as Cr 6+ . Hexavalent Cr removal is achieved by reducing Cr 6+ to Cr 3+ , which then later precipitates in the form of neutral hydroxide. It was found that a higher CD corresponds to faster removal of Cr. Additionally, unlike Fe electrodes, COD removal was not affected by the presence of Cr 6+ by using Al electrodes 152 . 5 min was sufficient for the removal of 99% of Cr 6+ , but 10 min is required with using Al electrodes 153 . After 60 min of EC, flecks of aggregates were observed (Fig. 3 ). Cr 6+ ions can react with Fe 2+ to produce Cr 3+ and Fe 3+ 154 . By using Fe electrodes, the residual Cr concentration is dependent on the residual Fe concentration, because the removal of Cr ions is carried out by co-precipitation of Cr ions and Fe ions. Hence, complete precipitation of Fe ions is preferred 154 .
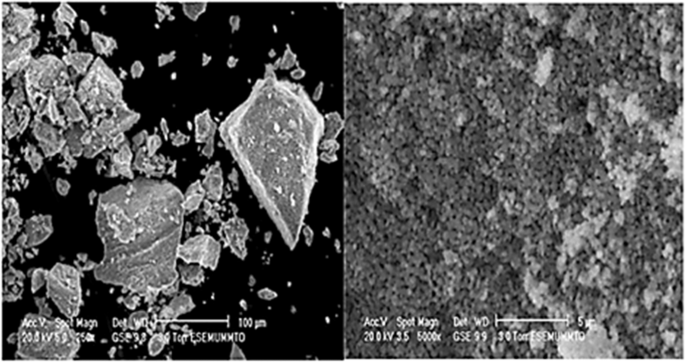
Flecks showed by scanning electron microscope (SEM) after 60 min of EC 153 .
EC has also been used recently to treat industrial wastewater containing Mn, Cu, and Zn at concentrations of 5 mg L –1 , 5 mg L –1 , and 10 mg L –1 , respectively, and at a pH of 6 155 . Cu and Zn were removed entirely, and more than 95% removal of Mn was achieved. Decreasing the initial pH resulted in a decrease in removal efficiency. Also, the higher applied current was associated with higher efficiency. The use of different initial concentrations did not affect the removal of heavy metals. Another study reported a 96% arsenic (As) reduction by EC when Fe electrodes were used 156 . Cu, Cr, Pb, and Zn removal from billet industry wastewater was also studied. Around 99% of these heavy metals were removed at pH 5, CD of 98 A m −2 , and 30 min treatment time 157 .
EC has also been utilized to extract fluoride (F − ) from treated industrial wastewater, particularly that generated from steel industry 158 . F − is additionally present in wastewater generated from semiconductor, electroplating, glass, and ceramic industries 159 , 160 . By using EC, F − concentration can be reduced to 0.5 mg L –1 from a concentration of 4.0–6.0 mg L −1 using optimum HRT of 5 min 158 . Although an increase in HRT is associated with increased removal efficiency, this increase in removal efficiency is negligible after 5 min. Another study reported that increasing the number of Al plates in a reactor from one to three, increased the F − removal from around 90% to 93% at a constant potential of 30 V 153 . However, increasing the number of plates to about 6 had a negative impact on the F − removal efficiency. This can be explained by Ohm’s law; the current and resistance are inversely proportional at a fixed voltage. It can also be observed that increasing the number of plates resulted in producing more coagulants, resulting in increased resistance, and reduced current. The CD determines the coagulant dosage and size of the bubble production, which affects the growth of flocs.
EC process has also been tested for the removal of organics from the textile industry wastewater. A study reported COD reduction from 1316 mg L –1 to 42.9 mg L –1 by using RuO 2 /IrO 2 /TaO 2 and titanium electrodes 161 . In another study, COD and turbidity removal, the effect of electrode material, cell voltage, and other parameters were investigated in textile wastewater treatment using Al and Fe electrodes 152 . EC removes organic matters by two mechanisms. The first mechanism involves the removal of organic matters through indirect oxidation by utilizing chloride. The second mechanism is adsorptive/entrapment of organic matter, particularly colloids, and SS on metal hydroxide flocs 162 , 163 . Apart from the removal of organics, several studies have been conducted to investigate the extraction of color from textile wastewater. The extraction efficiency of color was found to be 95%-99% by EC using Fe and Al electrodes 164 . The decolorization kinetics followed the first-order law. The highest color extraction efficiency was attained at neutral and slightly acidic pH values 164 . Another study tested EC’s color removal efficiency for both synthetic and real wastewaters. It was found that the removal of color from synthetic wastewater was higher than that of real wastewater. This was due to the higher organic content in real wastewater, in addition to the complexity 165 . Table 6 summarizes the recent advances in industrial wastewater treatment by EC.
The specific energy consumption is directly proportional to the current and time 166 . It was predicted that US $1.86 is required for treating 1 m 3 of dye by EC process 167 . The operating cost increases with the increase in operating time for iron and aluminum electrode, while the operating cost is double for aluminum electrode EC as compared to iron electrode EC for the same operating time 168 . The operating cost of EC process for the treatment of industrial wastewater is minimal as compared with other process like Fenton and chemical coagulation. Furthermore, the cost of adsorption process is less compared to that of EC, where the generation of huge quantity of sludge is the major problem 169 .
Electrochemical oxidation (EO) of pollutants in industrial wastewater can be achieved via two main mechanisms. The first is direct anodic oxidation, where oxygen or OH • in the oxide lattice MOx+1 is generated 170 . The other mechanism is by indirect electrooxidation process in which the oxidation process is carried out via a generated mediator, such as chlorine, hypochlorite, ozone, and H 2 O 2 171 . Generally, the EO technique can remove a wide variety of pollutants, such as nitrogen species, microorganisms, and refractory organic matter, which are often found in industrial wastewater. Additionally, it is effective in treating non-biodegradable, toxic organic pollutants, nitrite, and nitrate, and some micro contaminants such as pharmaceuticals 135 . Advanced technologies that are based on chemical oxidation are usually used to treat biologically recalcitrant effluents 172 . Electrochemical reactions are additionally utilized for disinfection purposes by generating oxidizing species. High disinfection efficiency would be obtained from waters that contain chloride ions because the generation of oxidizing species would be achieved 173 .
EO is affected by cell design, pH, electrolyte composition, CD, temperature, and electrode materials. Electrodes should be stable and should display low activity toward oxygen evolution reaction and high activity towards organic oxidation 174 .
The most common electrochemical oxidant is chlorine, which is formed by the oxidation of chloride at the anode. The electrochemical oxidation of ammonia has also been reported, specifically that present in saline industrial wastewater 175 . An electrochemical cell consisting of circular BDD on a stainless-steel cathode and silicon anode was used. A high level of chloride ions in wastewater increased the ammonia elimination, and the TOC removal was reached up to 90% 175 . It was also found that the highest efficiency was associated with the lowest CD 175 . If the chloride content in wastewater is not high enough, salt must be added to increase the treatment efficiency 135 . The removal of dyes, solvents, and surfactants has also been achieved by EO 111 . BDD was used as an anode and stainless steel (AISI 304) as a cathode. A complete COD removal was achieved with very high current efficiency, depending on CD and the type of anions in the wastewater. The treatment of dyes is more efficient with using chlorine, while phosphates are better suited for the extraction of aliphatic compounds.
EO has also been utilized for the disinfection and treatment of latex wastewater. The initial concentration of COD and microorganism was 3820 mg L –1 and 180 CFU mL –1 , respectively. COD was reduced to a level of 78 mg L –1 , while the microorganism was completely removed. This was achieved through the hydrochlorites acid produced from electrolytic reactor that utilizes graphite as an anode and stainless steel as cathode. Equations ( 3 – 5 ) illustrates the electrochemical generation of chlorine/hydrochlorite in a solution that contains chloride ions 176 :
Nowadays, due to their high stability and efficiency, conductive-diamond anodes have been gaining more attention in the treatment of industrial wastewater containing organic pollutants 177 . This can be justified by the fact that the anodic activity depends on the value of the overpotential of oxygen evolution. High oxygen evolution overpotential is essential to avoid undesired side reactions that reduce the current efficiency of oxidizing organics 135 . IrO 2 , graphite, and Pt exhibit low values of overpotential of oxygen evolution in EO when compared to conductive-diamond anodes. This necessitates the application of a very low CD to remove pollutants effectively or the use of this anode when there is a high concentration of chlorides or metallic mediators. BDD film on titanium substrate also gives a high value of oxygen evolution overpotential. When this anode is used, oxidation can take place with a low amount of oxygen evolved when high values of current densities are present.
EO has been additionally used to remove tetrahydrofuran (THF) from rubber manufacturing wastewater 178 . THF is a cyclic ether used as a solvent and raw material for synthesizing polymers in the industry. The wastewater has an initial THF concentration of 688 ± 140 mg L –1 . THF was treated by EO by using four anodic materials, namely, BDD, RuO 2, PbO 2, and Pt. The CD applied was 300–1200 A m –2 . The experiment resulted in a fast THF removal when BDD anode was used using sodium sulfate as an electrolyte to prevent the formation of organochloride secondary products. Also, COD removal was higher than 98% and TOC elimination higher than 95%. However, the mineralization of organic pollutants was not achieved by using RuO 2 as an anode and sodium chloride as an electrolyte. The reason why sodium chloride was chosen as an electrolyte was that DSA, such as RuO 2 generates chlorine when NaCl is the electrolyte. The reduced efficiency of Ti/RuO 2 in NaCl electrolyte showed that the free chlorine generated is not an active oxidant for THF removal and mineralization 179 .
EO has also been used to remove COD from vegetable tannery wastewater by using a graphite anode 180 . The initial COD value was 9600 mg L −1 , and the final value obtained was 59 mg L −1 at a CD of 34 mA cm –2 and 120 min of electrolysis. In the case of chrome tanning wastewater, Cr 3+ was oxidized to Cr 6+ with a conversion of 96 ± 3% at a pH of 2.5–5.5. Cr 6+ was then converted to Cr 2 (SO 4 ) 3 for reuse in tanning operation 180 . EO treatment of tannery wastewater has also been carried out by using Ti/Pt and Ti/Pt/Ir anodes 181 . It was found that COD removal was not enough to meet the discharge regulations. Hence, the EO process via these electrodes cannot be used alone in the treatment of tannery wastewater 181 . Moreover, EO has been utilized to extract organic pollutants from textile and finishing wastewater 182 . The wastewater produced from the textile industry is challenging since it has a high pH, high temperature, intense color, high COD, and low degradability. Usually, dyeing wastewater can be treated by biological oxidation and adsorption. The effect of EO on finishing wastewater and textile dye was investigated using a stainless-steel cathode and titanium or platinum anode. The organic pollutants present in the wastewater were oxidized to water and carbon dioxide when passing through the cell. This is because of the high oxidizing ability of the chemicals generated in the cell, which include hydroxyl, chlorine, oxygen, and other oxidants. In total, 2 mL of 36% hydrochloric acid was added, and electrolysis was allowed to take place for 18 min at a current of 0.89 A cm −2 . The COD/BOD ratio decreased from 2.16 to 1.52, indicating that the biodegradability of wastewater was improved. Additionally, COD removal was found to be 86%, BOD removal was 71%, and ADMI color units were reduced completely 182 .
One comparative study that investigated energy consumption of EC and photocatalytic process for textile dye wastewater treatment has concluded that EC was more economically feasible as the energy consumption of EC process was less than 0.01 kWh m −3 per unit COD removed when compared to >100 kWh m −3 for the photocatalytic process 183 . Photocatalytic ozonation is considered to be one of the least energy-demanding amongst AOPs technologies. It was reported that the specific energy consumption of the photocatalytic ozonation system in the decomposition of oxalic acid and dichloroacetic acid was 0.007 kWh mM –1 and 0.024 kWh mM –1 , respectively. These values were less than those of catalytic ozonation (0.017 kWh/mM and 0.050 kWh mM –1 ) and photocatalytic oxidation (0.063 kWh mM –1 and 0.350 kWh mM –1 ) 184 .
EF technique is an electrokinetic phenomenon that has been used for the treatment of various types of industrial wastewater treatment 185 , 186 , 187 , 188 . It involves the flotation by electrically generated bubbles to separate two liquid phases or solid from liquid phases. Colloidal or finely dispersed particles in wastewater are removed by the small bubbles of O 2 and H 2 lifting contaminants to clarify the solution. The best recoveries could be obtained in the particle size range of 20–50 µm. The advantages of EF include simplicity, efficiency, environmental compatibility, safety, selectivity, reduction in sludge generation, minimization of added chemicals, and relatively little space requirement due to its shorter residence time 189 . The efficiency of EF in treating wastewater rich in heavy metals was studied by preparing a solution of NiSO 4 .6H 2 O, CuSO 4 .5H 2 O, PbSO 4, ZnSO 4 .7H 2 O, CdSO 4 .8H 2 O, and FeSO 4 .7H 2 O at an initial concentration of 100 mg L –1 for each salt, initial pH of 8, and conductivity of 2.7 mS cm −1 in the presence of sulfate 189 . EF showed an average heavy metal removal efficiency of 93%, and the kinetics was found to be fast (around 15 min) except for Ni. Another study investigated the feasibility of EC/EF process for heavy metals removal such as Pb, Ba, and Zn 190 . Up to 97% removal was achieved with stainless steel mesh electrodes. EC/EF has also been used to remove F − and CaF 2 nanoparticles from semiconductor industry wastewater 191 . Suspended matter and F − were eliminated by the combined methods. Additionally, the high turbidity removal efficiency was achieved by EF technique. Stainless steel was the cathode, and DSA titanium coated with RuO 2 (Ti/RuO 2 ) was the anode of the EF cell. The turbidity and F − removals were 97% and 73%, respectively.
EF is the most effective method for the separation of low-density SS and oil 185 , 187 , 188 . Up to 99.71% oil removal from an initial concentration of 1050 mg L –1 emulsified oil has been achieved by using an EF cell with DSA anode composed of Ti/Ru 0.34 Ti 0.66 O 2 and a stainless-steel cathode 187 . EF has also been used to treat wastewater from the dairy industry 192 , palm oil effluent using Pb dioxide-coated titanium anode 193 , mining wastewater 194 , and others. Moreover, EF can be a part of a hybrid process. One example of a hybrid EF process is composed of three stages: (i) adsorption by a bonding agent; (ii) wastewater filtration to separate the loaded bonding agent by two variants, i.e. crossflow MF for low-contaminated wastewater or a hybrid process combining flotation and submerged MF for highly contaminated wastewater; and (iii) bonding agent regeneration 195 .
Photodegradation by nano-scale TiO 2
Nano-TiO 2 can be utilized to photocatalytically degrade the residual organic contaminants in treated effluents. Nano-TiO 2 is useful for the degradation of endocrine disruptors, organic contaminants, micropollutants, and in water filtration membranes. Nano-TiO 2 structure and performance would depend on the preparation method. However, certain limitations such as inefficiency under visible light illumination, post-recovery, incomplete removal of toxic byproducts (in some cases), and low mechanical strength still need to be addressed for enhanced performance. TiO 2 post-treatment of secondary treated effluents from industrial wastewater treatment plants is a possible solution for the extraction of toxic organics. Nanocrystals of TiO 2 possess a high surface-area-to-volume ratio, making them suitable for photocatalysis and adsorption 196 , 197 . This means that nano-TiO 2 has a higher number of delocalized carriers on its surface, which ensures better-charged transport and efficient generation and separation of photo-generated electrons and holes. Photo-generated holes in TiO 2 nanocrystals are powerful oxidants 198 . Nanocrystals exhibit these properties because of their low dimensionality and quantum size effects 199 . TiO 2 is an n-type semiconductor with a relatively wide bandgap, and has three crystalline phases: rutile (tetragonal), brookite (orthorhombic), and anatase (tetragonal). TiO 2 has become the most popular photocatalyst at the nanoscale, and a lot of energy can be saved with photocatalysis without secondary pollution through process control. The types of TiO 2 nanostructures include: nanoparticles, nanotubes, nanorods, nanofibers, nanoflowers, and nanowires, in accordance with the preparation method and desired characteristics 200 . Nano-TiO 2 can be prepared through sol-gel 201 , hydrothermal 202 , solvothermal 203 , anodic oxidation 204 , hard template 205 , and reverse microemulsion 206 methods.
Nano-TiO 2 prepared from a sol-gel method has been used for phenol degradation 207 . Phenols possess endocrine-disrupting properties. Zeng et al. synthesized the nano-TiO 2 via the sol-gel process 208 . Titanium n-butoxide was dissolved in anhydrous ethanol to obtain the solution A. DI water, glacial CH 3 COOH, and C 2 H 6 O were mixed to obtain solution B. Solution A was mixed with solution B to get the sol. The sol was aged for 72 h and then dried at 100 °C and annealed. Regular sizes of anatase nano-TiO 2 were obtained. The TiO 2 was doped with B, Ni, and Ce for increased phenol degradation under visible light illumination. The best performance was attributed to BNiCeTiO 2 . In the work of Liu et al. 209 , porous TiO 2 hollow aggregates were synthesized through the hydrothermal method for the photocatalytic degradation of Rhodamine B. NH 4 F and Ti(SO 4 ) 2 were dissolved in DI water, and then the mixture was added to a Teflon-lined autoclave. Hydrothermal synthesis was carried out at 160 °C for 6 h. It was observed that the obtained photocatalyst were more effective than the commercially available photocatalyst P25 for Rhodamine B degradation.
Nano-TiO 2 has also been used for chloroform decomposition. In the work of Kang et al. (2001), C 12 H 28 O 4 Ti was dispersed in 1,4-butanediol under 300 °C for 50 min 210 . The anatase powder was efficient in chloroform degradation. In chloroform decomposition under the UV-light (254 nm, 24 W m –2 ) with O 2 bubbling (500 mL min –1 ), more than 95% of the chloroform was removed. Nano-TiO 2 has been employed for ethylene decomposition. In the work of Praserthdam et al., titanium n-butoxide was added to toluene 211 . The mixture was autoclaved at 300 °C for 2 h. Spherical shaped particles obtained promoted ethylene decomposition. A high amount of Ti 3+ surface defect with Ti 3+ /OH was found in the TiO 2 sample that was quenched in the air at 77K. The sample also exhibited the highest photocatalytic activity for ethylene decomposition. However, despite its versatility for photodegradation of trace pollutants in water, the use of nano-TiO 2 still faces some limitations 212 . Currently, the exploitation of readily available visible light for photodegradation via nano-TiO 2 is still inefficient for large-scale treatment, as most studies on large-scale TiO 2 photocatalysis have focused on the use of UV light 213 . Secondly, there is a low adsorption capacity of nano-TiO 2 for hydrophobic contaminants because TiO 2 is hydrophilic 214 . Therefore, the efficiency of removing hydrophobic contaminants through nano-TiO 2 structures is low. Thirdly, there is inadequate post-recovery of TiO 2 particles after treatment in water. The process of regenerating the particles after dispersion in water might be tedious and costly. Fourthly, there might be the production of toxic byproducts after the degradation of the primary contaminants by nano-TiO 2 215 . Although these byproducts might subsequently be removed by further photo-degradation, they remain in trace amounts in the final effluents in many cases. Lastly, many nano-TiO 2 structures lack mechanical strength or stabilization for long-time utilization in production plants. These structures would become fractured or destabilized under continuous feed system after some time. In case of polymer membranes, there is a danger of destruction of the membrane structure by UV light or OH • and problems of high cost 216 .
More recent progress in applications of TiO 2 and other photocatalysts is captured in the reviews of Giwa and co-authors 217 , 218 , 219 . This progress is mainly in the aspects of artificial neural network (ANN) modeling, plasma activation, functionalization with quantum dots, and use of nanoreactors. ANN modeling has been used to predict the discoloration of maxilon blue 5G dye by catalysts including TiO 2 , ZnO, and TiO 2 –ZnO integrated with Fe 220 . A Multilayer Perceptron neural model consisting of backpropagation algorithm was employed to assess the influence of operating conditions on the discoloration efficiency. An ANN model has also been employed for the prediction of the efficiency of a photocatalyst consisting of TiO 2 and Ag/S for 2-nitrophenol degradation 221 . The degradation efficiency of 2-nitrophenol was considered as the output variable in this model. The ability of TiO 2 nanoparticles to oxidize phenol in a system integrated with photo-electro-Fenton process has been predicted via another ANN model 222 . This model was coupled with genetic algorithm and optimum phenol removal efficiency was predicted by varying operating conditions such as phenol concentration and pH. Photocatalytic disinfection of water has been predicted using ANN modeling. Two back-propagation neural networks were employed by Lin et al. to assess the efficiency of TiO 2 coupled with UV for coliform removal from wastewater 223 . Input variables including the intensity of UV, coliform counts, color and turbidity, temperature and pH were included in the data that was used to train and evaluate the model. The model was also validated experimentally.
On the aspect of plasma activation, photocatalysts can be combined with plasmonic metals including gold and silver to enhance the transfer of incident photon energy to the photocatalysts and improve their ability to degrade organic pollutants under visible light conditions. Ag-AgI plasmonic photocatalyst has been synthesized by Hu et al. and used to degrade chlorophenolic compounds in water 224 . The plasmonic photocatalyst exhibited better efficiency than undoped TiO 2 P25 nanoparticles under visible (or simulated solar) light conditions. An azo dye, Reactive Brilliant Red, has also been removed from water by Jie et al. using plasmonic Ag/AgCl/polydopamine-TiO 2 photocatalysts 225 . The ability of the synthesized plasmonic photocatalyst to degrade Reactive Brilliant Red in water was four times higher than that of pure TiO 2 nanofibers.
On the aspect of functionalization of nanostructured photocatalysts with quantum dots, nanostructured TiO 2 has been functionalized with Ag 2 S quantum dots to improve the photocatalytic efficiency of nanostructured TiO 2 . Quantum dots exhibit the potential to improve the active sites on a catalyst surface, due to their small sizes, and tuning of the energy bandgap to the region required for visible-light photocatalysis. Visible light is abundant in form of natural solar light in the environment, so visible light-driven photocatalysis may be cheaper than UV or near-infra red-driven photocatalysis. The removal of methyl orange pollutant from water by TiO 2 /Ag 2 S quantum dots was reported to be 3.5 times higher than that of undoped TiO 2 nanobelts under visible light irradiation 226 . Other ways to improve the performance of photocatalysts under visible light conditions are well-documented in the work of Giwa et al. [268]. These ways involve the use of visible light-responsive nanocubes including reduced graphene oxide/mesoporous copper ferrite aerogel, porous Fe 2 O 3 nanocube-impregnated graphene aerogel, Au/Au/Ag nanocubes/polyvinylchloride substrate, and 3D/2D In 2 O 3 nanocube/ZnIn 2 S 4 nanosheet. These nanocubes are preferred due to their high adsorption ability, which influences their effective application under the visible range of the solar spectrum.
It is often problematic to use photocatalysts to remove organic pollutant in saline water due to the inference of salts on the photocatalytic process. An emerging technique, which has been tested for degradation of pollutants in seawater, is the use of nanoreactors. In nanoreactors, photocatalysts are synthesized by the adsorbed-layer nanoreactor synthesis (ANS). ANS improves photocatalysis by selective adsorption. ANS and solvothermal synthesis have been employed by Wang et al. to synthesize La 3+ or Yb 3+ - doped TiO 2 for the removal of phenol from simulated saline water 227 . Photocatalyst crystal lattice distortion and oxygen vacancies required for enhanced photocatalysis efficiency were impacted by ANS. About 90% removal of phenol was achieved. This high removal efficiency was as a result of enhanced adsorption, leading to a reduction in the adverse effect of the salt ions in the saline water. Acid fuchsine, a printing dye, has also been removed using zero-valent iron nanoparticles on polyacrylic acid/polyvinyl alcohol fiber mat 228 . The mat was employed as the nanoreactor. The synthesized nanoreactor showed superior activity when used to decolorize dye wastewater.
Hybrid systems
Hybrid systems consist of two or more treatment methods used together to provide better energy and treatment efficiencies, and/or to overcome the challenges associated with using stand-alone technology. The subsequent sections highlight the recent developments of such hybrid systems for wastewater treatment.
Adsorption on AC/MBR
The hybrid AC/MBR system integrates biological activity, membrane separation, and adsorption on PAC or minerals for the removal of pollutants. An application of this process is the treatment of sugarcane molasses-based distillery wastewater.
The vermiculite/MBR process resulted in a combined attached and suspended growth system since biomass growth occurred both in the mixed liquor and on the surface of vermiculite. Furthermore, it resulted in the removal of 88% Cu, 85% Zn, and 60% Ni for influent metal concentrations in the range of 3-15 mg L −1 , which were periodically spiked to municipal wastewater. Also, the addition of vermiculite in the bioreactor mitigated the inhibition of autotrophic and heterotrophic biomass 229 . Another process that utilized MBR, specifically zeolite/AnMBR process, was tested for the treatment of dyeing wastewater. The zeolite addition gave a reduction in the membrane filtration resistance and improved the decolorizing rate of the system 230 .
Combined filtration – adsorption
The integrated adsorption–filtration process has been successfully applied to remove heavy metals from industrial wastewater. The combined mineral-UF system for industrial pre-wastewater treatment at pH = 8 resulted in effective removal of Pb, Cu, Ni and Zn using vermiculite, bentonite, and zeolite as minerals 21 . Another combined adsorption-UF process was also tested for the treatment of textile wastewater. The examined process produced an effluent with a low metal concentration that can safely and efficiently be cleared into municipal sewers. The COD removal was between 76% to 92%, and color removal ranging between 45% and 70% 21 . This process was also applied to treat several different industrial wastewater streams from the chemical, metal plating, and textile industries. The performance of this combined process in terms of heavy metal removal varied according to the wastewater characteristics, and the type of sorbent used [24]. This adsorption-UF process was also tested for the removal of heavy metals from electroplating wastewater, which resulted in more 97% heavy metal removal at a pH greater than 8 due to combined adsorption and UF.
Combined EC-EF treatment
EC is an efficient method for the removal of suspended and colloidal particles, but ineffective to remove persistent organic compounds. On the other hand, EO is very effective in breaking down organic compounds by oxidation, but it necessitates more time than EC (30 min vs 21 h). As a result, the applicability of EO is limited. Hence, a synergistic combination of the two technologies can be used to achieve the removal of persistent organic compounds at a reasonable period. The electrooxidative mineralization of electrocoagulated wastewater reduces the treatment time 134 . In the hybrid technology, EC removes colloids, TSS, and charged species while EO oxidizes the remaining persistent organics. In the case of EC treatment alone, there was a drastic drop in the COD for the initial 30 min of treatment, in which a restrictive value was reached and no additional increase in efficiency was observed 134 . When EO was applied alone, the sample was totally mineralized after 21 h of treatment. About 3 hours were needed to reduce the COD to about half with EO. In the combined system, the initial feed of wastewater for EO was electrocoagulated wastewater (COD = 425 mg L −1 and pH 8). At a pH of 8, almost a complete removal of COD was achieved after 70 min of treatment. Also, the fastest rate for total mineralization by EO was found to occur at pH 8 134 .
Photocatalytic membrane reactors
Photocatalytic membranes (PMRs) are hybrid reactors in which photocatalysis is coupled with membrane process; the catalyst can be immobilized on a membrane (i.e., photocatalytic membrane) or suspended in the mixture 216 . Recently, nano-TiO 2 has been shown to be effective for the photodegradation of contaminants in the PMRs using entrapped or suspended catalyst 216 . The schematic of a PMR is shown in Fig. 4 .
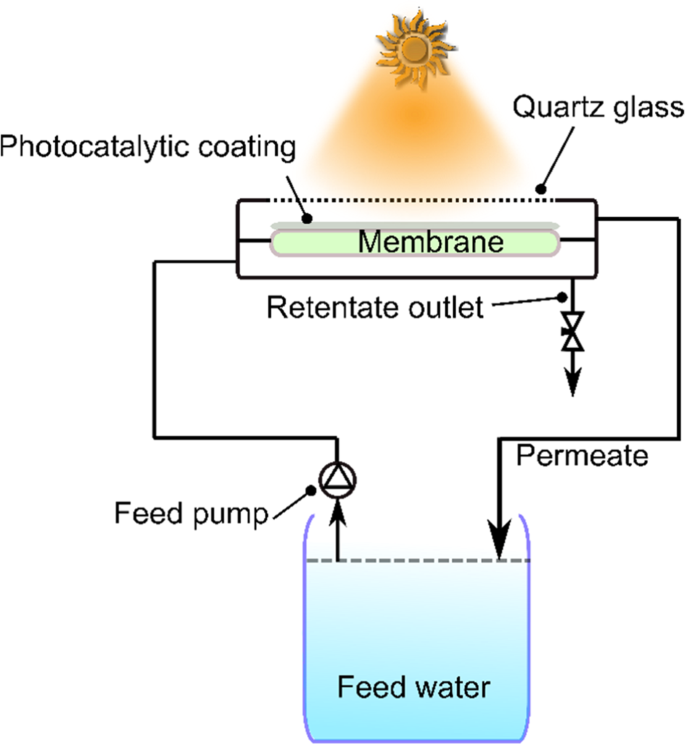
An illustration of lab-scale PMR system operating via recirculating batch mode (inspired from 309 ).
PMRs have been employed to remove various pollutants present in industrial wastewater, such as pharmaceuticals 121 , dyes 231 , textile and wood processing 232 , organics such as phenols 231 , 233 , and others 234 , 235 . The main advantages of PMRs with TiO 2 immobilized on the membrane are the reduction in fouling due to the presence of TiO 2, and the ability to use the membrane without recycling and regenerating the catalyst. On the other hand, some disadvantages include a lower degradation efficiency when compared to suspended TiO 2 processes, a necessity to change the membrane when the catalyst’s activity is lowered, a risk of membrane damage by UV light or OH • , and inability to change the catalyst’s loading due to the fixed amount of immobilized catalyst on the membrane 216 . A pilot-scale PMR employing UV/TiO 2 photocatalysis has been applied recently to remove some of the pollutants that can be present in industrial effluents 121 . The removal of 32 different pharmaceuticals, endocrine disrupting compounds, and estrogenic activity was evaluated. The degradation of all compounds followed pseudo-first-order kinetics. More than 70% of 29 compounds were removed while 50% of 3 compounds were removed 121 .
Most of the applications that combine photocatalysis with pressure-driven membrane processes employ MF or UF 216 . The application of a hybrid RO/photocatalysis system is limited since RO should not be used when the feed contains SS. Considering the above, more studies should be conducted on the possible applications of the RO/photocatalysis system. Some studies should have been carried out to find better catalysts that can enhance the catalytic effect 236 , 237 , 238 . Future research should be directed towards the modifications of the substrate to lower the bandgap required to activate the whole generation sites in PMRs.
Recently, a “fungal membrane bioreactor” (FMBR) using Phanerochaete chrysosporium was integrated with PMR for the treatment of textile industry wastewater 239 . It was observed that COD abatement and color removals were 53% and 58% for photocatalytic degradation, respectively, while the values were 56% and 60% for fungal biodegradation. The hybrid process achieved total removal efficiencies of 93% and 99% for color and COD, respectively. These results suggest that using photocatalysis as a post-treatment technique to the fungal biodegradation process is more effective than applying photocatalysis as a pre-treatment technique for the advanced treatment in the textile industry wastewater.
Sonication or hydrodynamic cavitation-assisted AOP
Hot spots, highly reactive free radicals, and liquid-circulation associated turbulence, can be used for the intensification of various physical/chemical operations 240 . Hydrodynamic cavitation ensures bubble dynamics and creates optimum operating parameters in reactor configurations. The use of this method may reduce chemical consumption, but it may be costlier. It has been shown that it is an energy-efficient method when compared with ultrasonication and high-speed homogenization 241 . Recently, the oxidants obtained from sonication have provided positive feedback 242 . Although the chemical process gave higher removal efficiency, the chemical-free sonication process is more environmentally friendly. For example, the use of Fe 2+ increased the degradation of 1,4-dioxane by 98.1%, but 79% removal efficiency was obtained when sonication was employed for the removal of 1,4-dioxane from water 242 .
The integration of photocatalysis and sonication has been used to achieve high removal efficiency of dye. The behavior of the hybrid method can be explained by two main reasons: (1) production of OH • from H 2 O 2 by photocatalyst, and (2) improvement of mass transfer between the liquid and the surface of catalyst as a result of sonolysis 243 .
Hydrodynamic cavitation has been used together with OH • for the degradation of Escherischia coli (E. Coli) 244 . A logarithmic equation based on the survived cells was used to calculate the dying-off rate of the E. Coli . It was observed that the OH • breaks the carbon bonds in the cell, therefore breaking its DNA chain and resulting in its eventual death. The disinfection property of H 2 O 2 also decreased protein synthesis in the cell. It was observed that the intensity and efficiency of the process are highly dependent on the cavitation field, energy consumed, gas content, and the initial concentration of the microbes. This positive effect of hydrodynamic cavitation on E. Coli was also confirmed by 245 , where it was shown that the concerned bacteria stopped dividing after only 3 min of treatment. However, further analysis showed that these bacteria did not actually die, but they were in an inactive state.
Hydrodynamic cavitation has been used to degrade volatile organic compounds such as ethylbenzene, toluene, benzene, and xylenes 246 . The highest degradation was achieved by toluene, i.e. 21% in 240 min. The conversion rate of the pollutants was influenced by an increase in the diameter of the hydrodynamic pipe. Sonication has also been used to remove polyaromatic hydrocarbon from industrial wastewater 247 . Low and high-molecular-weight pollutants were degraded. Meanwhile, it was observed that a rise in temperature played a significant role in improving the removal efficiency. In addition, hydrodynamic cavitation has been used recently to degrade carbamazepine 248 . 96% degradation was achieved in only 15 min by using hydrodynamic acoustic cavitation, as compared to 27% degradation when only hydrodynamic cavitation was employed 249 . A batch mode sonication was implemented on olive mill factory wastewater by 250 . The main observations in this study were as follows: (1) the increase in the initial temperature affects the degradation rates, and (2) COD was not entirely removed due to an insufficient amount of formed OH • . The study also emphasized the economic impact of using sonication for the treatment of olive mill factory wastewater. The total cost of sonication was estimated to be 665 Euros m −3 yr −1 . The nature and extent of pollutants removed by sonication and hydrodynamic cavitation-assisted AOPs are shown in Table 7 .
Electrically-enhanced membrane bioreactor (eMBR)
Reduction of membrane fouling and enhancing effluent’s quality is possible with using an electrically-enhanced membrane bioreactor (eMBR), a wastewater treatment technology that combines electrochemical processes, biodegradation, and membrane filtration in one system 251 . A schematic is shown in Fig. 5 . The electrochemical treatment works on increasing pollutants removal efficiency and controlling the mobility of foulants and their deposition on the membrane surface via electrochemical mechanisms, such as electrophoresis, electrocoagulation, and electroosmosis. Various metal species in the system are released due to electric dissociation, which can contribute to the aggregation and destabilization of collides and suspended solids through the coagulation process 136 . The metal species characteristics depend on the pH of the media. Moreover, extracellular polymeric substances and extracellular polymeric substances can be transported to the oppositely charged electrodes, away from the membrane, via electrophoretic motion 198 . This helps in reducing membrane fouling and the formation of biofilms.
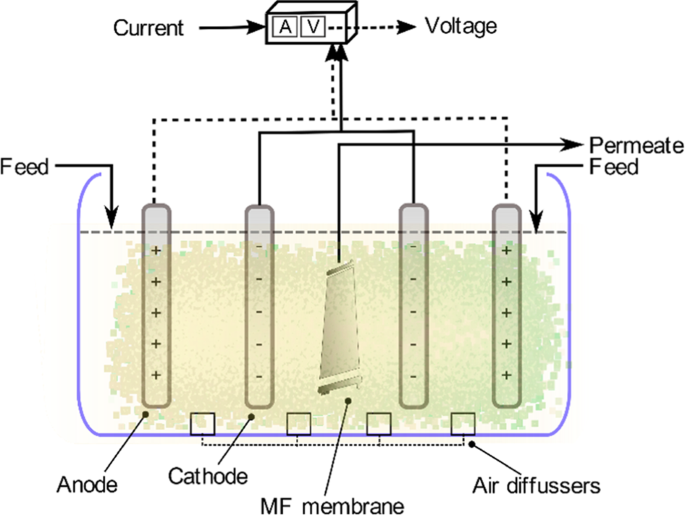
An overview of an eMBR system.
eMBR has been employed to remove organics (COD), nutrients, color, and turbidity 252 , 253 . Most of the conducted studies were performed at a lab-scale. Also, most of the experimental studies were limited to low-to-medium strength wastewater 252 .
The concept of circular economy in metal recovery
Circular economy is defined as a transition in which the generation of waste is minimized while resources, material, and products are maintained in the economy for extended period and utilized as much as possible 254 . The concept of circular economy has come into place in response to the drawbacks of the conventional ‘take-make-consume-and dispose’ model. The idea of a circular economy can be applied to wastewater treatment by metal recovery via electroplating, galvanizing, and anodizing and its utilization in various applications. For instance, aluminum ores contain ~30% alumina whereas wastewater sludge may contain up to 10–15% 255 . Conventional metal recovery methods include physical (e.g., ion exchange by ED, membrane filtration, etc.), chemical (e.g., precipitation, electrochemical methods, etc.), and biological (e.g., biosorption, bioremediation, etc.) methods. However, these methods are energy and chemical-intensive. Biological processes, for instance, have been used intensively for heavy metals extraction. Most heavy metals are easily adsorbed onto membrane lips and proteins such as phospholipids, peptidoglycan, lipopolysaccharides, teichoic acids, and teichuronic, in addition to anionic functional groups that are present in EPS. Most of the biovolume fraction is unoccupied since it is a surface process, which limits the potential of heavy metal immobilization. Nevertheless, by some kind of chemical transformation inside the cells, fungi, and bacteria can actively bioaccumulate heavy metals. Furthermore, the biological recovery of radionuclides is primarily driven by biosorption. Uranium has been previously immobilized by using organisms such as Rhodotorula glutinis 256 . Previous comprehensive review papers have addressed metal extraction using biological methods 256 . One of the challenges of using biological methods for metals extraction is the relatively low concentration of metals in wastewater effluents, which necessitates a step of pre-concentration of metals by nanofiltration, electrodialysis or reverse osmosis for instance. These technologies are costly especially at large scale treatment, which is an issue that future studies should address. Membrane filtration, for example, includes MF, UF, NF, and RO, which physically retain metals while allowing water to pass through. Chemical sorbents can also be used to modify membranes to increase the removal selectively of metal ions. Polycysteine functionalized MF membranes were efficient in removing Hg and Cd. However, the main disadvantages associated with using membrane technology for heavy metal recovery are membrane fouling, high energy consumption, and the high operational cost. On the other hand, ion exchange by ED requires solid resin to exchange the metals in wastewater with other cations such as H + . The solid resins are not selective; however, they can preferentially bind ions. Additionally, although ion exchange has fast kinetics and high removal efficiency, it is not suitable for high concentrations of metal ions due to resins saturation. Another technique that is widely used for metals recovery is chemical precipitation, due to its simple operation and low capital cost. However, the challenge associated with chemical precipitation is toxic sludge generation, which requires additional processing for disposal 257 . The recent development of bioelectrochemical technologies offers a platform for both oxidation and reduction related reactions, which provides an alternative approach for efficient metal recovery. These technologies employ microorganisms to produce chemical and electric current by utilizing the chemical energy stored in biodegradable materials. Many recent studies have discussed using bioelectrochemical technology in metal recovery from wastewater 257 .
Concluding remarks and future perspectives
A comprehensive review of various technologies for industrial wastewater treatment has been carried out, with a particular focus on effluents with high heavy metal content. The development of technologies for industrial wastewater treatment that can remove toxic pollutants is pivotal for meeting the growing water demands and for providing water security. As can be seen from this review, the focus of most research is to develop membranes and nanomaterials for water treatment, to optimize operating conditions of existing technologies, to investigate the potential use of low-cost adsorbents, and to develop hybrid technologies for water recycle and reuse. Recent breakthroughs in membrane technologies have emerged as significant innovations for the treatment and reclamation of industrial wastewater. Membrane bioreactors and low-pressure membranes have been used for wastewater treatment; however, the biggest challenge with these technologies is their high potential of fouling, which leads to reduced efficiency and shortened membrane life. Other technical problems include the complexity and cost of residuals disposal, especially when using membrane technologies. Adsorption is another method that is recently preferred for the removal of low concentrations and non-degradable organic compounds. It has advantages over the other methods due to its simple design and low initial costs. Recently, researchers have been investigating low-cost adsorbents such as natural materials, agricultural and industrial wastes, and others. The physio-chemical treatment processes are effective and quick; however, the high chemical and operational costs associated with them as well as sludge generation limit their applications. It can also be concluded that the treatment process is strongly dependent on the characteristics of wastewater. Also, although many techniques can be employed for industrial effluent treatment, each technology has its drawbacks. Thus, the selection of the most suitable treatment method depends on some parameters such as pH, initial pollutants concentration, the types of targeted pollutants, potential environmental impacts, as well as the process economic feasibility.
Membrane-based processes offer potential performance benefits, especially when coupled with electrochemical advanced oxidation procedures such as photoelectron-catalysis, electro-Fenton and electro-catalysis, and other processes. However, membrane fouling remains a big challenge. Also, utilizing membrane biofilms for the transformation of emerging chemicals should be explored. In doing so, the microbial strains have to be carefully selected, and operating conditions have to be precisely optimized such that the growth of biofilm on the membranes does not cause an increase in trans-membrane pressure. Future studies should aim to develop genetically engineered cultures to improve microbial strains to exhibit desirable characteristics that help reduce membrane fouling. Moreover, more research should focus on developing anti-fouling membranes specifically for MBRs as there is only limited work on this aspect. Efficient membrane cleaning techniques should be developed, incorporating recent advances in biochemical- and sonication-enabled cleaning strategies. Integrating self-cleaning membranes should also be investigated. Adsorption systems should be fully integrated with the existing treatment technologies such that synergies could be tapped on in ensuring the treated water quality standards. Another area to focus on is the green regeneration techniques to increase the recyclability of adsorbents. Similarly, the selectivity of the adsorbents may be further fine-tuned to capture emerging contaminants, which are otherwise leaked through the system untreated. Similarly, in the case of advanced oxidation processes, hydrogen peroxide dosage should be optimized such that excess of them do not scavenge the free radicals in the system and reduce the overall efficiency of the pollutant degradation. The toxicity of the by-products must also be considered. The potential of coupling should be investigated to tackle the growing concerns of emerging pollutants and toxic by-products. This requires additional studies for finding the best couplings and optimizing operating conditions to maximize the capabilities of these advanced treatment technologies. Research should also focus on integrating these processes within the existing treatments plants infra-structure with minimum disruption to the plant operations.
Data availability
The authors declare that the data supporting the findings of this study are available within the manuscript. Further data can be requested (if need be) by contacting the corresponding author.
Water, U. N. Water and Jobs . The United Nations World Water Development Report 2016 https://www.unwater.org/publications/world-water-development-report-2016/ (2016).
Mutamim, N. S. et al. Membrane bioreactor: Applications and limitations in treating high strength industrial wastewater. Chem. Eng. J. 225 , 109–119 (2013).
Article CAS Google Scholar
Lin, H. et al. Membrane bioreactors for industrial wastewater treatment: a critical review. Crit. Rev. Environ. Sci. Technol. 42 , 677–740 (2012).
Hai, F. I., Yamamoto, K. & Lee, C.-H. Membrane Biological Reactors Theory, Modeling, Design, Management and Applications to Wastewater Reuse . (IWA Publishing, 2013).
Smythe, G., Matelli, G., Bradford, M. & Rocha, C. Biological treatment of salty wastewater. Environ. Prog. 16 , 179–183 (1997).
UN. Wastewater management a UN-water analytical brief Analytical Brief . UN Water (2014).
UNESCO. Water in a changing world . The United Nations World Water Development Report 3. United Nations Educational, scientific and Cultural Organization (2009).
Ferella, F., De Michelis, I., Zerbini, C. & Vegliò, F. Advanced treatment of industrial wastewater by membrane filtration and ozonization. Desalination 313 , 1–11 (2013).
Eccles, H. Treatment of metal-contaminated wastes: Why select a biological process? Trends Biotechnol. 17 , 462–465 (1999). vol.
Barakat, M. A. New trends in removing heavy metals from industrial wastewater. Arab. J. Chem. 4 , 361–377 (2011).
Zhao, M., Xu, Y., Zhang, C., Rong, H. & Zeng, G. New trends in removing heavy metals from wastewater. Appl. Microbiol. Biotechnol. 100 , 6509–6518 (2016).
Adib, H., Hassanajili, S., Sheikhi-Kouhsar, M. R., Salahi, A. & Mohammadi, T. Experimental and computational investigation of polyacrylonitrile ultrafiltration membrane for industrial oily wastewater treatment. Korean J. Chem. Eng. 32 , 159–167 (2014).
Article Google Scholar
Deowan, S. A. et al. Novel low-fouling membrane bioreactor (MBR) for industrial wastewater treatment. J. Memb. Sci. 510 , 524–532 (2016).
Qdais, H. A. & Moussa, H. Removal of heavy metals from wastewater by membrane processes: a comparative study. Desalination 164 , 105–110 (2004).
Mohsen-Nia, M., Montazeri, P. & Modarress, H. Removal of Cu 2+ and Ni 2+ from wastewater with a chelating agent and reverse osmosis processes. Desalination 217 , 276–281 (2007).
Galambos, I., Mora Molina, J., Járay, P., Vatai, G. & Bekássy-Molnár, E. High organic content industrial wastewater treatment by membrane filtration. Desalination 162 , 117–120 (2004).
Barakat, M. A. & Schmidt, E. Polymer-enhanced ultrafiltration process for heavy metals removal from industrial wastewater. Desalination 256 , 90–93 (2010).
Juang, R. S. & Shiau, R. C. Metal removal from aqueous solutions using chitosan-enhanced membrane filtration. J. Memb. Sci. 165 , 159–167 (2000).
Trivunac, K. & Stevanovic, S. Removal of heavy metal ions from water by complexation-assisted ultrafiltration. Chemosphere 64 , 486–491 (2006).
Aroua, M. K., Zuki, F. M. & Sulaiman, N. M. Removal of chromium ions from aqueous solutions by polymer-enhanced ultrafiltration. J. Hazard. Mater. 147 , 752–758 (2007).
Katsou, E., Malamis, S. & Haralambous, K. J. Industrial wastewater pre-treatment for heavy metal reduction by employing a sorbent-assisted ultrafiltration system. Chemosphere 82 , 557–564 (2011).
Malamis, S., Katsou, E., Kosanovic, T. & Haralambous, K. J. Combined adsorption and ultrafiltration processes employed for the removal of pollutants from metal plating wastewater. Sep. Sci. Technol. 47 , 983–996 (2012).
Malamis, S., Katsou, E., Stylianou, M., Haralambous, K. J. & Loizidou, M. Copper removal from sludge permeate with ultrafiltration membranes using zeolite, bentonite and vermiculite as adsorbents. Water Sci. Technol. 61 , 581–589 (2010). vol.
Katsou, E., Malamis, S., Haralambous, K. J. & Loizidou, M. Use of ultrafiltration membranes and aluminosilicate minerals for nickel removal from industrial wastewater. J. Memb. Sci. 360 , 234–249 (2010).
Afonso, M. D. & Bórquez, R. Review of the treatment of seafood processing wastewaters and recovery of proteins therein by membrane separation processes — prospects of the ultrafiltration of wastewaters from the fish meal industry. Desalination 142 , 29–45 (2002).
Turano, E., Curcio, S., De Paola, M. G., Calabrò, V. & Iorio, G. An integrated centrifugation–ultrafiltration system in the treatment of olive mill wastewater. J. Memb. Sci. 209 , 519–531 (2002).
Lester, N. Heavy Metals in Wastewater and Sludge Treatment Processes . vol. 2 (CRC Press, 1987).
Santos, A. et al. Fate and behaviour of copper and zinc in secondary biological wastewater treatment processes: II Removal at varying sludge age. Environ. Technol. 31 , 725–743 (2010).
Clara, M., Kreuzinger, N., Strenn, B., Gans, O. & Kroiss, H. The solids retention time—a suitable design parameter to evaluate the capacity of wastewater treatment plants to remove micropollutants. Water Res 39 , 97–106 (2005).
Noble, J. GE ZeeWeed MBR technology for pharmaceutical wastewater treatment. Membr. Technol. 2006 , 7–9 (2006).
Scholz, W. & Fuchs, W. Treatment of oil contaminated wastewater in a membrane bioreactor. Water Res 34 , 3621–3629 (2000).
Kim, B. R. et al. Design and startup of a membrane-biological-reactor system at a Ford-engine plant for treating oily wastewater. Water Env. Res 78 , 362–371 (2006).
Di Fabio, S. et al. Are centralized MBRs coping with the current transition of large petrochemical areas? A pilot study in Porto-Marghera (Venice). Chem. Eng. J. 214 , 68–77 (2013).
Battistoni, P., Cola, E., Fatone, F., Bolzonella, D. & Eusebi, A. L. Micropollutants removal and operating strategies in ultrafiltration membrane systems for municipal wastewater treatment: Preliminary results. Ind. Eng. Chem. Res. 46 , 6716–6723 (2007).
Nelson, P. O., Chung, A. K. & Hudson, M. C. Factors affecting the fate of heavy metals in the activated sludge process. J. Water Pollut. Control Fed. 53 , 1323–1333 (1981).
CAS Google Scholar
Fatone, F., Eusebi, A. L., Pavan, P. & Battistoni, P. Exploring the potential of membrane bioreactors to enhance metals removal from wastewater: Pilot experiences. Water Sci. Technol. 57 , 505–511 (2008). vol.
Robles, A., Ruano, M. V., Ribes, J. & Ferrer, J. Sub-critical long-term operation of industrial scale hollow-fibre membranes in a submerged anaerobic MBR (HF-SAnMBR) system. Sep. Purif. Technol. 100 , 88–96 (2012).
Tazi-Pain, A., Schrotter, J. C., Bord, G., Payreaudeau, M. & Buisson, H. Recent improvement of the BIOSEP® process for industrial and municipal wastewater treatment. Desalination 146 , 439–443 (2002).
Tao, Y., Gao, D. W., Fu, Y., Wu, W. M. & Ren, N. Q. Impact of reactor configuration on anammox process start-up: MBR versus SBR. Bioresour. Technol. 104 , 73–80 (2012).
Kanai, M., Ferre, V., Wakahara, S., Yamamoto, T. & Moro, M. A novel combination of methane fermentation and MBR — Kubota Submerged Anaerobic Membrane Bioreactor process. Desalination 250 , 964–967 (2010).
Saravanane, R. & Sundararaman, S. Effect of loading rate and HRT on the removal of cephalosporin and their intermediates during the operation of a membrane bioreactor treating pharmaceutical wastewater. Water Sci. Technol. 61 , 1907–1914 (2010).
Qu, Y. Y. et al. Population dynamics in bioaugmented membrane bioreactor for treatment of bromoamine acid wastewater. Bioresour. Technol. 100 , 244–248 (2009).
Shrout, J. D. & Nerenberg, R. Monitoring bacterial twitter: does quorum sensing determine the behavior of water and wastewater treatment biofilms? Env . Sci. Technol. 46 , 1995–2005 (2012).
Ergön-Can, T., Köse-Mutlu, B., Koyuncu, İ. & Lee, C.-H. Biofouling control based on bacterial quorum quenching with a new application: Rotary microbial carrier frame. J. Memb. Sci. 525 , 116–124 (2017).
Davies, D. G. et al. The involvement of cell-to-cell signals in the development of a bacterial biofilm. Sci. (80-.) 280 , 295–298 (1998).
Stuckey, D. C. Recent developments in anaerobic membrane reactors. Bioresour. Technol. 122 , 137–148 (2012).
Ren, T., Li, X. & Yu, H. Effect of N-acy-l-homoserine lactones-like molecules from aerobic granules on biofilm formation by Escherichia coli K12. Bioresour. Technol. 129 , 655–658 (2013).
Lade, H., Paul, D. & Kweon, J. H. Quorum quenching mediated approaches for control of membrane biofouling. Int J. Biol. Sci. 10 , 550–565 (2014).
Zhang, W. & Li, C. Exploiting quorum sensing interfering strategies in gram-negative bacteria for the enhancement of environmental applications. Front. Microbiol. 6 , 1535 (2015).
Google Scholar
Kim, S.-R. et al. Biofouling control with bead-entrapped quorum quenching bacteria in membrane bioreactors: physical and biological effects. Environ. Sci. Technol. 47 , 836–842 (2013).
Kim, H. W. et al. Microbial population dynamics and proteomics in membrane bioreactors with enzymatic quorum quenching. Appl Microbiol Biotechnol. 97 , 4665–4675 (2013).
Guo, W., Ngo, H.-H. & Li, J. A mini-review on membrane fouling. Bioresour. Technol. 122 , 27–34 (2012).
Malaeb, L., Le-Clech, P., Vrouwenvelder, J. S., Ayoub, G. M. & Saikaly, P. E. Do biological-based strategies hold promise to biofouling control in MBRs? Water Res 47 , 5447–5463 (2013).
Gnirss, R. & Dittrich, J. Microfiltration of Municipal Wastewater for Disinfection and Advanced Phosphorus Removal: Results from Trials with Different Small-Scale Pilot Plants. Water Environ. Res. 72 , 602–609 (2000).
Pellegrin, M. L. & Kinnear, D. J. MBR Energy Consumption: Comparing Operating Full-Scale Plants. in In Proceedings of 84th Annual Water Environment Federation Technical Exposition & Conference (ed. In Proceedings of 84th Annual Water Environment Federation Technical Exposition & Conference) 5544–5560 (Water Environment Federation, 2011).
K, M. & M, S. Benchmarking energy consumption in municipal wastewater treatment plants in Japan. Water Sci. Technol. 62 , 2256–2262 (2010).
Hua, M. et al. Heavy metal removal from water/wastewater by nanosized metal oxides: A review. J. Hazard. Mater. 211–212 , 317–331 (2012).
Worch, E. Adsorption Technology in Water Treatment: Fundamentals, Processes, and Modeling . (De Gruyter, 2012).
Adeleye, A. S. et al. Engineered nanomaterials for water treatment and remediation: Costs, benefits, and applicability. Chem. Eng. J. 286 , 640–662 (2016).
Doke, K. M. & Khan, E. M. Adsorption thermodynamics to clean up wastewater; critical review. Rev. Environ. Sci. Bio/Technol. 12 , 25–44 (2013).
Cecen, F. & Aktas, Ö. Activated Carbon for Water and Wastewater Treatment: Integration of Adsorption and Biological Treatment (1) . (Wiley-VCH, 2011).
Singh Vats, S., Tyagi, P. & Industrial, R. Wastewater treatment by bilogical activated carbon - a review. Res J. Pharm. Biol. Chem. Sci. 2 , 1053–1058 (2011).
Crini, G. Recent developments in polysaccharide-based materials used as adsorbents in wastewater treatment. Prog. Polym. Sci. 30 , 38–70 (2005).
Moreno-Castilla, C. Adsorption of organic molecules from aqueous solutions on carbon materials. Carbon N. Y. 42 , 83–94 (2004).
Al-Jiboury, K. Adsorption of phenol from industrial wastewater using commercial powdered activated carbon. ICOEST (2013).
Zhang, K., Gao, N., Deng, Y., Shui, M. & Tang, Y. Granular activated carbon (GAC) adsorption of two algal odorants, dimethyl trisulfide and β-cyclocitral. Desalination 266 , 231–237 (2011).
Sarabadan, M., Bashiri, H. & Mousavi, S. M. Removal of crystal violet dye by an efficient and low cost adsorbent: Modeling, kinetic, equilibrium and thermodynamic studies. Korean J. Chem. Eng. 2019 3610 36 , 1575–1586 (2019).
Zhou, Y.-F. & Haynes, R. J. Sorption of heavy metals by inorganic and organic components of solid wastes: significance to use of wastes as low-cost adsorbents and immobilizing agents. Crit. Rev. Environ. Sci. Technol. 40 , 909–977 (2010).
Malamis, S. & Katsou, E. A review on zinc and nickel adsorption on natural and modified zeolite, bentonite and vermiculite: Examination of process parameters, kinetics and isotherms. J. Hazard. Mater. 252–253 , 428–461 (2013).
Şölener, M., Tunali, S., Özcan, A. S., Özcan, A. & Gedikbey, T. European Desalination Society and Center for Research and Technology Hellas (CERTH), Sani Resort 22–25 April 2007, Halkidiki, GreeceAdsorption characteristics of lead(II) ions onto the clay/poly(methoxyethyl)acrylamide (PMEA) composite from aqueous soluti. Desalination 223 , 308–322 (2008).
Vhahangwele, M. & Mugera, G. W. The potential of ball-milled South African bentonite clay for attenuation of heavy metals from acidic wastewaters: Simultaneous sorption of Co 2+ , Cu 2+ , Ni 2+ , Pb 2+ , and Zn 2+ ions. J. Environ. Chem. Eng. 3 , 2416–2425 (2015).
Inglezakis, V. J., Stylianou, M. A., Gkantzou, D. & Loizidou, M. D. Removal of Pb(II) from aqueous solutions by using clinoptilolite and bentonite as adsorbents. Desalination 210 , 248–256 (2007).
Quan, D. X. et al. 2013 International Symposium on Environmental Science and Technology (2013 ISEST)Immobilization of Pb and Cd in Contaminated Soil Using Nano-Crystallite Hydroxyapatite. Procedia Environ. Sci. 18 , 657–665 (2013).
Amalhay, M. et al. SYMPHOS 2013 - 2nd International Symposium on Innovation and Technology in the Phosphate IndustryRemoval of Cadmium from Water Using Natural Phosphate as Adsorbent. Procedia Eng. 83 , 386–393 (2014).
Keleş, E., Özer, A. K. & Yörük, S. Removal of Pb 2+ from aqueous solutions by rock phosphate (low-grade). Desalination 253 , 124–128 (2010).
Ghahremani, D. et al. Potential of nano crystalline calcium hydroxyapatite for Tin(II) removal from aqueous solutions: Equilibria and kinetic processes. Arab. J. Chem . https://doi.org/10.1016/j.arabjc.2012.10.006 .
Barka, N., Qourzal, S., Assabbane, A., Nounah, A. & Ait-Ichou, Y. Removal of Reactive Yellow 84 from aqueous solutions by adsorption onto hydroxyapatite. J. Saudi Chem. Soc. 15 , 263–267 (2011).
Wei, W., Sun, R., Cui, J. & Wei, Z. Removal of nitrobenzene from aqueous solution by adsorption on nanocrystalline hydroxyapatite. Desalination 263 , 89–96 (2010).
Maretto, M. et al. Adsorption of hydrocarbons from industrial wastewater onto a silica mesoporous material: Structural and thermal study. Microporous Mesoporous Mater. 203 , 139–150 (2015).
Pollard, S. J. T., Fowler, G. D., Sollars, C. J. & Perry, R. Low-cost adsorbents for waste and wastewater treatment: a review. Sci. Total Environ. 116 , 31–52 (1992).
Zhang, F. S. & Itoh, H. Iron oxide-loaded slag for arsenic removal from aqueous system. Chemosphere 60 , 319–325 (2005).
Kanel, S. R., Choi, H., Kim, J. Y., Vigneswaran, S. & Shim, W. G. Removal of arsenic(III) from groundwater using low-cost industrial by-products - Blast furnace slag. Water Qual. Res. J. Can. 41 , 130–139 (2006).
Oh, C., Rhee, S., Oh, M. & Park, J. Removal characteristics of As(III) and As(V) from acidic aqueous solution by steel making slag. J. Hazard. Mater. 213–214 , 147–155 (2012).
Duan, J. & Su, B. Removal characteristics of Cd(II) from acidic aqueous solution by modified steel-making slag. Chem. Eng. J. 246 , 160–167 (2014).
Balsamo, M. et al. Arsenate removal from synthetic wastewater by adsorption onto fly ash. Desalination 263 , 58–63 (2010).
Sun, D., Zhang, X., Wu, Y. & Liu, X. Adsorption of anionic dyes from aqueous solution on fly ash. J. Hazard. Mater. 181 , 335–342 (2010).
Ahmaruzzaman, M. Industrial wastes as low-cost potential adsorbents for the treatment of wastewater laden with heavy metals. Adv. Colloid Interface Sci. 166 , 36–59 (2011).
Mofarrah, A., Husain, T. & Chen, B. Optimizing Cr(VI) adsorption on activated carbon produced from heavy oil fly ash. J. Mater. Cycles Waste Manag. 16 , 482–490 (2014).
Visa, M., Andronic, L. & Duta, A. Fly ash-TiO 2 nanocomposite material for multi-pollutants wastewater treatment. J. Environ. Manag. 150 , 336–343 (2015).
Arief, V. O., Trilestari, K., Sunarso, J., Indraswati, N. & Ismadji, S. Recent Progress on Biosorption of Heavy Metals from Liquids Using Low Cost Biosorbents: Characterization, Biosorption Parameters and Mechanism. Stud. CLEAN – Soil, Air, Water 36 , 937–962 (2008).
Raj, K. R., Kardam, A., Arora, J. K., Srivastava, S. & Srivastava, M. M. Prediction of the As(III) and As(V) Abatement Capacity of Zea mays Cob Powder: ANN Modelling. Natl Acad. Sci. Lett. 36 , 41–47 (2013).
Rajeswari, M. et al. Continuous biosorption of cadmium by Moringa olefera in a packed column. Biotechnol. Bioprocess Eng. 18 , 321–325 (2013).
Tan, T. & Cheng, P. Biosorption of metal ions with Penicillium chrysogenum. Appl. Biochem. Biotechnol. 104 , 119–128 (2003).
Akar, T., Tunali, S. & Çabuk, A. Study on the characterization of lead (II) biosorption by fungus Aspergillus parasiticus. Appl. Biochem. Biotechnol. 136 , 389–405 (2007).
Ahmady-Asbchin, S., Andrès, Y., Gérente, C. & Cloirec, P. L. Biosorption of Cu(II) from aqueous solution by Fucus serratus: Surface characterization and sorption mechanisms. Bioresour. Technol. 99 , 6150–6155 (2008).
Panda, G. C., Das, S. K., Bandopadhyay, T. S. & Guha, A. K. Adsorption of nickel on husk of Lathyrus sativus: Behavior and binding mechanism. Colloids Surf. B Biointerfaces 57 , 135–142 (2007).
Witek-Krowiak, A., Szafran, R. G. & Modelski, S. Biosorption of heavy metals from aqueous solutions onto peanut shell as a low-cost biosorbent. Desalination 265 , 126–134 (2011).
Piotrowska-Niczyporuk, A., Bajguz, A., Zambrzycka, E. & Godlewska-Żyłkiewicz, B. Phytohormones as regulators of heavy metal biosorption and toxicity in green alga Chlorella vulgaris (Chlorophyceae). Plant Physiol. Biochem. 52 , 52–65 (2012).
Feng, N., Guo, X., Liang, S., Zhu, Y. & Liu, J. Biosorption of heavy metals from aqueous solutions by chemically modified orange peel. J. Hazard. Mater. 185 , 49–54 (2011).
Gomez, P. F. et al. Heavy metal biosorption by Rhizopus sp. biomass immobilized on textiles. Water Air Soil Pollut. 225 , 1834 (2014).
Al-Anber, Z. A. & Matouq, M. A. D. Batch adsorption of cadmium ions from aqueous solution by means of olive cake. J. Hazard. Mater. 151 , 194–201 (2008).
Rahman, M. S. & Sathasivam, K. V. Heavy metal adsorption onto Kappaphycus sp. from aqueous solutions: the use of error functions for validation of isotherm and kinetics models. Biomed. Res. Int. 2015 , 126298 (2015).
Malamis, S., Katsou, E. & Haralambous, K. J. Study of Ni(II), Cu(II), Pb(II), and Zn(II) removal using sludge and minerals followed by MF/UF. Water, Air, Soil Pollut. 218 , 81–92 (2011).
Putri, K. N. A., Keereerak, A. & Chinpa, W. Novel cellulose-based biosorbent from lemongrass leaf combined with cellulose acetate for adsorption of crystal violet. Int. J. Biol. Macromol. 156 , 762–772 (2020).
Pan, J., Gao, B., Song, W., Xu, X. & Yue, Q. Modified biogas residues as an eco-friendly and easily-recoverable biosorbent for nitrate and phosphate removals from surface water. J. Hazard. Mater. 382 , 121073 (2020).
Wang, J. & Chen, C. Chitosan-based biosorbents: modification and application for biosorption of heavy metals and radionuclides. Bioresour. Technol. 160 , 129–141 (2014).
Phetphaisit, C. W., Yuanyang, S. & Chaiyasith, W. C. Polyacrylamido-2-methyl-1-propane sulfonic acid-grafted-natural rubber as bio-adsorbent for heavy metal removal from aqueous standard solution and industrial wastewater. J. Hazard. Mater. 301 , 163–171 (2016).
Lee, S.-T., Mi, F.-L., Shen, Y.-J. & Shyu, S.-S. Equilibrium and kinetic studies of copper(II) ion uptake by chitosan-tripolyphosphate chelating resin. Polym. (Guildf.) 42 , 1879–1892 (2001).
Liu, X. D., Tokura, S., Nishi, N. & Sakairi, N. A novel method for immobilization of chitosan onto nonporous glass beads through a 1,3-thiazolidine linker. Polym. (Guildf.) 44 , 1021–1026 (2003).
Dotto, G. L. & McKay, G. Current scenario and challenges in adsorption for water treatment. J. Environ. Chem. Eng. 8 , 103988 (2020).
Poyatos, J. M. et al. Advanced oxidation processes for wastewater treatment: state of the art. Water Air. Soil Pollut. 205 , 187–204 (2009).
Bautista, P., Mohedano, A. F., Casas, J. A., Zazo, J. A. & Rodriguez, J. J. An overview of the application of Fenton oxidation to industrial wastewaters treatment. J. Chem. Technol. Biotechnol. 83 , 1323–1338 (2008).
Andreozzi, R., Caprio, V., Insola, A. & Marotta, R. Advanced oxidation processes (AOP) for water purification and recovery. Catal. Today 53 , 51–59 (1999).
Wang, Y. et al. Dimethyl phthalate degradation at novel and efficient electro-Fenton cathode. Appl. Catal. B Environ. 156–157 , 1–7 (2014).
EPA., U. S. Wastewater Technology Fact Sheet Ozone Disinfection. Off. Water Washington, D.C . (1999) doi:EPA 832-F-99-063.
Kommineni, S. et al. Advanced oxidation processes: literature review. Cent. Groundw. Restor. Prot. Natl. water Res. Inst . 110–208 (2000).
Umar, M., Aziz, H. A. & Yusoff, M. S. Trends in the use of Fenton, electro-Fenton and photo-Fenton for the treatment of landfill leachate. Waste Manag 30 , 2113–2121 (2010).
Brillas, E., Sirés, I. & Oturan, M. A. Electro-Fenton process and related electrochemical technologies based on Fenton’s reaction chemistry. Chem. Rev. 109 , 6570 (2009).
Panizza, M. & Cerisola, G. Electro-Fenton degradation of synthetic dyes. Water Res. 43 , 339–344 (2009).
Wang, C.-T., Chou, W.-L., Chung, M.-H. & Kuo, Y.-M. COD removal from real dyeing wastewater by electro-Fenton technology using an activated carbon fiber cathode. Desalination 253 , 129–134 (2010).
Benotti, M. J., Stanford, B. D., Wert, E. C. & Snyder, S. A. Evaluation of a photocatalytic reactor membrane pilot system for the removal of pharmaceuticals and endocrine disrupting compounds from water. Water Res 43 , 1513–1522 (2009).
Xia, Q., Jiang, Z., Li, D., Wang, J. & Yao, Z. Green synthesis of a dendritic Fe 3 O 4 @Feo composite modified with polar C-groups for Fenton-like oxidation of phenol. J. Alloys Compd . (2018) https://doi.org/10.1016/j.jallcom.2018.02.311 .
Liu, S. et al. The degradation of tetracycline in a photo-electro-Fenton system. Chem. Eng. J. 231 , 441 (2013).
Ferrag-Siagh, F. et al. Electro-Fenton pretreatment for the improvement of tylosin biodegradability. Environ. Sci. Pollut. Res. 21 , 8534–8542 (2014).
Komtchou, S., Dirany, A., Drogui, P. & Bermond, A. Removal of carbamazepine from spiked municipal wastewater using electro-Fenton process. Environ. Sci. Pollut. Res. 22 , 11513–11525 (2015).
Carra, I. et al. Performance of different advanced oxidation processes for tertiary wastewater treatment to remove the pesticide acetamiprid. J. Chem. Technol. Biotechnol. 91 , 72–81 (2015).
Babuponnusami, A. & Muthukumar, K. A review on Fenton and improvements to the Fenton process for wastewater treatment. J. Environ. Chem. Eng. 2 , 557–572 (2014).
Thirugnanasambandham, K. & Sivakumar, V. Optimization of treatment of grey wastewater using Electro-Fenton technique–Modeling and validation. Process Saf. Environ. Prot. 95 , 60–68 (2015).
Grčić, I., Vujević, D., Šepčić, J. & Koprivanac, N. Minimization of organic content in simulated industrial wastewater by Fenton type processes: A case study. J. Hazard. Mater. 170 , 954–961 (2009).
Navarro, R. R., Ichikawa, H. & Tatsumi, K. Ferrite formation from photo-Fenton treated wastewater. Chemosphere 80 , 404–409 (2010).
Li, R. et al. Removal of triazophos pesticide from wastewater with Fenton reagent. J. Hazard. Mater. 167 , 1028–1032 (2009).
Oturan, N., Brillas, E. & Oturan, M. A. Unprecedented total mineralization of atrazine and cyanuric acid by anodic oxidation and electro-Fenton with a boron-doped diamond anode. Environ. Chem. Lett. 10 , 165–170 (2012).
Iglesias, O., Meijide, J., Bocos, E., Sanromán, M. Á. & Pazos, M. New approaches on heterogeneous electro-Fenton treatment of winery wastewater. Electrochim. Acta 169 , 134–141 (2015).
Linares-Hernández, I., Barrera-Díaz, C., Bilyeu, B., Juárez-GarcíaRojas, P. & Campos-Medina, E. A combined electrocoagulation–electrooxidation treatment for industrial wastewater. J. Hazard. Mater. 175 , 688–694 (2010).
Chen, G. Electrochemical technologies in wastewater treatment. Sep. Purif. Technol. 38 , 11–41 (2004).
Mollah, M. Y. A., Schennach, R., Parga, J. R. & Cocke, D. L. Electrocoagulation (EC) — science and applications. J. Hazard. Mater. 84 , 29–41 (2001).
Calvo, L. S. et al. An electrocoagulation unit for the purification of soluble oil wastes of high COD. Environ. Prog. 22 , 57–65 (2003).
El-Naas, M. H., Al-Zuhair, S., Al-Lobaney, A. & Makhlouf, S. Assessment of electrocoagulation for the treatment of petroleum refinery wastewater. J. Environ. Manag. 91 , 180–185 (2009).
Şengil, İ. A., Kulaç, S. & Özacar, M. Treatment of tannery liming drum wastewater by electrocoagulation. J. Hazard. Mater. 167 , 940–946 (2009).
GilPavas, E., Dobrosz-Gómez, I. & Gómez-García, M. Á. The removal of the trivalent chromium from the leather tannery wastewater: the optimisation of the electro-coagulation process parameters. Water Sci. Technol. 63 , 385–394 (2011).
Espinoza-Quiñones, F. R. et al. Electrocoagulation efficiency of the tannery effluent treatment using aluminium electrodes. Water Sci. Technol. 60 , 2173–2185 (2009).
Moreno-Casillas, H. A. et al. Electrocoagulation mechanism for COD removal. Sep. Purif. Technol. 56 , 204–211 (2007).
Bensadok, K., Benammar, S., Lapicque, F. & Nezzal, G. Electrocoagulation of cutting oil emulsions using aluminium plate electrodes. J. Hazard. Mater. 152 , 423–430 (2008).
Karhu, M., Kuokkanen, V., Kuokkanen, T. & Rämö, J. Bench scale electrocoagulation studies of bio oil-in-water and synthetic oil-in-water emulsions. Sep. Purif. Technol. 96 , 296 (2012).
Ahmadi, S., Sardari, E., Javadian, H. R., Katal, R. & Sefti, M. V. Removal of oil from biodiesel wastewater by electrocoagulation method. Korean J. Chem. Eng. 30 , 634–641 (2013).
Hassan, I., Nirdosh, I. & Sedahmed, G. H. Separation of Oil from Oil–Water Emulsions by Electrocoagulation in an Electrochemical Reactor with a Fixed-Bed Anode. Water, Air, Soil Pollut. 226 , 1–12 (2015).
Safari, S., Azadi Aghdam, M. & Kariminia, H. R. Electrocoagulation for COD and diesel removal from oily wastewater. Int. J. Environ. Sci. Technol. 13 , 231–242 (2016).
Cañizares, P., Martínez, F., Jiménez, C., Sáez, C. & Rodrigo, M. A. Coagulation and electrocoagulation of oil-in-water emulsions. J. Hazard. Mater. 151 , 44–51 (2008).
Sahu, O., Mazumdar, B. & Chaudhari, P. K. Treatment of wastewater by electrocoagulation: a review. Environ. Sci. Pollut. Res. 21 , 2397–2413 (2014).
Cañizares, P., Martínez, F., Jiménez, C., Lobato, J. & Rodrigo, M. A. Comparison of the aluminum speciation in chemical and electrochemical dosing processes. Ind. Eng. Chem. Res. 45 , 8749–8756 (2006).
Cañizares, P., Martínez, F., Lobato, J. & Rodrigo, M. A. Break-up of oil-in-water emulsions by electrochemical techniques. J. Hazard. Mater. 145 , 233–240 (2007).
Zongo, I. et al. Electrocoagulation for the treatment of textile wastewaters with Al or Fe electrodes: Compared variations of COD levels, turbidity and absorbance. J. Hazard. Mater. 169 , 70–76 (2009).
Cheballah, K., Sahmoune, A., Messaoudi, K., Drouiche, N. & Lounici, H. Simultaneous removal of hexavalent chromium and COD from industrial wastewater by bipolar electrocoagulation. Chem. Eng. Process. Process. Intensif. 96 , 94–99 (2015).
Gao, P., Chen, X., Shen, F. & Chen, G. Removal of chromium(VI) from wastewater by combined electrocoagulation–electroflotation without a filter. Sep. Purif. Technol. 43 , 117–123 (2005).
Gatsios, E., Hahladakis, J. N. & Gidarakos, E. Optimization of electrocoagulation (EC) process for the purification of a real industrial wastewater from toxic metals. J. Environ. Manag. 154 , 117–127 (2015).
Hansen, H. K., Nuñez, P. & Jil, C. Removal of Arsenic from Wastewaters by Airlift Electrocoagulation. Part 1: Batch Reactor Experiments. Sep. Sci. Technol. 43 , 212–224 (2008).
Petsriprasit, C., Namboonmee, J. & Hunsom, M. Application of the electrocoagulation technique for treating heavy metals containing wastewater from the pickling process of a billet plant. Korean J. Chem. Eng. 27 , 854–861 (2010).
Khatibikamal, V., Torabian, A., Janpoor, F. & Hoshyaripour, G. Fluoride removal from industrial wastewater using electrocoagulation and its adsorption kinetics. J. Hazard. Mater. 179 , 276–280 (2010).
Sujana, M. G., Thakur, R. S. & Rao, S. B. Removal of fluoride from aqueous solution by using alum sludge. J. Colloid Interface Sci. 206 , 94–101 (1998).
Toyoda, A. & Taira, T. A new method for treating fluorine wastewater to reduce sludge and running costs. IEEE Trans. Semicond. Manuf. 13 , 305–309 (2000).
Raju, G. B., Karuppiah, M. T., Latha, S. S., Parvathy, S. & Prabhakar, S. Treatment of wastewater from synthetic textile industry by electrocoagulation–electrooxidation. Chem. Eng. J. 144 , 51–58 (2008).
Feng, J. et al. Treatment of tannery wastewater by electrocoagulation. J. Environ. Sci. 19 , 1409–1415 (2007).
Benhadji, A., Taleb Ahmed, M. & Maachi, R. Electrocoagulation and effect of cathode materials on the removal of pollutants from tannery wastewater of Rouïba. Desalination 277 , 128–134 (2011).
Kabdaşlı, I., Arslan-Alaton, I., Ölmez-Hancı, T. & Tünay, O. Electrocoagulation applications for industrial wastewaters: a critical review. Environ. Technol. Rev. 1 , 2–45 (2012).
Merzouk, B. et al. Effect of modification of textile wastewater composition on electrocoagulation efficiency. Desalination 275 , 181–186 (2011).
Secula, M. S., Crežescu, I. & Petrescu, S. An experimental study of indigo carmine removal from aqueous solution by electrocoagulation. Desalination 277 , 227–235 (2011).
Pirkarami, A. & Olya, M. E. Removal of dye from industrial wastewater with an emphasis on improving economic efficiency and degradation mechanism. J. Saudi Chem. Soc. 21 , S179–S186 (2017).
Bayramoglu, M., Eyvaz, M. & Kobya, M. Treatment of the textile wastewater by electrocoagulation: Economical evaluation. Chem. Eng. J. 128 , 155–161 (2007).
Syam Babu, D., Anantha Singh, T. S., Nidheesh, P. V. & Suresh Kumar, M. Industrial wastewater treatment by electrocoagulation process. Sep. Sci. Technol. (Phila.) 55 , 3195–3227 (2020). vol.
Comninellis, C. Electrocatalysis in the electrochemical conversion/combustion of organic pollutants for waste water treatment. Electrochim. Acta 39 , 1857–1862 (1994).
Anglada, Á., Urtiaga, A. & Ortiz, I. Contributions of electrochemical oxidation to waste-water treatment: fundamentals and review of applications. J. Chem. Technol. Biotechnol. 84 , 1747–1755 (2009).
Mantzavinos, D. & Psillakis, E. Enhancement of biodegradability of industrial wastewaters by chemical oxidation pre-treatment. J. Chem. Technol. Biotechnol. 79 , 431–454 (2004).
Polcaro, A. M. et al. Characterization of a stirred tank electrochemical cell for water disinfection processes. Electrochim. Acta 52 , 2595–2602 (2007).
Sáez, C., Cañizares, P., Llanos, J. & Rodrigo, M. A. The treatment of actual industrial wastewaters using electrochemical techniques. Electrocatalysis. 4 , 252–258 (2013).
Anglada, A., Ibañez, R., Urtiaga, A. & Ortiz, I. Electrochemical oxidation of saline industrial wastewaters using boron-doped diamond anodes. Catal. Today 151 , 178–184 (2010).
Rajkumar, D. & Palanivelu, K. Electrochemical treatment of industrial wastewater. J. Hazard. Mater. 113 , 123–129 (2004).
Cañizares, P., Paz, R., Sáez, C. & Rodrigo, M. A. Costs of the electrochemical oxidation of wastewaters: A comparison with ozonation and Fenton oxidation processes. J. Environ. Manag. 90 , 410–420 (2009).
Chadwick, S. S. Ullmann’s encyclopedia of industrial chemistry. Ref. Serv. Rev. 16 , 31–34 (1988).
Urtiaga, A., Gómez, P., Arruti, A. & Ortiz, I. Electrochemical removal of tetrahydrofuran from industrial wastewaters: anode selection and process scale-up. J. Chem. Technol. Biotechnol. 89 , 1243–1250 (2014).
Vijayaraghavan, K., Ramanujam, T. K. & Balasubramanian, N. In situ hypochlorous acid generation for treatment of tannery wastewaters. J. Environ. Eng. 124 , 887–891 (1998).
Szpyrkowicz, L., Naumczyk, J. & Zilio-Grandi, F. Electrochemical treatment of tannery wastewater using Ti Pt and Ti/Pt/Ir electrodes. Water Res. 29 , 517–524 (1995).
Vlyssides, A. G. & Israilides, C. J. Electrochemical oxidation of a textile dye and finishing wastewater using a Pt/Ti electrode. J. Environ. Sci. Heal. Part A 33 , 847–862 (1998).
Khorram, A. G. & Fallah, N. Comparison of electrocoagulation and photocatalytic process for treatment of industrial dyeing wastewater: Energy consumption analysis. Environ. Prog. Sustain. Energy 39 , 13288 (2020).
Mehrjouei, M., Müller, S. & Möller, D. Energy consumption of three different advanced oxidation methods for water treatment: a cost-effectiveness study. J. Clean. Prod. 65 , 178–183 (2014).
Hosny, A. Y. Separating oil from oil-water emulsions by electroflotation technique. Sep. Technol. 6 , 9–17 (1996).
Chen, W. & Horan, N. J. The treatment of a high strength pulp and paper mill effluent for wastewater re-use. Env. Technol. 19 , 173–182 (1998).
Nahui, F. N. B., Nascimento, M. R., Cavalcanti, E. B. & Vilar, E. O. Electroflotation of emulsified oil in industrial wastes evaluated with a full factorial design. Braz. J. Chem. Eng. 25 , 435–442 (2008).
Vaughan, R., Brian, E., Gar, W. & David, M. Pilot-scale investigation of chemical addition-dissolved air flotation for the treatment of an oily wastewater. Environ. Eng. Sci. 17 , 267–277 (2009).
Belkacem, M., Khodir, M. & Abdelkrim, S. Treatment characteristics of textile wastewater and removal of heavy metals using the electroflotation technique. Desalination 228 , 245–254 (2008).
de Oliveira da Mota, I., de Castro, J. A., de Góes Casqueira, R. & de Oliveira Junior, A. G. Study of electroflotation method for treatment of wastewater from washing soil contaminated by heavy metals. J. Mater. Res. Technol. 4 , 109–113 (2015).
Aoudj, S., Khelifa, A., Drouiche, N. & Hecini, M. Removal of fluoride and turbidity from semiconductor industry wastewater by combined coagulation and electroflotation. Desalin. Water Treat. 57 , 18398–18405 (2016).
Melchiors, M. S. et al. Treatment of wastewater from the dairy industry using electroflocculation and solid whey recovery. J. Environ. Manag. 182 , 574–580 (2016).
Ho, C. C. & Chan, C. Y. The application of lead dioxide-coated titanium anode in the electroflotation of palm oil mill effluent. Water Res 20 , 1523–1527 (1986).
Alexandrova, L., Nedialkova, T. & Nishkov, I. Electroflotation of metal ions in waste water. Int. J. Miner. Process. 41 , 285–294 (1994).
Mavrov, V., Erwe, T., Blöcher, C. & Chmiel, H. Desalination and the Environment: Fresh Water for allStudy of new integrated processes combining adsorption, membrane separation and flotation for heavy metal removal from wastewater. Desalination 157 , 97–104 (2003).
Anpo, M. & Takeuchi, M. The design and development of highly reactive titanium oxide photocatalysts operating under visible light irradiation. J. Catal. 216 , 505–516 (2003).
Zheng, X., Chen, D., Wang, Z. W., Lei, Y. & Cheng, R. Nano-TiO 2 membrane adsorption reactor (MAR) for virus removal in drinking water. Chem. Eng. J. 230 , 180–187 (2013).
Chen, X. & Mao, S. S. Titanium dioxide nanomaterials: synthesis, properties, modifications, and applications. Chem. Rev. 107 , 2891–2959 (2007).
Lin, H. et al. Size dependency of nanocrystalline TiO 2 on its optical property and photocatalytic reactivity exemplified by 2-chlorophenol. Appl. Catal. B-Environ. 68 , 1–11 (2006).
Wang, Y., He, Y., Lai, Q. & Fan, M. Review of the progress in preparing nano TiO 2 : an important environmental engineering material. J. Environ. Sci. (China) 26 , 2139–2177 (2014).
Gupta, S. M. & Tripathi, M. A review on the synthesis of TiO 2 nanoparticles by solution route. Cent. Eur. J. Chem. 10 , 279–294 (2012).
Byrappa, K. & Adschiri, T. Hydrothermal technology for nanotechnology. Prog. Cryst. Growth Charact. Mater. 53 , 117–166 (2007).
Nam, C. T., Yang, W.-D. & Duc, L. M. Solvothermal Synthesis of TiO 2 Photocatalysts in Ketone Solvents with Low Boiling Points. J. Nanomater. 2013 , 11 (2013).
Paulose, M. et al. Anodic growth of highly ordered TiO 2 nanotube arrays to 134 microm in length. J. Phys. Chem. B 110 , 16179–16184 (2006).
Zhao, L. & Yu, J. Controlled synthesis of highly dispersed TiO 2 nanoparticles using SBA-15 as hard template. J. Colloid Interface Sci. 304 , 84–91 (2006).
Chen, Y. et al. Synthesis of BiOI-TiO 2 composite nanoparticles by microemulsion method and study on their photocatalytic activities. Scientific World Journal. 2014 , 647040 (2014).
de Luna, L. A., da Silva, T. H., Nogueira, R. F., Kummrow, F. & Umbuzeiro, G. A. Aquatic toxicity of dyes before and after photo-Fenton treatment. J. Hazard Mater. 276 , 332–338 (2014).
Zeng, P. et al. Efficiency comparison for treatment of amantadine pharmaceutical wastewater by Fenton, ultrasonic, and Fenton/ultrasonic processes. Environ. Earth Sci. 73 , 4979–4987 (2015).
Liu, Z., Sun, D. D., Guo, P. & Leckie, J. O. One-step fabrication and high photocatalytic activity of porous TiO 2 hollow aggregates by using a low-temperature hydrothermal method without templates. Chem. (Easton) 13 , 1851–1855 (2007).
Kang, M. et al. Characterization of a TiO 2 photocatalyst synthesized by the solvothermal method and its catalytic performance for CHCl3 decomposition. J. Photochem. Photobiol. A Chem. 144 , 185–191 (2001).
Supphasrirongjaroen, P., Praserthdam, P., Panpranot, J., Na-Ranong, D. & Mekasuwandumrong, O. Effect of quenching medium on photocatalytic activity of nano-TiO 2 prepared by solvothermal method. Chem. Eng. J. 138 , 622–627 (2008).
Tan, Y. N., Wong, C. L. & Mohamed, A. R. An overview on the photocatalytic activity of nano-doped- in the degradation of organic pollutants. ISRN Mater. Sci. 2011 , 18 (2011).
Gupta, S. M. & Tripathi, M. A review of TiO 2 nanoparticles. Chin. Sci. Bull. 56 , 1639–1657 (2011).
Kuwahara, Y. & Yamashita, H. Efficient photocatalytic degradation of organics diluted in water and air using TiO 2 designed with zeolites and mesoporous silica materials. J. Mater. Chem. 21 , 2407–2416 (2011).
Chen, C.-C. & Lu, C.-S. Mechanistic studies of the photocatalytic degradation of methyl green: an investigation of products of the decomposition processes. Env. Sci. Technol. 41 , 4389–4396 (2007).
Mozia, S. Photocatalytic membrane reactors (PMRs) in water and wastewater treatment. A review. Sep. Purif. Technol. 73 , 71–91 (2010).
Giwa, A. et al. Recent advances in advanced oxidation processes for removal of contaminants from water: A comprehensive review. Process Saf. Environ. Prot. 146 , 220–256 (2021).
Yusuf, A. et al. A review of emerging trends in membrane science and technology for sustainable water treatment. J. Clean. Prod. 266 , 121867 (2020).
Giwa, A., Dindi, A. & Kujawa, J. Membrane bioreactors and electrochemical processes for treatment of wastewaters containing heavy metal ions, organics, micropollutants and dyes: Recent developments. J. Hazard. Mater. 370 , 172–195 (2019).
Lenzi, G. G. et al. Photocatalytic degradation of textile reactive dye using artificial neural network modeling approach. N. Pub Balaban 57 , 14132–14144 (2015).
Bararpour, S. T. et al. Investigation of 2-nitrophenol solar degradation in the simultaneous presence of K2S2O8 and H2O2: Using experimental design and artificial neural network. J. Clean. Prod. 176 , 1154–1162 (2018).
Khataee, A. R., Fathinia, M., Zarei, M., Izadkhah, B. & Joo, S. W. Modeling and optimization of photocatalytic/photoassisted-electro-Fenton like degradation of phenol using a neural network coupled with genetic algorithm. J. Ind. Eng. Chem. 20 , 1852–1860 (2014).
Lin, C. H., Yu, R. F., Cheng, W. P. & Liu, C. R. Monitoring and control of UV and UV-TiO 2 disinfections for municipal wastewater reclamation using artificial neural networks. J. Hazard. Mater. 209–210 , 348–354 (2012).
Hu, C. et al. Plasmon-induced photodegradation of toxic pollutants with Ag−AgI/Al2O3 under visible-light irradiation. J. Am. Chem. Soc. 132 , 857–862 (2009).
Jie, X., Bao, N., Gong, B. & Zhou, S. Facile synthesis of plasmonic Ag/AgCl/polydopamine-TiO 2 fibers for efficient visible photocatalysis. Nano-Struct. Nano-Objects 12 , 98–105 (2017).
Hu, X., Li, Y., Tian, J., Yang, H. & Cui, H. Highly efficient full solar spectrum (UV-vis-NIR) photocatalytic performance of Ag2S quantum dot/TiO 2 nanobelt heterostructures. J. Ind. Eng. Chem. 45 , 189–196 (2017).
Wang, T. et al. Enhanced performance of TiO 2 /reduced graphene oxide doped by rare-earth ions for degrading phenol in seawater excited by weak visible light. Adv. Powder Technol. 30 , 1920–1931 (2019).
Xiao, S., Shen, M., Guo, R., Wang, S. & Shi, X. Immobilization of zerovalent iron nanoparticles into electrospun polymer nanofibers: synthesis, characterization, and potential environmental applications. J. Phys. Chem. C. 113 , 18062–18068 (2009).
Katsou, E., Malamis, S. & Loizidou, M. Performance of a membrane bioreactor used for the treatment of wastewater contaminated with heavy metals. Bioresour. Technol. 102 , 4325–4332 (2011).
Huang, X., Shen, Y. & X, K. Recent advances in membrane bioreactor technology for wastewater treatment in China. Front. Environ. Sci. Eng. 4 , 245–271 (2010).
Ryu, J., Choi, W. & Choo, K. H. A pilot-scale photocatalyst-membrane hybrid reactor: Performance and characterization. Water Sci. Technol. 51 , 491–497 (2005).
Doruk, N., Yatmaz, H. C. & Dizge, N. Degradation efficiency of textile and wood processing industry wastewater by photocatalytic process using in situ ultrafiltration membrane. CLEAN – Soil Air Water 44 , 224–231 (2016).
Thiruvenkatachari, R., Kwon, T. O. & Moon, I. S. Application of slurry type photocatalytic oxidation-submerged hollow fiber microfiltration hybrid system for the degradation of bisphenol A (BPA). Sep. Sci. Technol. 40 , 2871–2888 (2005).
Fu, J., Ji, M., Zhao, Y. & Wang, L. Kinetics of aqueous photocatalytic oxidation of fulvic acids in a photocatalysis-ultrafiltration reactor (PUR). Sep. Purif. Technol. 50 , 107–113 (2006).
Le-Clech, P., Lee, E. K. & Chen, V. Hybrid photocatalysis/membrane treatment for surface waters containing low concentrations of natural organic matters. Water Res 40 , 323–330 (2006).
Sato, S. Photocatalysts sensitive to visible light. Sci. (80-.) 295 , 626–627 (2002).
Ihara, T., Miyoshi, M., Iriyama, Y., Matsumoto, O. & Sugihara, S. Visible-light-active titanium oxide photocatalyst realized by an oxygen-deficient structure and by nitrogen doping. Appl. Catal. B Environ. 42 , 403–409 (2003).
Nolan, M., Iwaszuk, A., Lucid, A. K., Carey, J. J. & Fronzi, M. Design of novel visible light active photocatalyst materials: surface modified TiO 2 . Adv. Mater. 28 , 5425–5446 (2016).
Deveci, E. Ü., Dizge, N., Yatmaz, H. C. & Aytepe, Y. Integrated process of fungal membrane bioreactor and photocatalytic membrane reactor for the treatment of industrial textile wastewater. Biochem. Eng. J. 105 , 420–427 (2016). Part .
Gogate, P. R. Hydrodynamic cavitation for food and water processing. Food Bioprocess Technol. 4 , 996–1011 (2011).
Jyoti, K. K. & Pandit, A. B. Water disinfection by acoustic and hydrodynamic cavitation. Biochem. Eng. J. 7 , 201–212 (2001).
Son, H.-S., Choi, S.-B., Khan, E. & Zoh, K.-D. Removal of 1,4-dioxane from water using sonication: Effect of adding oxidants on the degradation kinetics. Water Res 40 , 692–698 (2006).
Maezawa, A. et al. Treatment of dye wastewater by using photo-catalytic oxidation with sonication. Ultrason. - Sonochem. 14 , 615–620 (2007).
Gashchin, O. R. & Viten’ko, T. N. Features of disinfection kinetics of water containing Escherichia Coli in conditions of hydrodynamic cavitation. J. Water Chem. Technol. 30 , 322–327 (2008).
Mezule, L., Tsyfansky, S., Yakushevich, V. & Juhna, T. A simple technique for water disinfection with hydrodynamic cavitation: Effect on survival of Escherichia coli. Desalination 248 , 152–159 (2009).
Braeutigam, P., Wu, Z. ‐L., Stark, A. & Ondruschka, B. Degradation of BTEX in Aqueous Solution by Hydrodynamic Cavitation. Chem. Eng. Technol. 32 , 745–753 (2009).
Sponza, D. T. & Oztekin, R. Effect of sonication assisted by titanium dioxide and ferrous ions on polyaromatic hydrocarbons (PAHs) and toxicity removals from a petrochemical industry wastewater in Turkey. J. Chem. Technol. Biotechnol. 85 , 913–925 (2010).
Musmarra, D. et al. Degradation of ibuprofen by hydrodynamic cavitation: Reaction pathways and effect of operational parameters. Ultrason. Sonochem. 29 , 76–83 (2016).
Braeutigam, P. et al. Degradation of carbamazepine in environmentally relevant concentrations in water by Hydrodynamic-Acoustic-Cavitation (HAC). Water Res 46 , 2469–2477 (2012).
Oztekin, R. & Sponza, D. T. Treatment of wastewaters from the olive mill industry by sonication. J. Chem. Technol. Biotechnol. 88 , 212–225 (2013).
Bani-Melhem, K. & Elektorowicz, M. Performance of the submerged membrane electro-bioreactor (SMEBR) with iron electrodes for wastewater treatment and fouling reduction. J. Memb. Sci. 379 , 434–439 (2011).
Ensano, B. M. B. et al. Combination of electrochemical processes with membrane bioreactors for wastewater treatment and fouling control: a review. Front. Environ. Sci . 4 , (2016). https://doi.org/10.3389/fenvs.2016.00057 .
Ahmed, M. A. L. & Hasan, S. W. Fe and Zn removal from steel making industrial wastewater by electrically enhanced membrane bioreactor. Desalin. Water Treat . 6–8 (2017). https://doi.org/10.5004/dwt.2017.21305 .
Hagelüken, C., Lee-Shin, U. J., Carpentier, A. & Heron, C. The EU circular economy and its relevance to metal recycling. Recycling 1 , 242–253 (2016).
(IWA), T. international water association. Water Utility Pathways in a Circular Economy . (2016).
Puyol, D. et al. Resource recovery from wastewater by biological technologies: opportunities, challenges, and prospects. Front. Microbiol . 7 , 2106 (2017).
Wang, H. & Ren, Z. J. Bioelectrochemical metal recovery from wastewater: A review. Water Res 66 , 219–232 (2014).
Bae, T.-H., Han, S.-S. & Tak, T.-M. Membrane sequencing batch reactor system for the treatment of dairy industry wastewater. Process Biochem 39 , 221–231 (2003).
Lu, S. G. et al. The performance of fermentation wastewater treatment in ultrafiltration membrane bioreactor by contiuous and intermittent aeration processes. Water Sci. Technol. 42 , 323–329 (2000).
Tsilogeorgis, J., Zouboulis, A., Samaras, P. & Zamboulis, D. European Desalination Society and Center for Research and Technology Hellas (CERTH), Sani Resort 22 –25 April 2007, Halkidiki, GreeceApplication of a membrane sequencing batch reactor for landfill leachate treatment. Desalination 221 , 483–493 (2008).
Hall, E. R., Onysko, K. A. & Parker, W. J. Enhancement of bleached kraft organochlorine removal by coupling membrane filtration and anaerobic treatment. Env. Technol. 16 , 115–126 (1995).
Hai, F. I., Yamamoto, K. & Fukushi, K. International Congress on Membranes and Membrane ProcessesDevelopment of a submerged membrane fungi reactor for textile wastewater treatment. Desalination 192 , 315–322 (2006).
Yigit, N. O. et al. The Third Membrane Science and Technology Conference of Visegrad Countries (PERMEA); part 1Treatment of a denim producing textile industry wastewater using pilot-scale membrane bioreactor. Desalination 240 , 143–150 (2009).
Delgado, L. F. et al. Removal of a cytostatic drug by a membrane bioreactor. Desalin. Water Treat. 9 , 112–118 (2009).
Torres, A. P. R., Santiago, V. M. J. & Borges, C. P. Performance evaluation of submerged membrane bioreactor pilot units for refinery wastewater treatment. Environ. Prog. 27 , 189–194 (2008).
Qin, J.-J., Oo, M. H., Tao, G. & Kekre, K. A. Feasibility study on petrochemical wastewater treatment and reuse using submerged MBR. J. Memb. Sci. 293 , 161–166 (2007).
Goltara, A., Martinez, J. & Mendez, R. Carbon and nitrogen removal from tannery wastewater with a membrane bioreactor. Water Sci. Technol. 48 , 207–214 (2003).
Munz, G. et al. The role of tannins in conventional and membrane treatment of tannery wastewater. J. Hazard. Mater. 164 , 733–739 (2009).
Chegrouche, S., Mellah, A. & Barkat, M. Removal of strontium from aqueous solutions by adsorption onto activated carbon: kinetic and thermodynamic studies. Desalination 235 , 306–318 (2009).
Barkat, M., Nibou, D., Chegrouche, S. & Mellah, A. Kinetics and thermodynamics studies of chromium(VI) ions adsorption onto activated carbon from aqueous solutions. Chem. Eng. Process. Process. Intensif. 48 , 38–47 (2009).
Kannan, N. & Rengasamy, G. Comparison of Cadmium Ion Adsorption on Various ACTIVATED CARBONS. Water Air. Soil Pollut. 163 , 185–201 (2005).
Çetinkaya Dönmez, G., Aksu, Z., Öztürk, A. & Kutsal, T. A comparative study on heavy metal biosorption characteristics of some algae. Process Biochem 34 , 885–892 (1999).
Dakiky, M., Khamis, M., Manassra, A. & Mer’eb, M. Selective adsorption of chromium(VI) in industrial wastewater using low-cost abundantly available adsorbents. Adv. Environ. Res. 6 , 533–540 (2002).
Anjana, K., Kaushik, A., Kiran, B. & Nisha, R. Biosorption of Cr(VI) by immobilized biomass of two indigenous strains of cyanobacteria isolated from metal contaminated soil. J. Hazard. Mater. 148 , 383–386 (2007).
Srinivasa Rao, P., Kalyani, S., Suresh Reddy, K. V. N. & Krishnaiah, A. Comparison of biosorption of Nickel (II) and Copper (II) ions from aqueous solution by sphaeroplea algae and acid treated sphaeroplea algae. Sep. Sci. Technol. 40 , 3149–3165 (2005).
Green-Ruiz, C., Rodriguez-Tirado, V. & Gomez-Gil, B. Cadmium and zinc removal from aqueous solutions by Bacillus jeotgali: pH, salinity and temperature effects. Bioresour. Technol. 99 , 3864–3870 (2008).
Ucun, H., Bayhana, Y. K., Kaya, Y., Cakici, A. & Algur, O. F. Biosorption of lead (II) from aqueous solution by cone biomass of Pinus sylvestris. Desalination 154 , 233–238 (2003).
Ahluwalia, S. S. & Goyal, D. Microbial and plant derived biomass for removal of heavy metals from wastewater. Bioresour. Technol. 98 , 2243–2257 (2007).
Pérez, M., Torrades, F., Domènech, X. & Peral, J. Fenton and photo-Fenton oxidation of textile effluents. Water Res 36 , 2703–2710 (2002).
Sahinkaya, S., Ozdemir, C. & Karatas, M. USE OF FENTON’S REAGENT FOR REMOVAL OF PESTICIDES FROM INDUSTRIAL WASTEWATER. in (SGEM Scientific GeoConference, 2007). citeulike-article-id:13484962.
Peternel, I. T., Koprivanac, N., Božić, A. M. L. & Kušić, H. M. Comparative study of UV/TiO 2 , UV/ZnO and photo-Fenton processes for the organic reactive dye degradation in aqueous solution. J. Hazard. Mater. 148 , 477–484 (2007).
Rivas, F. J., Beltrán, F. J., Gimeno, O. & Alvarez, P. Optimisation of Fenton’s reagent usage as a pre-treatment for fermentation brines. J. Hazard. Mater. 96 , 277–290 (2003).
Sun, J.-H. et al. Oxidative decomposition of p-nitroaniline in water by solar photo-Fenton advanced oxidation process. J. Hazard. Mater. 153 , 187–193 (2008).
Kušić, H., Koprivanac, N., Božić, A. L. & Selanec, I. Photo-assisted Fenton type processes for the degradation of phenol: A kinetic study. J. Hazard. Mater. 136 , 632–644 (2006).
Tekin, H. et al. Use of Fenton oxidation to improve the biodegradability of a pharmaceutical wastewater. J. Hazard. Mater. 136 , 258–265 (2006).
Cui, X. et al. Pilot-scale treatment of pharmaceutical berberine wastewater by Fenton oxidation. Environ. Earth Sci. 73 , 4967–4977 (2015).
Pérez, M., Torrades, F., Garcı́a-Hortal, J. A., Domènech, X. & Peral, J. Removal of organic contaminants in paper pulp treatment effluents under Fenton and photo-Fenton conditions. Appl. Catal. B Environ. 36 , 63–74 (2002).
Catalkaya, E. C. & Color, F. K. TOC, and AOX removals from pulp and mill effluent by advanced oxidation processes: a comparative study. J. Hazard. Mater. 139 , 244–253 (2007).
Neyens, E. & Baeyens, J. A review of classic Fenton’s peroxidation as an advanced oxidation technique. J. Hazard. Mater. 98 , 33–50 (2003).
Kochany, J. & Lipczynska-Kochany, E. Utilization of landfill leachate parameters for pretreatment by Fenton reaction and struvite precipitation-A comparative study. J. Hazard. Mater. 166 , 248–254 (2009).
De la Cruz, N. et al. Degradation of 32 emergent contaminants by UV and neutral photo-fenton in domestic wastewater effluent previously treated by activated sludge. Water Res 46 , 1947–1957 (2012).
Li, J., Luan, Z., Yu, L. & Ji, Z. Pretreatment of acrylic fiber manufacturing wastewater by the Fenton process. Desalination 284 , 62–65 (2012).
Ma, X.-J. & Xia, H.-L. Treatment of water-based printing ink wastewater by Fenton process combined with coagulation. J. Hazard. Mater. 162 , 386–390 (2009).
Minella, M. et al. Photo-Fenton oxidation of phenol with magnetite as iron source. Appl. Catal. B Environ. 154–155 , 102–109 (2014).
Bagher Miranzadeh, M. et al. Comparison of Fenton and Photo-Fenton Processes for Removal of Linear Alkyle Benzene Sulfonate (Las) from Aqueous Solutions . vol. 25 (2016).
Iglesias, O., Gómez, J., Pazos, M. & Sanromán, M. Á. Electro-Fenton oxidation of imidacloprid by Fe alginate gel beads. Appl. Catal. B Environ. 144 , 416–424 (2014).
Kongjao, S., Damronglerd, S. & Hunsom, M. Simultaneous removal of organic and inorganic pollutants in tannery wastewater using electrocoagulation technique. Korean J. Chem. Eng. 25 , 703–709 (2008).
Wang, C.-T. & Chou, W.-L. Performance of COD removal from oxide chemical mechanical polishing wastewater using iron electrocoagulation. J. Environ. Sci. Heal. Part A 44 , 1289–1297 (2009).
Kumar, M., Ponselvan, F. I. A., Malviya, J. R., Srivastava, V. C. & Mall, I. D. Treatment of bio-digester effluent by electrocoagulation using iron electrodes. J. Hazard. Mater. 165 , 345–352 (2009).
Kushwaha, J. P., Srivastava, V. C. & Mall, I. D. Organics removal from dairy wastewater by electrochemical treatment and residue disposal. Sep. Purif. Technol. 76 , 198–205 (2010).
Valero, D. et al. Electrocoagulation of wastewater from almond industry. Chemosphere 84 , 1290–1295 (2011).
Akyol, A. Treatment of paint manufacturing wastewater by electrocoagulation. Desalination 285 , 91–99 (2012).
Chou, W. L., Wang, C. T. & Huang, K. Y. Investigation of process parameters for the removal of polyvinyl alcohol from aqueous solution by iron electrocoagulation. Desalination 251 , 12–19 (2010).
Heidmann, I. & Calmano, W. Removal of Ni, Cu and Cr from a galvanic wastewater in an electrocoagulation system with Fe- and Al-electrodes. Sep. Purif. Technol. 71 , 308–314 (2010).
Vasudevan, S., Lakshmi, J. & Sozhan, G. Effects of alternating and direct current in electrocoagulation process on the removal of cadmium from water. J. Hazard. Mater. 192 , 26–34 (2011).
Mansouri, K., Elsaid, K., Bedoui, A., Bensalah, N. & Abdel-Wahab, A. Application of electrochemically dissolved iron in the removal of tannic acid from water. Chem. Eng. J. 172 , 970–976 (2011).
Li, P., Song, Y. & Yu, S. Removal of Microcystis aeruginosa using hydrodynamic cavitation: performance and mechanisms. Water Res 62 , 241 (2014).
Nidheesh, P. V. & Gandhimathi, R. Trends in electro-Fenton process for water and wastewater treatment: An overview. Desalination 299 , 1 (2012).
Horovitz, I. et al. Carbamazepine degradation using a N-doped TiO 2 coated photocatalytic membrane reactor: Influence of physical parameters. J. Hazard. Mater. 310 , 98–107 (2016).
Download references
Acknowledgements
We would like to acknowledge the support, guidance, and assistance provided by the library services team at Khalifa University of Science and Technology, Abu Dhabi, United Arab Emirates. This review would not have been possible without the access to the different library database and e-sources. We would also like to acknowledge the funding from Khalifa University through the Center for Membranes and Advanced Water Technology (CMAT), under grant number RC2-2018-009.
Author information
Authors and affiliations.
Center for Membranes and Advanced Water Technology (CMAT), Department of Chemical Engineering, Khalifa University of Science and Technology, PO Box 127788, Abu Dhabi, UAE
Menatalla Ahmed, Musthafa O. Mavukkandy, Adewale Giwa & Shadi W. Hasan
Department of Building, Civil and Environmental Engineering, Concordia University, 1455 Blvd de Maisonneuve W., Montreal, QC, H3G 1M8, Canada
Maria Elektorowicz
Department of Civil and Environmental Engineering, Brunel University London, Uxbridge, UB8 3PH, UK
Evina Katsou
Norwegian University of Life Sciences (NMBU), PO Box 5003-IMT, 1432, Ås, Norway
Olfa Khelifi
Sanitary Environmental Engineering Division (SEED), Department of Civil Engineering, University of Salerno - Via Giovanni Paolo II #132, 84084, Fisciano (SA), Italy
Vincenzo Naddeo
You can also search for this author in PubMed Google Scholar
Contributions
M.A.: Conceptualization, Methodology, Writing—original draft. M.O.M.: Writing—review & editing. A.G.: Conceptualization, Methodology, Writing—original draft. M.E.: Review & editing. E.K.: Writing—review & editing. O.K.: Writing—review & editing. V.N.: Review & editing. S.W.H.: Conceptualization, Validation, Resources, Supervision, Review & editing, Administration.
Corresponding author
Correspondence to Shadi W. Hasan .
Ethics declarations
Competing interests.
The authors declare no competing interests.
Additional information
Publisher’s note Springer Nature remains neutral with regard to jurisdictional claims in published maps and institutional affiliations.
Rights and permissions
Open Access This article is licensed under a Creative Commons Attribution 4.0 International License, which permits use, sharing, adaptation, distribution and reproduction in any medium or format, as long as you give appropriate credit to the original author(s) and the source, provide a link to the Creative Commons license, and indicate if changes were made. The images or other third party material in this article are included in the article’s Creative Commons license, unless indicated otherwise in a credit line to the material. If material is not included in the article’s Creative Commons license and your intended use is not permitted by statutory regulation or exceeds the permitted use, you will need to obtain permission directly from the copyright holder. To view a copy of this license, visit http://creativecommons.org/licenses/by/4.0/ .
Reprints and permissions
About this article
Cite this article.
Ahmed, M., Mavukkandy, M.O., Giwa, A. et al. Recent developments in hazardous pollutants removal from wastewater and water reuse within a circular economy. npj Clean Water 5 , 12 (2022). https://doi.org/10.1038/s41545-022-00154-5
Download citation
Received : 18 July 2021
Accepted : 23 February 2022
Published : 12 April 2022
DOI : https://doi.org/10.1038/s41545-022-00154-5
Share this article
Anyone you share the following link with will be able to read this content:
Sorry, a shareable link is not currently available for this article.
Provided by the Springer Nature SharedIt content-sharing initiative
This article is cited by
Assessment of toxicity and antimicrobial performance of polymeric inorganic coagulant and evaluation for eutrophication reduction.
- Marwa Youssef
- Sara S. El-Tanany
- Mohamed Eid M. Ali
Scientific Reports (2024)
Construction of flake ball-shaped Bi2WO6 embedded on phenyl functionalized g-C3N4 nanosheet for efficient degradation insight of colorless pollutants and its biological application
- Ziyaur Rasool
- Mohammad Saud Athar
- Mohammad Muneer
Environmental Science and Pollution Research (2024)
WO3–NiO/rGO Based Photocatalyst for Effectively Degradation of Colored and Colorless Pollutants Using Solar Light Irradiation
- Beriham Basha
- Imran Shakir
- Muhammad Farooq Warsi
Journal of Inorganic and Organometallic Polymers and Materials (2024)
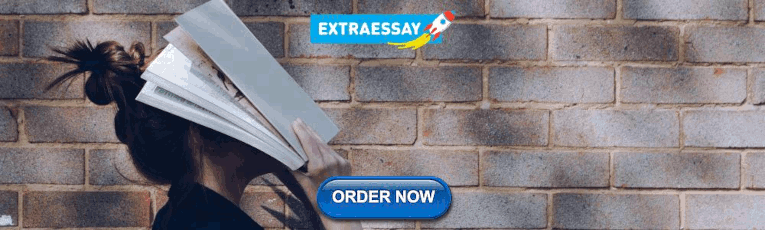
Experimental and Theoretical Investigation of the Effect of Cellulose Nanowhiskers on the Pb(II) Adsorption by Superabsorbent Hydrogel Nanocomposites
- André R. Fajardo
- Robson S. Oliboni
- Francisco H. A. Rodrigues
Water, Air, & Soil Pollution (2024)
Visible light-induced H2 production and pollutant degradation by copper oxide nanosphere embedded zinc-cadmium-sulfide composite
- Muhammad Imran
- Ammar Bin Yousaf
- Peter Kasak
Emergent Materials (2024)
Quick links
- Explore articles by subject
- Guide to authors
- Editorial policies
Sign up for the Nature Briefing: Microbiology newsletter — what matters in microbiology research, free to your inbox weekly.

EDITORIAL article
Editorial: recent trends in integrated wastewater treatment for sustainable development.
- 1 Waste Re-processing Division, CSIR-National Environmental Engineering Research Institute (CSIR-NEERI), Nagpur, India
- 2 School of Life Science and Food Engineering, Huaiyin Institute of Technology, Huaian, China
- 3 Waste and Effluent Treatment Laboratory, Institute of Technology and Research (ITP), Tiradentes University, Aracaju, Brazil
- 4 Graduate Program in Process Engineering, Tiradentes University, Aracaju, Brazil
Editorial on the Research Topic Recent Trends in Integrated Wastewater Treatment for Sustainable Development
Water, one of the utmost valuable natural resources for life on Earth, is critical to our society's economic development and success (agriculture, industry, and energy production). Nothing is more important to life on Earth than water. Although water is abundant on Earth, covering around 70% of the surface area, only 1% of that resource is available for human consumption. Water usage is significantly increasing in many parts of the world as a result of rising population, increasing economic activities, widespread industrialization, and urbanization, which are all raising living standards and boosting food demand. Thus, the problem of freshwater scarcity and contamination of natural water resources has become a menace to the developing globe that requires rapid response ( Yaashikaa et al., 2022 ). Worldwide the freshwater use is projected to have increased twice as quickly as the human population. In 2050, consumption is predicted to be tremendously higher than it is today, with over 40% of the world's population facing water scarcity issues. These factors compel us to consider non-conventional water sources as a means of meeting the growing need for fresh water. Wastewater and waste treatment are feasible options for dealing with freshwater scarcity. Various industries, municipalities, agricultural lands, commercial regions, and urban areas all emit large amounts of highly contaminated wastewater ( Nookwam et al., 2022 ). According to the World Health Organization (WHO), providing good quality water for sustainable development of the society, wastewater treatment is essential before its discharge into surface water systems to protect the environment and public health.
According to Lamastra et al. (2016) , more than 700 different metabolites and emerging pollutants are discharged into the environment without treatment. This fact is further worse by the lack of knowledge about the transportation, destination, and toxic potential of these toxic contaminants when they entered into the ecosystem, besides hampering the development of environmental policies controlled by regulatory agencies that promote the sustainable treatment and management of pollutants in the open environment ( Taheran et al., 2018 ). However, the persistent increase of these in the contaminated site still present due to the consumerist profile of the current society ( Vasilachi et al., 2021 ). In this context, biocatalysis plays a vital role in the growth of many industries, such as food, energy, and fine chemistry, since this green alternative allows replacing traditional processes through the use of microorganisms and their enzymes in catalytic reactions that can be applied in the biodegradation of various organic and emerging contaminants present in various agro-industrial and industrial effluents, reducing environmental concerns and improving the water quality after the use of microbiotecnologies.
Physicochemical approaches such as membrane filtration, ion exchange, advanced oxidation processes, and coagulation/flocculation, despite their efficacy, are not preferred due to sensitivity to variable water input, excessive chemical consumption, high operational costs, and the generation of a large amount of sludge as well as secondary pollutants. Although traditional wastewater treatment technologies are widely used, they fail to eradicate numerous complex contaminants. These chemicals eventually make their way into wastewater, posing a threat to water quality ( Fito and Van Hulle, 2021 ; Daud et al., 2022 ). Even after secondary treatment, wastewater is expected to produce substantial volumes of new and complex contaminants that are always more difficult to deal with. Adequate wastewater treatment is rarely achieved by the use of a single treatment technique.
There is an urgent need to develop a cost-effective, efficient, and comprehensive technologies or improve the existing methods through some interventions for adequate treatment and eliminating pollutants from wastewater to achieve environmental sustainability ( Varjani et al., 2020 ; Shah et al., 2022 ). In the recent past, innovative integrated treatment technologies by coupling two or more biological, physical, and chemical processes to remediate or clean up many environmental contaminants from wastewater have been gained worldwide attention ( Figure 1 ). Overall, this Research Topic (RT) provided an appropriate platform for discussing current trends, developments, and applications of integrated treatment technologies in the degradation and detoxification of wastewater or pollutants, including new emerging contaminants, industrial discharges, and municipal wastewater treatment plants.
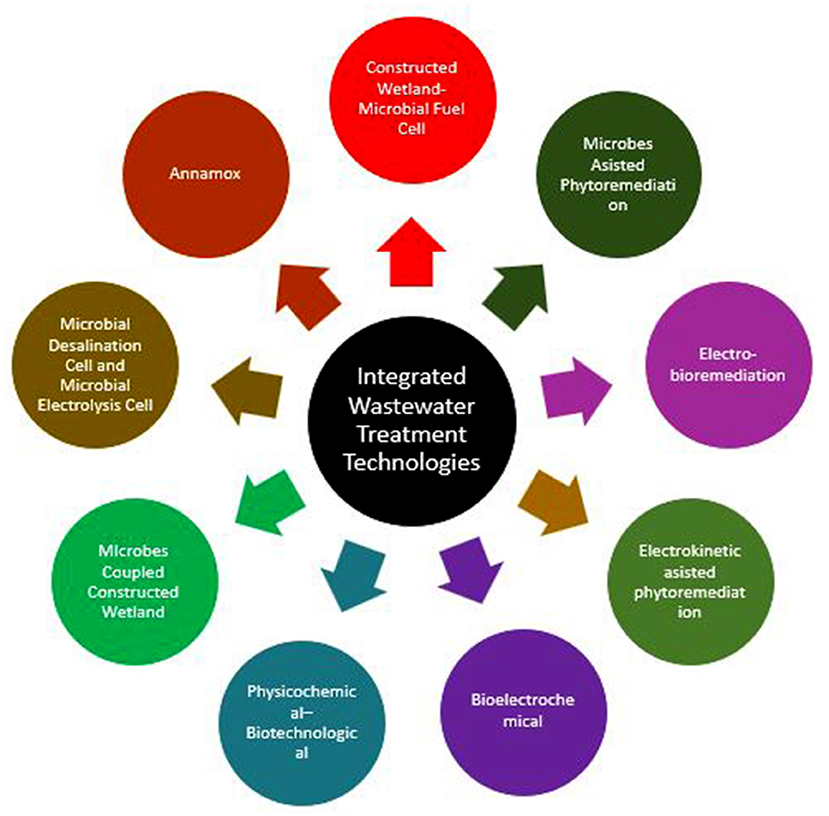
Figure 1 . Various integrated environmental technology used for wastewater treatment and management.
This RT consists of three research articles and four reviews contributed by leading experts from around the globe. The first research article, which was contributed by Verdel et al. and the team, has focused on the development of a biobased approach for the remediation of white water utilizing an indigenous bacteria isolate. Authors developed a bacterial consortium comprises Aeromonas sp. RES19-BTP, Cellulosimicrobium sp. AKD4- BF, Sphingomonas sp. BLA14-CF, and Xanthomonadales sp. CST37-CF for degradation of organic additives. The consortium immobilized on carriers showed a reduction of COD up to 88% in the white-water in a pilot-scale 33-liter tubular reactor filled with white water even after 21 days. Marathe et al. investigated the phytotreatment efficiency of low-cost organic raw materials for the remediation of saline effluent generated by tannery, textile, and pulp-paper industries. According to the Food and Agriculture Organization (FAO), wastewater having high total dissolved solids (TDS; 2,000 mg/L) is laid under severe restriction use for irrigation due to high salt accumulation in crop roots resulting in a total loss in crop yield. Authors used saline wastewater had high TDS (6,143.33 mg/L) for its treatment using lysimeters aided with organic filter bedding raw material as planted with potential plant, Eucalyptus camaldulensis . E. camaldulensis plant species displayed high efficiency to treat wastewater simultaneous accumulation of the high amount of sodium, calcium, magnesium, potassium, and produce higher biomass, and chlorophyll content without showing any negative impact on the growth as a function of wastewater. The high TDS management efficiency of coconut and rice husk as bedding material in a combination with forestry plant species was highest compared to other tested organic raw materials. The authors concluded that organic waste based amendments are an eco-sustainable option for managing saline wastewater.
Mishra et al. reviewed bacterial-assisted degradation of toxic dyes used in textile, cosmetics, and pharmaceuticals industries and other co-contaminants that pose a significant threat to humans and the ecosystem. Thus, public demands for decontaminated effluent to the receiving environment have made degradation, decolorization, and detoxification of contaminated wastewater discharges from industries a top priority. The authors highlight the decolorization and biodegradation mechanisms of various azo dyes by several bacterial enzymes. In addition, molecular docking of bacterial enzymes with dye molecules gives a new insight at the molecular level to explore the detailed mechanism of bacterial enzymes in dye degradation. The authors also discussed the molecular and physiological capabilities of isolates, which were beneficial for bioremediation processes.
Mathew et al. summarized the integrated algae and bacterial approaches for the degradation of contaminated sewage water. Due to the presence of an overwhelming blend of numerous refractory chemicals, the conventional approaches are unsuitable neither as on-site nor as a centralized treatment, and the urgent need of specific treatments is required. The integrated use of microbes for biodegradation is convenient because it is versatile, has dynamic metabolisms. The direct application of algae also helps to remove toxic heavy metals like aluminum, nickel, copper, etc. with simultaneous reduction of nutrients from the wastewater. Their growth also creates the potential for biofuels and bioproducts from their biomass. The augmentation of potent bacterial islates further enhanced the remediation efficacy of the wastewater. This happens as the inorganic nutrients was assimilated into the algal biomass whereas bacteria utilized organic nutrients. Furthermore, the mutualistic exchange of carbon dioxide and oxygen between bacterial and algae species helps intensify the photosynthetic activity of algae while oxidation-reduction by bacteria lead to the removal of nutrients from wastewater.
In general, one of the key motives of research organizations around the world is to develop technological improvements directed at the production chain of sustainable processes. Beyond the current topic's scientific, technological, and environmental importance, it is critical to develop integrated treatment methods for long-term development, using alternate reuse techniques concurrently to treat these residues and other resistant harmful chemicals. The latest recent innovations in integrated wastewater treatment methods provide an option to deal with organic and developing pollutants and increase process efficiency, making biotech and associated businesses more appealing because they use higher added value renewable inputs. At the same time, it enables creative methods to treat these residues and other resistant aromatic compounds via biological treatment systems, with the possibility of linking to new processes, and the practicality of deployment in biorefineries and meet the circular bioeconomy.
In summary, the new knowledge provided by the articles in this RT will expand our understanding of the current development in wastewater treatment by emerging integrated approaches and enable us to reach eco-sustainable treatment technology to obtain safe wastewater.
Author Contributions
VK drafted this editorial, which all authors reviewed and commented on, and eventually approved the final version of the manuscript for publication.
Conflict of Interest
The authors declare that the research was conducted in the absence of any commercial or financial relationships that could be construed as a potential conflict of interest.
Publisher's Note
All claims expressed in this article are solely those of the authors and do not necessarily represent those of their affiliated organizations, or those of the publisher, the editors and the reviewers. Any product that may be evaluated in this article, or claim that may be made by its manufacturer, is not guaranteed or endorsed by the publisher.
Acknowledgments
We wish to acknowledge the Frontiers Editorial Office for their assistance in completing this RT. We are also grateful to all authors for their outstanding contributions to this RT with readers and the scientific community. We would like to thank the reviewers for assuring the quality of the papers in this RT.
Daud, N. M., Abdullah, S. R. S., Hasan, H. A., and Dhokikah, Y. (2022). Integrated physical-biological treatment system for batik industry wastewater: a review on process selection. Sci. Total Environ . 819, 152931. doi: 10.1016/j.scitotenv.2022.152931
PubMed Abstract | CrossRef Full Text | Google Scholar
Fito, J., and Van Hulle, S. W. H. (2021). Wastewater reclamation and reuse potentials in agriculture: towards environmental sustainability. Environ. Dev. Sustain . 23, 2949–2972. doi: 10.1007/s10668-020-00732-y
CrossRef Full Text | Google Scholar
Lamastra, L., Balderacchi, M., and Trevisan, M. (2016). Inclusion of emerging organic contaminants in groundwater monitoring plans. Methods X . 3, 459-476. doi: 10.1016/j.mex.2016.05.008
Nookwam, K., Cheirsilp, B., Maneechote, W., Boonsawang, P., and Sukkasem, C. (2022). Microbial fuel cells with Photosynthetic-Cathodic chamber in vertical cascade for integrated bioelectricity, biodiesel feedstock production and wastewater treatment. Bioresour. Technol . 346, 126559. doi: 10.1016/j.biortech.2021.126559
Shah, A. V., Singh, A., Mohanty, S. S., Srivastava, V. K., and Varjani, S. (2022). Organic solid waste: Biorefinery approach as a sustainable strategy in circular bioeconomy. Bioresour. Technol . 349, 126835. doi: 10.1016/j.biortech.2022.126835
Taheran, M., Naghdi, M., Brar, S. K., Verma, M., and Surampalli, R. Y. (2018). Emerging contaminants: here today, there tomorrow!. Environ. Nanotechnol. Monit. Manag . 10, 122–126. doi: 10.1016/j.enmm.2018.05.010
Varjani, S., Pandey, A., and Upasani, V. N. (2020). Oilfield waste treatment using novel hydrocarbon utilizing bacterial consortium—a microcosm approach. Sci. Total Environ . 745, 141043. doi: 10.1016/j.scitotenv.2020.141043
Vasilachi, I. C., Asiminicesei, D. M., Fertu, D. I., and Gavrilescu, M. (2021). Occurrence and fate of emerging pollutants in water environment and options for their removal. Water 13, 181. doi: 10.3390/w13020181
Yaashikaa, P. R., Kumar, P. S., and Varjani, S. (2022). Valorization of agro-industrial wastes for biorefinery process and circular bioeconomy: a critical review. Bioresour. Technol . 343, 126126. doi: 10.1016/j.biortech.2021.126126
Keywords: phytotreatment, refractory contaminants, tertiary treatment, bioaugmentation, pulp-paper industry
Citation: Kumar V, Bilal M and Ferreira LFR (2022) Editorial: Recent Trends in Integrated Wastewater Treatment for Sustainable Development. Front. Microbiol. 13:846503. doi: 10.3389/fmicb.2022.846503
Received: 31 December 2021; Accepted: 11 March 2022; Published: 31 March 2022.
Reviewed by:
Copyright © 2022 Kumar, Bilal and Ferreira. This is an open-access article distributed under the terms of the Creative Commons Attribution License (CC BY) . The use, distribution or reproduction in other forums is permitted, provided the original author(s) and the copyright owner(s) are credited and that the original publication in this journal is cited, in accordance with accepted academic practice. No use, distribution or reproduction is permitted which does not comply with these terms.
*Correspondence: Vineet Kumar, drvineet.micro@gmail.com
Disclaimer: All claims expressed in this article are solely those of the authors and do not necessarily represent those of their affiliated organizations, or those of the publisher, the editors and the reviewers. Any product that may be evaluated in this article or claim that may be made by its manufacturer is not guaranteed or endorsed by the publisher.
Open Access is an initiative that aims to make scientific research freely available to all. To date our community has made over 100 million downloads. It’s based on principles of collaboration, unobstructed discovery, and, most importantly, scientific progression. As PhD students, we found it difficult to access the research we needed, so we decided to create a new Open Access publisher that levels the playing field for scientists across the world. How? By making research easy to access, and puts the academic needs of the researchers before the business interests of publishers.
We are a community of more than 103,000 authors and editors from 3,291 institutions spanning 160 countries, including Nobel Prize winners and some of the world’s most-cited researchers. Publishing on IntechOpen allows authors to earn citations and find new collaborators, meaning more people see your work not only from your own field of study, but from other related fields too.
Brief introduction to this section that descibes Open Access especially from an IntechOpen perspective
Want to get in touch? Contact our London head office or media team here
Our team is growing all the time, so we’re always on the lookout for smart people who want to help us reshape the world of scientific publishing.
Home > Books > Promising Techniques for Wastewater Treatment and Water Quality Assessment
Emerging Trends in Wastewater Treatment Technologies: The Current Perspective
Submitted: 19 August 2020 Reviewed: 04 September 2020 Published: 04 December 2020
DOI: 10.5772/intechopen.93898
Cite this chapter
There are two ways to cite this chapter:
From the Edited Volume
Promising Techniques for Wastewater Treatment and Water Quality Assessment
Edited by Iqbal Ahmed Moujdin and J. Kevin Summers
To purchase hard copies of this book, please contact the representative in India: CBS Publishers & Distributors Pvt. Ltd. www.cbspd.com | [email protected]
Chapter metrics overview
1,725 Chapter Downloads
Impact of this chapter
Total Chapter Downloads on intechopen.com

Total Chapter Views on intechopen.com
The quality of freshwater and its supply, particularly for domestic and industrial purposes are waning due to urbanization and inefficient conventional wastewater treatment (WWT) processes. For decades, conventional WWT processes have succeeded to some extent in treating effluents to meet standard discharge requirements. However, improvements in WWT are necessary to render treated wastewater for re-use in the industrial, agricultural, and domestic sectors. Three emerging technologies including membrane technology, microbial fuel cells and microalgae, as well as WWT strategies are discussed in this chapter. These applications are a promising alternative for manifold WWT processes and distribution systems in mitigating contaminants to meet acceptable limitations. The basic principles, types and applications, merits, and demerits of the aforementioned technologies are addressed in relation to their current limitations and future research needs. The development in WWT blueprints will augment the application of these emerging technologies for sustainable management and water conservation, with re-use strategies.
- contaminants
- membrane technology
- microbial fuel cell
- wastewater treatment
Author Information
Edward kwaku armah *.
- Department of Chemical Engineering, Durban University of Technology, South Africa
Maggie Chetty
Jeremiah adebisi adedeji, donald tyoker kukwa, boldwin mutsvene, khaya pearlman shabangu.
- Department of Chemical Engineering, Mangosuthu University of Technology, South Africa
Babatunde Femi Bakare
*Address all correspondence to: [email protected]
1. Introduction
The modern-day world has seen a boom in industrial activities. Due to extensive manufacturing activities taking place, large volumes of waste are produced, including wastewaters which are of major interest for re-use due to the scarcity of potable water in most countries. The wastewater produced poses serious environmental problems in its disposal. Because of new products that are emerging and being manufactured, so are new and recalcitrant wastes produced in production lines. Convectional wastewater technologies may be limited to process these contaminants, further exacerbating the problems the world is already facing with respect to potable water. Hence, there is a dire need to develop new methods to mitigate wastewater’s effect on the already degrading environment. On the other hand, clean, fresh potable water has become scarce especially in most African countries due to contamination by intensive industrial activities. To date over one hundred technologies for the treatment of organic and inorganic wastewater streams have been documented; several of these technologies have been emerging and these range from chemical and physical to biological methods. This book chapter focuses on the emerging trends of wastewater treatment technologies, with respect to membrane and biological methods.
Exhibiting high levels of novelty in purification technologies, membranes have been widely used and serve a crucial role in various fields, such as fatty and oily industrial water treatment [ 1 , 2 , 3 ].
Microalgae-based technologies are autotrophic in nature and microalgae is a highly potential atmospheric carbon fixation technology. After upstream treatment processes, microalgae technology is usually employed as secondary or tertiary treatment process for effluents that are laden with inorganic components such as nitrogen and phosphorus which cause eutrophication and more long term challenges that are caused by organic material and heavy metals in disposed of wastewater. Microalgal processes then chip in to offer at attractive dimension for the treatment of wastewater coupled with the generation of possibly biomass of high value which can further used for various purposes. Microalgae has minimal risk of production of secondary pollution because of its ability to use inorganic nitrogen and phosphorus for their growth; and their ability to remove heavy metals and toxic organics [ 4 , 5 , 6 ].
Another powerful, emerging treatment methodology is the Microbial fuel cells (MFCs) technology which capitalizes on the bioelectrical catalytic activity of microorganisms to generate electric power by oxidizing the organic matter and sometimes inorganic material in wastewater. MFC technology offers a dual goal as it allows for energy recovery and wastewater treatment in a single configuration [ 7 , 8 ].
2. Wastewater contaminants
The term wastewater is said to be water containing contaminants mainly due to human use. It emanates from diverse sources such as domestic, commercial, agricultural, or infiltration and storm run-off, with most wastewater being 99.9% water and the rest solids [ 9 ]. The characteristics of wastewater are usually determined by the chemical components and flow conditions, as this is used in the design of each wastewater treatment plant [ 10 ]. The flow conditions of wastewater are based on the seasons and it is mainly the wet season which will result in an inflow of storm run-offs. The organic and inorganic constituents of wastewater are used as an indicator of the chemical quality of wastewater. The following parameters are usually considered when measuring the chemical characteristics of wastewater; biochemical oxygen demand (BOD), chemical oxygen demand (COD), total solids (TS), volatile solids (VS), total nitrogen (TN), total phosphorus (TP), pH and alkalinity [ 11 ]; among others.
2.1 Chemical oxygen demand (COD)
This is usually a representative of the contaminants in wastewater as the higher the COD content in wastewater, the higher the degree of contamination. The COD content in industrial wastewater is usually higher when compared to that of domestic/municipal wastewater as presented in Table 1 . It gives an indication of the degree of biodegradation in wastewater when compared with BOD as the ratio of BOD to COD higher than 0.5 makes the wastewater biologically treatable [ 16 ]. It is measured as the quantity of oxygen required to stabilize the carbonaceous organic matter chemically. It is used to quantify the organic matter, nitrite, sulphide and ferrous salts present in wastewater [ 17 ].
Characteristics of raw industrial wastewater [ 12 , 13 , 14 , 15 ].
COD in wastewater could either be readily biodegradable matter, active autotrophic and heterotrophic biomass, soluble inert organic matter, inert inorganic matter [ 18 ]. Generally, the COD content in wastewater is either soluble or particulate (suspended). Classification of domestic wastewater based on COD include low (300-500 mg/L), medium (500-750 mg/L) and high (700 – 1200 mg/L) strength wastewater [ 19 ]. According to Henze and Comeau [ 19 ], the degradable COD content of a typical medium strength is 90% for soluble COD, 66% for particulate COD and 76% for total COD while the remaining percent are the inert component. The use of membrane technology only is very effective for low-strength wastewater [ 20 ] but the efficiency can be increased when combined with other technologies for treatment of high strength wastewater such as seen in the study by Matheus et al. [ 21 ] where microfiltration and nanofiltration was preceded by coagulation and flocculation to achieve a 96% COD removal (from 4610 mg/L to 184 mg/L) for dairy wastewater. Wastewater with high COD content usually causes fouling for the membrane [ 21 ], therefore, the use of biological treatment techniques such as microalgae and microbial fuel cell are more appropriate for high strength wastewater [ 22 , 23 ].
2.2 Biochemical oxygen demand (BOD)
This is the quantity of oxygen required by microorganisms for the decomposition of organic matter under aerobic conditions. As stated for COD, BOD is also an indication of the degree of contamination, it affects the amount of dissolved oxygen required by aquatic organisms, and if lower than 6 mg/L could lead to their death. The typical BOD value of domestic wastewater with minor industrial wastewater in it ranges from 100 – 200 mg/L, 200 – 300 mg/L and 300 – 560 mg/L for low, medium and high strength wastewater [ 19 ]. The relationship between BOD and dissolved oxygen is inversely proportional, as a low dissolved oxygen indicates a high BOD content in wastewater [ 24 ]. However, as the organic biodegradable content of water increases, the BOD increases also [ 25 ]. Since increase in biodegradable organic pollutants is an increase in the BOD, therefore, most biological treatment processes such as microalgae or microbial fuel cell technique can remove the BOD content in wastewater. Zhang et al. [ 26 ], indicated a 98.6% BOD removal using MFC while Marassi et al. [ 27 ] reported a 96-97% efficiency using a tubular MFC. The use of microalgae has also been reported to have effectively reduce the BOD content of wastewater by generation of O 2 during photosynthesis [ 28 ] and 87% removal efficiency [ 29 ].
2.3 Total solid (TS)
This is the organic and inorganic matter; suspended and dissolved solids; settleable and volatile solid content of wastewater. Though physical separation techniques easily remove most suspended solids, some still find their way into the environment. The dissolved and volatile solid (VS) contents are a representative of the degradable content in wastewater; therefore, some treatment techniques do account for the number of volatile solids removed. The VS content of wastewater, likewise, indicate its strength as higher VS indicate high strength wastewater and vice versa. The more the VS content of wastewater, the greater the impact on the treatment plant as it is an indication of the organic solid content. Total dissolved solids (TDS) are composed of inorganic salts and small quantities of organic matter dissolved in water. TDS in wastewater increases due to chemicals either from washing, cleaning, and production processes [ 30 ].
2.4 Total nitrogen and phosphorus
These are plant nutrients that are present in wastewater as either nitrates or ammonia, and fertilizer manufacturing companies usually generate them, agricultural sectors and industries that utilize corrosion inhibitors. Total nitrogen is the combination of both the inorganic and organic nitrogen, and ammonia in wastewater, it exists as either nitrate, nitrite, ammonium, and organic dissolved compounds such amino acids, urea, and organic nitrogen composites. In aquatic ecosystems, phosphorus is also present as phosphates such as orthophosphates, condensed phosphates and phosphates organically bound [ 25 ].
Nitrogen and phosphorus in wastewater cause eutrophication in water bodies which can lead to the death of aquatic habitats, if discharged without treatment [ 31 ]. High removal rate of nitrogen and phosphorus have been achieved using microalgae treatment process with industrial application of this technique been reported to achieve between 87 and 93% removal [ 32 ].
Metals are generally found in wastewater, mainly from the manufacturing, mining, and textile industries. Metals such as arsenic, iron, chromium, lead, copper, tin, sodium, potassium, mercury, aluminum, and nickel are common pollutants in industrial wastewaters [ 33 ]. Industries such as iron and steel, mining, micro-electronics, and textiles often generate wastewater with heavy metals therein. Metals in wastewater lead to an increase in the treatment costs, and they are known to cause varying environmental problems such as distortion in plant growth, algal bloom, death of aquatic biota, debris formation and sedimentation [ 34 ]. Human related health effects include carcinogenicity, chronic asthma, skin related problems, depression, internal organ damage, coughing and nervous system-related diseases [ 35 ].
The presence of metal in wastewater in low concentration (1-3 mg/L) is toxic because metals are non-biodegradable and some metals do accumulate overtime [ 33 , 36 ]. Although some metals which are essential to human, animal and plants may still be tolerated in minimal quantities such as copper, zinc, chromium but above the limit required can be toxic. An example is the reproduction of water flea Daphnia affected by exposure to 0.01 mg hexavalent chromium/L, therefore, the lethal chromium level for several aquatic and terrestrial invertebrates has been reported to be 0.05 mg/L. Some elements, however, such as arsenic, lead, cadmium, mercury is known to be toxic to living beings at any concentration and are not required to be taken into the body even at ultra-trace level [ 33 ].
2.6 Viruses and bacteria
The occurrence of human pathogenic viruses in wastewater is a usual occurrence, and newly discovered cases that were not associated with wastewater previously, are now considered as wastewater pollutants. Viral and bacterial infections from waterborne outbreaks are usually connected with environments associated with the discharge of wastewater [ 37 , 38 ]. Enteric viruses are known to cause gastroenteritis infections, hepatitis, and respiratory tract infections. Enteric viruses such as noroviruses, rotaviruses, enteroviruses, sapoviruses, astroviruses, bocaviruses, hepatitis A virus, hepatitis E virus, Aichi virus, Human polyomaviruses (PyVs), papillomaviruses, a plant virus called pepper mild mottle virus (PMMoV), and enteric bacteria such as bacteriophages, fecal coliforms and Escherichia coli are found in wastewater, and the full details of their occurrence and concentration in untreated and treated wastewater by continents can be seen in a review by Farkas et al., [ 37 ]. The emergence of severe acute respiratory syndrome coronavirus 2 (SARS-CoV-2), the virus related to the COVID-19 pandemic has been discovered in wastewater with entry through human feaces into sewer systems as other viruses [ 39 , 40 ]. Research is on-going on the effect of SARS-CoV-2 on aquatic habitats and its resulting long-term effect.
2.7 Pharmaceutical compounds
These compounds are part of the emerging pollutants in wastewater since their long-term effect on human and aquatic habitats are unknown. Compounds such as analgesics, antibiotics, anticonvulsants, anti-cancer agents, beta-blockers, contrast agents, hormones, lipid-regulators and antidepressants are pharmaceutical compounds that have recently been found in wastewater [ 41 ]. This is because human drugs are excreted either in original or metabolized form after administration. Though most pharmaceutical compounds are biologically degradable, but some product is seen in the effluent of wastewater treatment plant [ 41 ]. In effluents from a sewage treatment plants about 2 μg/L of tetracycline, ibuprofen, contrast products, caffeine, and codeine were found [ 42 ]. Likewise, Clara et al. [ 43 ] reported the presence of antibiotics (such as metronidazole, norfloxacin, and dextromethorphan (DMP)) at concentrations below 0.05 μg/L in another effluent. Studies indicate that the removal rate of antibiotic is around 50% and Bisphenol A 71%, that of analgesics, anti-inflammatory drugs, and beta-blockers is within 30–40% because of their resistant to treatment [ 41 ].
3. Emerging trends in wastewater treatment technologies
One of the primary reasons that has driven the inception of new or improved wastewater treatment technologies is the legislation and hefty fines that are attracted when the disposal of wastewater does not meet the set discharge limits. This impact on the financial wellbeing of factories and industries has fueled the emergence of new or improved treatment technologies.
Anaerobic and aerobic technologies have been popular lately in the treatment of organic wastewater because of their friendliness to the environment and cost-effectiveness. Anaerobic technologies are, however, a cut above other technologies because of the low energy consumption.
The nature of the wastewater primarily dictates the choice of technology to be adopted, and thus it is crucial to characterize streams to determine key wastewater characteristics, such as COD, TS, VS, and salt content, among others. The main thrust of this chapter is premised on three emerging technologies, that is, membrane, microalgal, and microbial fuel cell (MFC) technologies. These technologies can be employed independently or in series as a treatment mechanism.
3.1 Membrane technology
Membrane technology (MT) encompasses the related engineering and scientific approaches for the transport of components, species, or substances through or by membranes [ 44 ]. This technique is generally adopted to explain the mechanical processes for the separation of gas or liquid streams. Membranes are classified as a thin layer barrier for size differential separation, which are usually integrated with chemical and biological treatments, or as a standalone system in secondary treatment of wastewater [ 44 , 45 ]. For a typical membrane mechanism, there is usually a driving force such as a semi-permeable barrier which controls the rate of movement of components by fractional permeation, and rejection through pores of different sizes as depicted in Figure 1 . The permeation and selective rejection are a function of the membrane pore size and chemical affinity, allows for a product stream devoid of target components. Some advantages and drawbacks are presented in Figure 2 .

Membrane selective permeation for various solutes adapted from Tetteh et al., 2019 [ 45 ].

Some advantages and drawbacks of membrane technology. Adapted from Burggraaf 1996 [ 46 ].
3.1.1 Classification of membranes
3.1.1.1 microfiltration.
Microfiltration (MF) employs a sieving mechanism to retain macromolecules or particles larger than 0.1 μm, or more specifically, in the range of 0.1–10 μm [ 45 ]. Unlike ultrafiltration (UF), reverse osmosis (RO) and nanofiltration (NF), the transmembrane pressure (TMP) for both sides of the membrane is low as a result of the retention of smaller particles. Thus MF requires a relatively small TMP, that is, lower than 2 bars but it may vary from 0.1 to 2 bar [ 47 ]. Larger pore sizes of MF membranes limit the removal of suspended solids, bacteria, viruses, protozoan cysts and on a lesser extent, organic colloids within the region [ 48 ].
3.1.1.2 Ultrafiltration
The performance of ultrafiltration (UF) processes are currently receiving increasing recognition as a pretreatment for desalination and membrane bioreactor applications. UF like MF uses physical sieving as a separation mechanism. The pore size, molecular weight cut-off (MWCO) and pressure for a UF membrane filtration ranges from 0.05 μm to 1 nm, 1–500 kDa and an operating pressure of 1–7 bar [ 47 ]. In effect, UF with a definite MWCO are impermeable to compounds with molecular weights exceeding the MWCO and have demonstrated a 3–6 log removal of chlorine resistant protozoan cysts, colloids, viruses, and coliform bacteria. The use of MF and UF as pretreatment to reverse osmosis (RO) has progressively arose at an industrial scale. Both could serve as pretreatment strategies for NF and RO processes for the reduction of membrane fouling, which is applied as a post treatment to chemical precipitation of organic chemical removal, pH adjustment, and phosphorus, hardness, and metal removal. Fouling is extremely distinguished in UF applications, due to the high molecular weight of fractions retained in relation with the small osmotic pressure differentials, and liquid phase diffusivity. However, this does not negatively influence the demand for UF’s, as any design, configuration and application will be fouled [ 49 , 50 ]. The configuration for application could be influenced by the mechanical stability, hydrodynamic requirement, and cost implications.
3.1.1.3 Ion exchange membranes
Membranes are classified as anion exchange membrane (AEM) if the polymer matrix is embedded with fixed positive charge groups, and vice versa, for cation exchange membranes (CEM) [ 51 ], which involves the permeation of anions/cations, and rejection of cations/anions in the effluent. Electrodialysis (ED), reverse electrodialysis (RED), diffusion dialysis (DD) and the Donnan membrane process (DMP) are examples of such, which usually involves the exchange of ions between solutions across the membrane as shown in Figure 3 . The application of these processes is usually based on the type of effluent which is usually reported as an energy resourceful mechanism of separation by potential gradient.

Schematic diagram of ED adapted from Obotey 2020 [ 47 ].
3.1.1.4 Reverse and forward osmosis
Reverse osmosis (RO) is often referred to as a tight membrane and has been widely used in brackish and WWT. Its effectiveness in desalination was found to be more effective than conventional thermal multistage flashing [ 49 ]. High external pressures of 15 to 150 bars is a result of the hypertonic feed and is usually greater than the osmotic pressure which is applied to retain dissolved solute, and prevent and allow for solvent permeation at a MWCO around 100 Da through diffusion mechanism [ 47 ]. Some advantages of the RO system that have been reported in previous studies include low energy consumption, simple configuration and operation, low membrane fouling tendencies and high rejection of a wide range of contaminants. With a concentration gradient as the driving force, the separation and concentration in forward osmosis (FO) occurs as the concentrated solution (e.g. salts such as NaCl) draws water from a less concentrated feed solution. The use of FO operates at ambient conditions, hence irreversible fouling is low. However, to attain the desired process flow and optimum configuration, ROs are arranged in stages and passes. The sequence of the stages has the concentrate stream of the first stage as the feed inlet to the second stage. In addition, the permeate streams from both stages are directed into one discharge channel.
3.1.1.5 Electro-dialysis (ED) and electro-dialysis reversal (EDR)
These processes combine the principles of electricity generation and ion-permeable membranes in the separation of dissolved ions from water [ 45 ]. A difference in electric potential leads to a transfer of ions from a dilute solution to a concentrated solution through an ion-permeable membrane. During electro dialysis, two types of ion exchange membrane are used as shown in Figure 3 . One is permeable to anions and rejects cations, while the other is permeable to cations and rejects anions. There are also two streams which are the concentrate and the diluate (feed). When an electric current is passed through the system, ions from the diluate migrate into the concentrate through oppositely charged membranes (cations migrate to the cathode whiles anions migrate to the anode). The cations are then retained by the positively charged anion-exchange membrane (AEM). Likewise, the anions are retained by the cation-exchange membrane (CEM). The outcome of this is a feed stream depleted of ions, while the concentrate stream becomes rich in ions [ 44 ].
3.1.2 Applications of membrane technology (MT)
A wider scope of industrial and environmental applications of MT are based on its advantages such as (1) clean technology, (2) energy saving (in most cases) and (3) ability to replace conventional processes; such as filtration, distillation, ion exchange, and chemical treatment systems [ 52 ]. A schematic representation of the applications of MT is depicted in Figure 4 . Other advantages are (4) its ability to produce high-quality products and (5) its flexibility in system design. Because of its multidisciplinary application, this technique is applied in several industries, including water treatment for domestic and industrial water supply, chemical, pharmaceutical, biotechnological, beverages, food, metallurgy, and various separation processes.

Application of membrane processes adapted from Obotey 2020 [ 47 ].
3.2 Microalgal wastewater treatment (MWWT)
Water-security is a perspective which defines the reliable availability of an acceptable quality and quantity of water for health, livelihoods and production; coupled with an acceptable level of water-related risks [ 53 , 54 ]. However, population dynamics and the proliferation of industrial set-ups have induced an imbalance in the water-resource equation. Domestic use of water and the demand for water in the production sector of the economy, coupled with commercial services and the agricultural sector, have surpassed the supply capacity of potable water sources [ 54 ]. The unethical discharge of wastewater from some of these sources results in serious social, health, and environmental problems. In addition, freshwater-scarce nations have the growing need to encourage strategies for water reuse, because of inadequate precipitation and lack of capacity to harvest rainwater, which in turn aims to reduce effluent wastewater disposal. Functional wastewater treatment plants (WWTP) for municipalities across the globe have proven to be highly demanding to run in terms of chemical input and energy. Although the basic stages of treatment are primary, secondary, and tertiary, the effluent from these plants contribute to secondary pollution as they are unable to meet the green-drop guidelines [ 54 ]. Phytoremediation is a green strategy that sequesters residual pollutants from wastewater and renders it potent for re-injection into the water supply system. The use of microalgae-based WWT systems has received serious scrutiny in the research community; and in synergy with industry, various wastewater technologies and strategies have been developed to address specific needs in the sector [ 55 ].
3.2.1 Microalgal intervention
Standard culture media have been optimized for specific microalgae strains and are subsequently modified to cultivate many other strains. These are then used as templates to define wastewater characteristics and to select the microalgal strain or microalgae consortium that would best be able to treat a given wastewater source. The microalgae intervention protocol (MAIP) is mainly designed to rid the effluent wastewater from WWTP of the residual pollutants and concurrently produce high value products, thereby meeting the green-drop requirements [ 2 , 3 ]. MAIP is therefore integrated into regular WWTP and upgrades it to advanced WWTPs (AWWTPs). This in turn confers the ability to sequester nitrates and orthophosphates, which, if unsuccessful will result in eutrophication to be induced and propagated in the receiving waters [ 3 ]. The need to regulate nitrogen and phosphorus discharge to the environment is born out of the following: (i) as free ammonia, ammonia-nitrogen is harmful to fish and other aquatic biota, (ii) ammonia consumes dissolved oxygen (DO) and therefore presents the potential of DO depletion, (iii) both phosphorus and nitrogen are plant nutrients and therefore contribute to eutrophication, (iv) is the NO 3 - ion, nitrate-nitrogen reacts and combines with hemoglobin, which contributes to infant mortality. In addition, nitrate-nitrogen can be reduced to mutagenic nitrosamines in the gastrointestinal tract thereby posing more hazards to infants [ 56 ]. Various research teams [ 57 , 58 , 59 , 60 ] reported the presence of emerging pollutants (EP) in WW and the possible undesirable effects many of them can have on the environment and living organisms. These EP include, among others, pesticides, pharmaceuticals, and personal care products; and some technologies have been proposed for their removal; such as physico-chemical and biological treatment strategies. EP removal using pure microalgae strains has been proven to be effective. However, microalgae-based EP removal technologies have not received appreciable attention in the global research community.
The advocacy for employing microalgae to sequester wastewater nutrients, as a treatment option has attracted global acceptance. However, there are skepticisms in employing wastewaters for microalgal cultivation to produce biomass and bio-products. This is primarily due to the reality that wastewaters are of a wide variety of sources and therefore have a wide range of properties whose stability is in question. Pre-treatment is therefore a necessary stage for microalgal WWT, which imposes on the economy of the process. This brings to bear the necessity to adopt the integrated microalgal WWT protocol [ 61 , 62 , 63 , 64 ].
3.2.2 Microalgal WWT strategies
Aside from the ability of microalgae to sequester NH3-N, NO3- -N and PO43-, microalgae also removes heavy metals as well as organic carbon from wastewater, while preventing secondary pollution. However, previous research has indicated that microalgae can rarely grow in undiluted wastewater due to high concentrations of ammonium and other compounds frequently present in wastewater. Different microalgae species present different growth indices in each wastewater treatment application. It is therefore paramount to select a suitable microalgal strain to treat a given wastewater source. Ungureanu and co-workers [ 63 , 65 ] reported that the microalga C. mexicana recorded the highest removal of N, P and C from piggery wastewater compared with five other species ( C. vulgaris , M. reisseri , Nitzschia cf. pusilla , O. multisporus and S. obliquus ). On cultivation of the microalga C. zofingiensis with piggery wastewater using different dilution ratios, 79.84% of COD, 82.70% of total N and 98.17% of total P were removed [ 63 ]. In another study with V. vulgaris , 60–70% of COD and 40–90% of NH4 + -N were removed from diluted piggery wastewater [ 65 ]. The highest removal percentage was obtained with 20-fold diluted wastewater. Whilst tertiary treatment of municipal wastewater effluent and remediation of animal waste streams are an additional technological and economic pressure on municipalities and farms that threatens their economic sustainability, but at the same time it also presents an opportunity [ 63 ]. However, there are several challenges with current microalgae growth systems. For example, algae grown in an open pond or raceway system are suspended in the water in the presence of soluble and suspended waste and can be extremely difficult to harvest because oilagenous microalgae are approximately 5–10 micrometers in diameter. Many of the highly productive microalgae cannot be easily filtered and harvested through centrifugation which is an expensive unit operation. Algae can be harvested by sedimentation; however, this is a slow process and requires considerable floor space. Metal salts can be used as flocculants to facilitate sedimentation; however, this results in water contamination. Algal pond systems are also susceptible to washout, where algae leaves the system and enters surface waters [ 63 , 65 ]. Integrated microalgal WWT systems are examples of green technology, which incorporates both the conventional WWTP and the microalgal WWT protocol which is primarily considered to address imperative issues such as global warming and climate change. The microalgal biomass generated during wastewater treatment, represents a carbon sink, and thus mitigates the negative effect of CO 2 by photosynthetic sequestration of this greenhouse gas [ 66 ].
3.2.2.1 Open ponds
Open ponds are grouped into natural systems, artificial ponds, and containers. Natural systems include the lakes and lagoons; artificial ponds which are either unmixed open ponds, circular open ponds mixed with a center pivot mixer, or raceway ponds; and containers. The commonly used forms include raceway ponds, circular ponds, and tanks, of which raceway ponds have received the most attention [ 64 ].
Waste stabilization ponds are used for wastewater treatment by tens of thousands of small communities around the world. These ponds are low cost, simple to operate and provide effective wastewater treatment in terms of organic carbon and pathogen removal. However, phosphorus removal in waste stabilization ponds is often low, generally between 15 and 50% [ 62 , 64 ]. Because of this, there is increasing pressure from regulators to upgrade pond systems to prevent eutrophication of receiving water bodies. The problem is that current upgrade options often involve the use of chemical dosing which contributes to secondary pollution that makes recovery and reuse of the phosphorus very difficult, and in some cases almost impossible. What is needed is a sustainable low-cost solution to remove phosphorus from the wastewater and ideally allow the phosphorus to be recovered and reused. A potentially emerging environmental process technology has been identified whereby microalgae in waste stabilization pond systems may be triggered to excessively accumulate phosphorus within their cells. While microalgae in lakes can store polyphosphate there is the potential of using this natural phenomenon to optimize for phosphorus removal in algal wastewater treatment ponds [ 62 , 63 ].
Figure 5(A) Is the raceway pond that uses a motorized paddle wheel (PW) to initiate and sustain movement and mixing of the microalgal cell (MCs,) thereby preventing them from settling to the reactor bed. It enhances exposure of the MC to light and nutrients and promotes interphase mass transfer. However, while the mixing energy requirement of a PW is relatively low, efficiency of gas transfer is also low. In some instances, aerators are used to supplement CO 2 to improve microalgae growth, and hence promote nutrient sequestration from the broth. The pond operates at the prevailing temperature and light intensity depends on the incoming solar insolation [ 68 ]. Figure 5(B) is a rectangular open unmixed pond (ROP). The MCs here do not have the privilege of equal exposure to light. The MCs that are near the bottom are shielded from light by those above, thereby creating blind zones to photosynthetic activities resulting in reduction in cell density (CD) and productivity. Figure 5(C) shows open circular containers (OCC) which are unmixed. Figure 5(D) shows circular open pond systems (COPS) equipped with mixers [ 15 , 16 ].

Microalgal open pond systems [ 66 , 67 , 68 ].
3.2.2.2 Closed bioreactor (CBR) systems
Closed photobioreactor systems are characterized by (i) efficient photosynthetic activities associated with adequate control of the operational variables, (ii) lower risk of contamination and (iii) minimization of water loss by evaporation, which is a serious concern in open systems. However, closed systems are more expensive, since they must be constructed with transparent materials, and are more complicated to operate and challenging to scale up. Closed photobioreactors vary in configuration, and the main types are bubble columns, airlift reactors, tubular (loop) and stirred tank reactors. Photobioreactors employing microalgae to treat wastewater and produce biomolecules have (i) elevated efficiency in the use of light energy, (ii) an adequate mixing system, (iii) ease of control of the reaction conditions, (iv) reduced hydrodynamic stress on the cells [ 69 , 70 , 71 ].
Figure 6 gives a pictorial view of photobioreactor scenarios for bubble column, airlift, and annular configurations. A bubble column reactor is basically a cylindrical vessel with a gas distributor at the bottom. The gas is sparged in the form of bubbles into either a liquid phase or a liquid–solid suspension without mechanical agitation. During operation, mixing and CO 2 mass transfer are carried out through the action of the spargers with an external light supply. The configuration of a gas sparger is important since it determines the properties of bubbles; such as bubble size, which in turn affects gas hold-up and other hydrodynamic parameters associated with bubble columns. Photosynthetic efficiency depends on the gas flow rate, which further depends on the photoperiod as the liquid is circulated regularly from central dark zones to external photic zones. This exposes more MCs to the nutrients in the medium, which in the context of this chapter, is wastewater. Photosynthetic efficiency can be increased by increasing the gas flow rate (≥ 0.05 m/s), which in turn leads to shorter photoperiods [ 69 , 70 ]. This type of reactor has advantages of higher mass transfer rates; and low operational and maintenance costs due to fewer moving parts. However, back-mixing and coalescence have been identified as major challenges for these reactors. There is an upper limit for increasing the flow rate, beyond which the heterogeneous flow formed will eventually cause the back-mixing of gas components. Scalability and economics of microalgae cultivation using photobioreactors remain the challenges that have to be overcome for large-scale microalgae production.

Bubble column reactors Płaczek et al., 2017 [ 47 ].
Hom-Diaz and co-workers [ 57 ], in an outdoor pilot 1200 L microalgal photobioreactor (PBR) used toilet wastewater (WW) and evaluated the PBR’s ability to remove pharmaceutically active compounds (PhACs). Nutrients (ammonia-nitrogen, nitrate-nitrogen and total phosphorous) were removed and chemical oxygen demand (COD) was efficiently reduced to the extent of 80%, whilst as much as 48% of the pharmaceutical residues were removed, thereby satisfying the green-drop requirement.
Airlift photobioreactors comprise of two interconnecting zones, called the riser and the down-comer, in an annular setup. Generally, there are two types of airlift photobioreactor: (i) the internal-loop and (ii) the external-loop [ 19 ]. For the internal-loop airlift reactor, the two regions are separated by either a draft tube or a split cylinder, whilst for an external-loop airlift reactor, the riser and down-comer are separated physically by two different tubes. Mixing is done by bubbling the gas through a sparger in the riser tube, with no mechanical agitation. A riser is synonymous with bubble column, where sparged gas moves upward randomly and haphazardly, and decreases the density of the riser making the liquid move upward. Gas hold up in the down-comer significantly influences the fluid dynamics of the airlift reactor thus forcing the liquid downwards The external-loop which is a draft tube confers certain advantages to the airlift bioreactor, namely, preventing bubble coalescence by directing them in one direction; distributing shear stresses more evenly throughout the reactor. This exposes more MCs to the nutrients, minerals, volatile organic compounds and a host of other pollutants for sequestration and for cell growth; enhancing the cyclical movement of fluid, thus increasing mass and heat transfer rates [ 71 , 72 , 73 ].
Fully closed tubular photobioreactors are potentially attractive for large-scale axenic culture of microalgae and is one of the more suitable types for outdoor mass culture. Tubular photobioreactors consist of an array of straight, coiled, or looped transparent tubes that are usually made of transparent plastic or glass. Algae are circulated through the tubes by a pump, or airlift technology [ 21 ].
Many factors contribute to the inability of microalgae to remove nutrients and produce biomass. Some minerals, such as calcium, iron, silica, magnesium, manganese, potassium, copper, sulfur, cobalt, and zinc, also influence microalgae development in wastewater, along with pH, temperature, light, mixing, and dissolved oxygen, which influence development rates and chemical composition of microalgae in wastewater treatment systems [ 74 , 75 ].
3.2.3 Benefits of microalgal WWT
treating diverse kinds of wastewater including domestic, commercial, agricultural, and industrial wastewater
reducing pollutants and pathogens
recovering nutrients as biomass
mitigating CO 2 gas emissions
recovery of metabolites and
energy savings
Starch-based textile de-sizing wastewater (TDW) was treated with the microalgae, Scenedesmus sp . to remove organic carbon with lab-scale reactors, which achieved 92.4% color removal, reduction in chemical oxygen demand (COD) by 89.5%, carbohydrates by 97.4% and organic acids by 94.7% [ 22 ]. Phasey and co-workers [ 23 ] averred that cultivation of microalgae using municipal and agricultural wastewater in high rate algal ponds (HRAP) partitions nutrients into microalgal biomass, which can be recovered and reused.
3.2.4 Microalgal WWT challenges
In spite of all the advantages, some challenges have to be surmounted before the microalgal WWT protocol can be applied. The challenges include (1) land requirement, (2) effect of wastewater characteristics, (3) environmental and operational condition influence and (4) biomass harvesting and valorization [ 14 ]. However, limitations such as algae biomass separation from water, process efficiency in cold climates and limited ability of the algae biomass to reduce micropollutant content in wastewater discourages full-scale use [ 77 ].
3.3 Microbial fuel cells for wastewater treatment
In order to build a sustainable platform for the future society needs to substantially reduce its reliance on fossil fuels. This reduction can then minimize the global scale of pollution. As has been discussed in this chapter, these two global challenges could be concurrently addressed through the application of wastewater treatment technologies which reduce pollution and provide the starting blocks for biofuels. In recent years, a paradigm shift has occurred where wastewater, which can also be referred to as waste matter, is being used by industries generate electricity. In particular, studies have illustrated that a number of biological processing methods can be used to produce bioenergy or bio-chemicals while treating industrial wastewater. Specifically, brewery wastewater treatment has been highlighted for the application of microbial fuel cells (MFCs) [ 78 ]. One such instance of this method is using MFCs to simultaneously treat wastewater and produce bioenergy which is most referred to as bioelectricity. Production of these bio-products happens from simply converting the organic and chemical energy contained in wastewater to electrical energy. To further explore these possibilities, this section first describes an MFC, second it discusses applications of MFCs in wastewater treatment, and thirdly it reviews the different techniques and operations that use MFCs to treat wastewater while concurrently producing electricity. In addition, it also describes other applications and bioenergy products of this technique, its advantages and disadvantages, further promising applications of the MFC technology in wastewater treatment. An MFC is a device that converts organic matter to electricity using microorganisms as the biocatalyst. Typical MFCs have three major components: electrodes, separator, and electrogens. All MFCs contain two electrodes, which, depending on the design, can either be separated into one or two chambers. These chambers operate as completely mixed reactors. As illustrated in Figure 7 below, each electrode is placed on each side of the membrane, which can either be a proton exchange membrane (PEM) or a cation exchange membrane (CEM). The anode faces the chamber that contains the liquid phase, and the cathode faces the chamber that only contains air [ 79 ].

Schematic diagram and pictures of a typical double-chamber microbial fuel cell (MFC), sourced from Logan et., 2006 [ 78 ].
Aforementioned literature proposed the use of carbon, graphite, and metal-based materials as electrodes. For example, materials made from carbon cloth, carbon paper, carbon felt [ 80 ], graphite granules, carbon mesh [ 81 ], platinum, platinum black and activated carbon with single or tubular or multi-electrode configurations are suitable as electrodes [ 82 ]. These electrodes should have properties which render them biocompatible and stable In addition high electrical conductivity, and large surface area is recommended [ 83 , 84 ]. The cathode can be exposed to air or other additional electron acceptors like permanganate, chromium hexacyanoferrate and azo dye, etc. [ 85 ]. The separator is either a cation exchange membrane [ 86 ] or a salt bridge [ 87 ] which is used to keep the chamber. The potential difference generated between the two chambers drives the electrons to move through the circuit while microbial degradation of wastewater acts as the substrate to generate bioelectricity [ 88 ]. MFCs were first considered to be used to treat wastewater as early as 1991 [ 89 ]. Municipal wastewater contains a multitude of organic compounds that can fuel MFCs. The amount of power generated by MFCs in the wastewater treatment process can potentially halve the electricity demand in a conventional treatment process which consumes a significant amount of electric power for aerating the activated sludge. MFCs yield 50–90% less solids to be disposed of than conventional activated sludge treatment methods. Anaerobic digesters, are sometimes integrated with aerobic sequencing batch reactors to overcome the challenges of sludge disposal [ 90 ]. Furthermore, organic molecules such as acetate, propionate and butyrate can be thoroughly broken down to CO2 and H2O. A hybrid MFC incorporating both electrophiles and anodophiles are especially suitable for wastewater treatment because more organics can be biodegraded by a variety of organics. MFCs using certain microbes display a special ability to remove sulphide as necessary in wastewater treatment [ 91 ]. MFCs can enhance the growth of bio electrochemically active microbes during wastewater treatment, thus enabling operational stabilities. Continuous flow, single-compartment MFCs and membrane-less MFCs are favored for wastewater treatment amidst concerns in scale-up of other technologies [ 92 , 93 , 94 ]. Sanitary waste, food processing wastewater, swine wastewater and corn stover are all favorable biomass sources for MFCs because they are rich in organic matters [ 95 , 96 , 97 ]. Up to 80% of the Chemical Oxygen Demand (COD) can be reduced in some cases [ 96 , 98 ] and a columbic efficiency as high as 80% was obtained by Kim et al. [ 99 ].
MFC technologies are a promising yet novel strategy in wastewater treatment, as the treatment process itself becomes a method to capture energy in the form of electricity or hydrogen gas, rather than being a net consumer of electrical energy. In the early 1990s Kim and colleagues illustrated that bacteria could be used in a biofuel cell as an indicator of the lactate concentration in water [ 80 ], which in turn supports electricity generation [ 81 ]. Although the power generation was low, it was not apparent whether the technology would have much impact on reducing wastewater strength. In 2004 this changed, and the link between electricity generation with MFCs and wastewater treatment was clearly forged when it was proven that domestic wastewater could be treated to practical levels while simultaneously producing electricity [ 82 ]. The amount of electricity produced in this study, while low (26 mW/m2), was considerably higher than previously obtained with other wastewater types. Research conducted prior to 2004 had shown that organic and inorganic matter in marine sediments could be used in a novel type MFC design [ 83 ], making it apparent that a wide variety of substrates, materials and system architectures could be used to generate electricity from organic content with bacterial biomass. Still, power levels in all these applications were relatively low. The final development that raised the current interest in MFCs was peaked when power densities of two orders of magnitude greater was produced in an MFC with the addition of glucose [ 84 ]. This application had no need for exogenous chemical mediators or catalyst thus ensuring this operation was purely biological.
Following these demonstrations, the competition was on to advance a rather practical approach to MFC applications. The first objective being the development of a scalable approach and design of the MFC for various wastewater treatment types [ 78 ]. While the energy that could be harnessed from the wastewater may not be enough to power a typical city, it has been reported that a substantial amount of energy can be used to power the WWTPs. As can be observed in the few studies discussed above on MFC technology, the per capital basis of the energy is not particularly substantial and impressive. Also, it can be noted that the most significant energy savings associated with the use of MFC for wastewater treatment, besides generation of electricity and removal of high strengths pollutants form these recalcitrant substrates, is savings in expenses for aeration and solids handling in typical WWTPs. The main operating costs for wastewater treatment are, aeration, sludge treatment and pumping. It has been argued that aeration alone can account for half of the operational costs at a typical WWTP [ 85 ]. Reducing this cost can also ensure that WWTPs become net producers of energy if MFCs are integrated with other treatment technologies.
3.3.1 Applications of microbial fuel cells in wastewater treatment
Applications of MFCs in wastewater treatment include a variety of advantages like long-term sustainability, use of renewable resources, degradation of organic and inorganic waste, bio-hydrogen production, and removal of compounds like nitrates, etc. [ 86 ]. The electrochemical active microbial community requires an in-depth understanding of its solution chemistry to engage in full-scale implementation and exploitation of MFC technology for electricity generation. [ 9 ]. Under ideal laboratory conditions, these systems have produced power densities of 2 to 20 mW/m 2 [ 87 ]. However, the amount of biomass-based energy produced by microbial processes is very low. It has yet to reach to its full potential to work in pilot scale units. It has also been noted that the success of specific MFC applications in wastewater treatment will depend on the concentrations and biodegradability of the organic matter in the effluent, the wastewater temperature, and the absence of toxic chemicals [ 78 ]. One of the first applications could be the development of a pilot-scale reactor at industrial locations where a high quality and reliable influent is available. Food processing wastewaters and digester effluents are good candidates. Moreover, decreased sludge production could substantially decrease the payback time. In the long term, dilute substrates, such as domestic sewage, could be treated with MFCs, thus decreasing society’s need to invest substantial amounts of energy in their treatment. A varied array of alternative applications could also emerge, ranging from biosensor development and sustained energy generation from the seafloor, to bio-batteries operating with various biodegradable fuels. While full scale, and highly effective MFCs are not yet within our reach, the technology holds considerable promise, and major hurdles will undoubtedly be overcome by engineers and scientist in the near future [ 88 ]. The growing pressure on our environment, and the call for renewable energy sources will further stimulate development of this technology, to full scale plant operation. As part of the aforementioned applications of MFC in wastewater treatment, potential for application of this technology it as a typical sensor for pollutant strength analysis for in situ process monitoring and control [ 89 ]. The proportional correction between the columbic efficacy of MFCs and the strength of the wastewater can propose MFCs to be potential biological oxygen demand (BOD) sensors [ 80 ]. An accurate method to measure the BOD value of a liquid is to calculate its Columbic yield. A number of works, namely [ 80 , 90 ] showed a strong linear relationship between the Columbic yield and the strength of wastewater in BOD concentration range. MFC-type BOD sensors are advantageous because they have excellent operational stability, and good reproducibility and accuracy. An MFC-type BOD sensor constructed with the microbes can be kept operational for over five years without extra maintenance [ 80 ]. These biological sensors promise a longer service life than ordinary versions of BOD sensors reported in literature.
3.3.2 Promising techniques of MFCs in wastewater treatment and electricity valorisation
Waste biomass is a cheap and relatively abundant source of electrons for microbes capable of producing electrical current outside the cell [ 85 ]. Rapidly developing microbial electrochemical technologies, such as microbial fuel cells, are part of a diverse platform of future substantial energy and chemical production technologies. In this section, we discuss the key advances that will enable the use of exo-electrogenic micro-organisms to generate biofuels, hydrogen gas, methane, and other valuable inorganic and organic chemicals. Moreover, this section will scrutinize the crucial challenges for implementing these systems and compare them to similar renewable energy technologies. Although commercial development is already underway in several different applications, ranging from wastewater treatment to industrial chemical production, further studies are still required regarding efficiency, scalability, system lifetimes and reliability of MFCs in the field of wastewater treatment and bioenergy production [ 85 ].
Power generation using domestic wastewater in the flat plate system was developed and found to be capable of continuously generating electricity from the organic matter in the wastewater while undergoing treatment [ 82 ]. Following an acclimation period of approximately 1-month, constant power generation from wastewater was obtained with the Flat Plat Microbial Fuel Cell (FPMFC) over a period of five months. For wastewater containing 2463 mg COD/L, an average power density of 560 mW/m 2 was obtained with a hydraulic retention time (HRT) of 2.0 h (0.22 mL/min flow rate; 164 mg/L log mean COD) and an air flow rate of 2 mL/min with a 470 ohms’ resistor. Under these operating conditions, the COD removal rate was 1.2 mg/L min (58% COD removal), and the maximum power density was achieved at a flow rate of 0.22 mL/min. This power density was about 10% higher than that obtained under typical operating conditions with a 470 ohms’ resistor.
Continuous wastewater treatment and electricity generation using a Single Chamber Microbial Fuel Cell (SCMFC) was successfully piloted with feasible results [ 82 , 91 ]. It was found that the system could generate 26 mW/m 2 at the maximum power density while reducing 80% of the COD. In a specially designed, smaller batch system by Liu et al. [ 92 ] showed that up to 28 mW/m 2 of power could be generated with domestic wastewater. It was further demonstrated that by removing the proton exchange membrane (PEM), they could generate a maximum of 146 mW/m 2 of power. In these systems, the anode was separated from the PEM/cathode or plain cathode in a large chamber, but the anode chamber was not mixed except by the flow of liquid into the system. In other MFCs, the anode chamber was often mixed in [ 93 , 94 , 95 ] . In hydrogen fuel cells, the electrodes are usually combined into a single strip separated by a PEM. This is necessary to keep the two electrodes near to enhance proton conduction between the two electrodes. However, PEMs such as nafion are permeable to oxygen, resulting in the transfer of small amounts of oxygen from the cathode chamber to the anode chamber.
Domestic wastewater treatment was examined under two different temperature gradients, (23 ± 3°C and 30 ± 1°C) and flow modes (fed-batch and continuous) using a single-chamber air–cathode microbial fuel cells (MFCs) in view of the effect of operating parameters which affect the production of electricity [ 94 ]. Temperature was an important parameter which influenced efficiency and power generation. The highest power density of 422 mW/m 2 (12.8 W/m 3 ) was achieved under continuous flow and mesophilic conditions, at an organic loading rate of 54 g COD/L-d with reduction of COD by 25.8%. Energy recovery was found to depend significantly on the operational conditions (flow mode, temperature, organic loading rate, and Hydraulic Retention Time (HRT)) as well as the reactor architecture. The results demonstrate that the main advantages of using temperature gradients, in series MFC configurations for domestic wastewater treatment are power savings, low solids production, and higher treatment efficiencies.
A study on MFCs used to produce electricity from different compounds sources, including acetate, lactate, and glucose has proven its ability in high efficiencies and versatility in applications for wastewater treatment [ 96 ]. Clearly, the possibility to produce electricity in a MFC from domestic wastewater, while at the same time accomplishing biological wastewater treatment (reduction of COD) was emphasized. Tests were conducted using SCMFC containing eight graphite electrodes (anodes) and a single air cathode. The system was operated under continuous flow conditions with primary clarifier effluent obtained from a local wastewater treatment plant. The prototype SCMFC reactor generated electrical power ( maximum of 26 mW/ m 2 ) while reducing the COD by about 80%. The power output was proportional to the hydraulic retention time over a range of 3 to 33 h, and to the influent wastewater strength over a range of 50–220 mg/L for COD. Current generation was controlled primarily by the efficiency of the cathode. Optimal cathode performance was obtained by allowing passive air flow rather than forced air flow (4.5–5.5 L/min). The Columbic efficiency of the system, based on COD reduction and current generation, was <12%, indicating that a substantial fraction of the organic matter was not accessible to the microorganisms thus limiting the current generation. Bioreactors based on power generation in MFCs may represent a completely new approach to wastewater treatment. If power generation in these systems can be increased, MFC technology may provide a new method to offset wastewater treatment plant operating costs, whilst making advanced wastewater treatment more affordable for both developing and industrialized nations.
The development of electric power from MFCs was initially investigated for its potential contribution to applications in space research [ 97 ]. It was discussed that one of the determining factors in MFC technology was the use of applied microbial cultures, which are responsible for converting electric energy from the chemical bonds in the substrates. In the last decade, despite the intensive development there is a knowledge gap regarding electricity production from microbes and the screening for electricity production. The fast screening method was based on microbial iron (III) – reduction, and do not require any MFC infrastructures. The method is suitable for the evaluation of numerous microbe species or strains simultaneously; and in this way there is possibility to extend the range of potential MFC biocatalysts and be able to predict the electricity generation from the chosen cultures. The knowledge which was generated from this study concerning the growth – iron (III) – reduction, substrate utilization, adhering and biofilm forming properties, extracellular conductive proteins and redox mediator production measurements is essential for the utilization of G.toluenoxydans and S. xiamenensis species for the different types of MFC applications (wastewater treatment and/or energy production). This information is vital for further strain-improvement and to create an efficient MFC design for electricity production. S.xiamenensis DSMZ 22215 species can catalyze maltose or maltodextrine efficiently. This ability makes the microbes available to be useful in MFC systems for the treatment of starch-based wastewaters (e.g. Brewery wastewater, starch wastewater and the pulp and paper industry).
Simultaneous wastewater treatment for biological electricity generation, through the membrane electrode assembly air-cathode MFC in starch processing wastewater (SPW) as substrate, was proven in this study [ 82 ]. Over the entire experimentation time, it was perceived that the optimum voltage output of 490.8 mV and power density of 293.4 mW/m 2 was ascertained with a current density of 893.3 mA/m 2 . An internal resistance of 120 ohms was also recorded within the third cycle of experiments. Removal efficiencies for COD and N H 4 + − N increased with time, with a maximum of 98.0% and 90.6%, respectively. This was higher than most reported works on MFC operations. High values of nitrate removal might have been a result of both biological and physiochemical processes. Columbic Efficiency (CE) was not high (maximum 8.0%) and was mainly caused by other electron acceptors in the SPW, and oxygen diffusion during long operation periods. SEM revealed the presence of biofilm on the anode, in which short rod-shaped bacillus might have been the dominating bacteria responsible for MFC operation. This study demonstrated the feasibility of using MFC technology to generate electricity and simultaneously treat SPW with high removals of COD and N H 4 + − N , thus providing an attractive alternative to reduce the cost of wastewater treatment whilst generating electricity from a renewable resource.
3.3.3 Advantages and disadvantages (Limitations) of MFCs in WWT
MFCs present several advantages and disadvantages ( Table 2 ), both operational and functional in comparison to currently implemented wastewater treatment technologies for both high organic pollutant removals in the form of CODs and for the valorization of bioenergy in the form of electricity [ 98 ]. The generation of bioenergy from wastewater treatment is mostly considered to be the green or blue energy aspect of MFCs [ 92 ]. Electricity is generated in a direct way from biomass and organic matter, hence chemical energy is directly converted to electrical energy. The direct conversion of wastewater substrates to bioenergy has also been reported to be a third of the input during the thermal combustion of biogas [ 85 ]. Due to the harvesting of electrical energy, the bacterial growth yield in a MFC is considerably lower than the sludge output of an aerobic process [ 85 , 99 ]. Generally the off-gas of an anaerobic process has a high content of nitrous gases together with the targeted hydrogen and methane [ 78 ]. The off-gases of MFCs has less economic viability, since the energy contained in the substrate was previously directed towards the anodic chamber of the MFC during processing [ 78 ]. The gas produced in the anodic chamber of the MFC can be literally discharged, considering no large amounts or other odorous compounds are present, and in addition no aerosols with noxious or undesired bacterial contents are liberated into the environment. Power generation from MFCs have improved considerably and reached the level of primary power target, at least in small scale systems, but the scale up is still a big challenge and a major limitation of the application of MFC technologies. The high cost of cation exchange membranes, the potential for biofouling and associated high internal resistance restrain the power generation and limit the practicality and commercial application of this technique [ 100 ].
List of advantages and disadvantages of MFCs, sourced from Quach-Cu et al., 2018 [ 61 ].
Domestic wastewater is organic matter with embedded energy content of almost 10 times the energy needed for treatment [ 101 ]. While emerging techniques are promising, none of the processes available today can yet fully extract all the energy available in wastewater without further investment in their research and development [ 100 ]. A major setback of MFC applications is associated with the process start up time, and sequence which may be between 4 to 103 days depending on the inoculum, electrode materials, reactor design and operating conditions (temperature, external loading rates etc.), but it is largely affected by the type of substrate being fed into the MFC system [ 96 ]. Another vital impediment in scaling up of MFCs for wastewater treatment is the shortage of buffer capacity of electrolytes. This might require some external mediators, or chemical substance to maintain and stabilize the hydrogen potential of the anodic and cathodic chambers. This has to enhance the wastewater treatment process but still favor the valorization of bioenergy within the MFC system.
4. Conclusion
In this chapter, we have reviewed the use of the MT, Microalgae and MFC technology, particularly focusing on their strengths and limitations in treating wastewater while producing bioenergy and other viable products. In the case of membrane distillation, continuous studies are needed to adequately understand the concept of temperature polarization and, accordingly, a suitable membrane should be developed to make the process viable for large scale application. Microalgal WWT achieves a dual purpose of reducing wastewater of their pollutants and producing biomass of value. It also adds the benefit of mitigating global warming as microalgae biofix anthropogenic carbon dioxide. Microalgal WWT by the airlift bioreactor technology application has advantages over other available reactor technologies as it maximizes carbon dioxide and oxygen gas mass transfer with high remediation potentials. Presently, MFC technology is at research stage hence more research and practical attempts are a necessity for its commercial viability and applications practically at large scale. Although some of the basic knowledge has been gained in MFC research, there is still a lot to be learned in the scale-up of MFC for real plant application and commercialization.
- 1. Khanzada NK, Farid MU, Kharraz JA, Choi J, Tang CY, Nghiem LD, et al. Removal of organic micropollutants using advanced membrane-based water and wastewater treatment: A review. J. Memb. Sci. 2020.
- 2. Yue X, Zhang T, Yang D, Qiu F, Li Z, Zhu Y, et al. Oil removal from oily water by a low-cost and durable flexible membrane made of layered double hydroxide nanosheet on cellulose support. J Clean Prod. 2018;
- 3. Zhang Y, Wei S, Hu Y, Sun S. Membrane technology in wastewater treatment enhanced by functional nanomaterials. J Clean Prod. 2018;
- 4. Abdel-Raouf N, Al-Homaidan AA, Ibraheem IBM. Microalgae and wastewater treatment. Saudi J. Biol. Sci. 2012.
- 5. Gupta S kumar, Bux F. Application of Microalgae in Wastewater Treatment Volume 1: Domestic and Industrial Wastewater Treatment. Appl. Microalgae Wastewater Treat. Springer; 2019.
- 6. Sukla LB, Pradhan D, Subbaiah T. Future Prospects of Microalgae in Wastewater Treatment. In: Sukla LB, Subudhi E, Pradhan D, editors. Role Microalgae Wastewater Treat. Springer Singapore; 2019. p. 129-35.
- 7. Tang RCO, Jang JH, Lan TH, Wu JC, Yan WM, Sangeetha T, et al. Review on design factors of microbial fuel cells using Buckingham’s Pi Theorem. Renew. Sustain. Energy Rev. 2020.
- 8. Yaqoob AA, Khatoon A, Setapar SHM, Umar K, Parveen T, Ibrahim MNM, et al. Outlook on the role of microbial fuel cells in remediation of environmental pollutants with electricity generation. Catalysts. 2020.
- 9. Yongabi K. Biocoagulants for water and waste water purification: a review. Int Rev Chem Eng. 2010;
- 10. Qasim SR. Wastewater treatment plants: Planning, design, and operation, second edition. Wastewater Treat. Plants Planning, Des. Oper. Second Ed. 2017.
- 11. Von Sperling M. Wastewater Characteristics, Treatment and Disposal. Water Intell Online. 2015;
- 12. Chang EE, Hsing HJ, Chiang PC, Chen MY, Shyng JY. The chemical and biological characteristics of coke-oven wastewater by ozonation. J Hazard Mater. 2008;
- 13. Fazal T, Mushtaq A, Rehman F, Ullah Khan A, Rashid N, Farooq W, et al. Bioremediation of textile wastewater and successive biodiesel production using microalgae. Renew. Sustain. Energy Rev. 2018.
- 14. Enitan AM, Adeyemo J, Kumari S, Swalaha FM, Bux F. Characterization of Brewery Wastewater Composition. Int J Environ Ecol Eng. 2015;
- 15. Munter R. Industrial wastewater characteristics. Water Use Manag. 2000;
- 16. Hu Z, Grasso D. Water Analysis - Chemical Oxygen Demand. Encycl Anal Sci Second Ed. 2004.
- 17. Li D, Liu S. Water Quality Detection for Lakes. Water Qual Monit Manag. 2019.
- 18. Yu P, Cao J, Jegatheesan V, Du X. A real-time BOD estimation method in wastewater treatment process based on an optimized extreme learning machine. Appl Sci. 2019;
- 19. Henze M, Comeau Y. Wastewater characterization. Biol Wastewater Treat Princ Model Des [Internet]. 2008 [cited 2020 Sep 3]. p. 33-52. Available from: https://books.google.com/books?hl=en&lr=&id=41JButufnm8C&oi=fnd&pg=PA33&ots=nSK7g0wE4j&sig=wKmtnw4CGaLlOlm5I-pfjmYkcvc
- 20. Buscio V, Crespi M, Gutiérrez-Bouzán C. Application of PVDF ultrafiltration membranes to treat and reuse textile wastewater. Desalin Water Treat [Internet]. Taylor and Francis Inc.; 2016 [cited 2020 Sep 3];57:8090-6. Available from: http://www.tandfonline.com/doi/full/10.1080/19443994.2015.1021854
- 21. Mateus GAP, Formentini-Schmitt DM, Nishi L, Fagundes-Klen MR, Gomes RG, Bergamasco R. Coagulation/Flocculation with Moringa oleifera and Membrane Filtration for Dairy Wastewater Treatment.
- 22. López Velarde Santos M, Rodríguez Valadéz FJ, Mora Solís V, González Nava C, Cornejo Martell AJ, Hensel O. Performance of a microbial fuel cell operated with vinasses using different cod concentrations. Rev Int Contam Ambient. Centro de Ciencias de la Atmosfera, UNAM; 2017;33:521-8.
- 23. Liu F, Sun L, Wan J, Shen L, Yu Y, Hu L, et al. Performance of different macrophytes in the decontamination of and electricity generation from swine wastewater via an integrated constructed wetland-microbial fuel cell process. J Environ Sci (China). 2020;
- 24. US EPA O of W. 5.2 Dissolved Oxygen and Biochemical Oxygen Demand.
- 25. Islam MMM, Shafi S, Bandh SA, Shameem N. Impact of environmental changes and human activities on bacterial diversity of lakes. Freshw Microbiol Perspect Bact Dyn Lake Ecosyst. 2019.
- 26. Zhang L, Fu G, Zhang Z. Electricity generation and microbial community in long-running microbial fuel cell for high-salinity mustard tuber wastewater treatment. Bioelectrochemistry. 2019;
- 27. Marassi RJ, Queiroz LG, Silva DCVR, Silva FT da, Silva GC, Paiva TCB d. Performance and toxicity assessment of an up-flow tubular microbial fuel cell during long-term operation with high-strength dairy wastewater. J Clean Prod. 2020;
- 28. Fard FA, Yengejeh RJ, Ghaeni M. Efficiency of Microalgae Scenedesmus in the Removal of Nitrogen from Municipal Wastewaters. Iran J Toxicol [Internet]. 2019 [cited 2020 Sep 3];1-6. Available from: http://www.ijt.ir
- 29. Ogwueleka TC, Samson B. The effect of hydraulic retention time on microalgae-based activated sludge process for Wupa sewage treatment plant, Nigeria. Environ Monit Assess [Internet]. Springer; 2020 [cited 2020 Sep 3];192:1-16. Available from: https://link.springer.com/article/10.1007/s10661-020-8229-y
- 30. Canada H. Total Dissolved Solids (TDS).
- 31. USEPA. Biological Nutrient Removal Processes and Costs. J Exp Psychol Gen. 2007;
- 32. Wollmann F, Dietze S, Ackermann JU, Bley T, Walther T, Steingroewer J, et al. Microalgae wastewater treatment: Biological and technological approaches. Eng. Life Sci. 2019.
- 33. Baysal A, Ozbek N, Akm S. Determination of Trace Metals in Waste Water and Their Removal Processes. Waste Water - Treat Technol Recent Anal Dev [Internet]. InTech; 2013 [cited 2020 Sep 3]. Available from: http://dx.doi.org/10.5772/52025
- 34. Akpor OB. Heavy Metal Pollutants in Wastewater Effluents: Sources, Effects and Remediation. Adv Biosci Bioeng. 2014;
- 35. Burakov AE, Galunin E V., Burakova I V., Kucherova AE, Agarwal S, Tkachev AG, et al. Adsorption of heavy metals on conventional and nanostructured materials for wastewater treatment purposes: A review. Ecotoxicol. Environ. Saf. 2018.
- 36. Al-Saydeh SA, El-Naas MH, Zaidi SJ. Copper removal from industrial wastewater: A comprehensive review. J. Ind. Eng. Chem. 2017.
- 37. Farkas K, Walker DI, Adriaenssens EM, McDonald JE, Hillary LS, Malham SK, et al. Viral indicators for tracking domestic wastewater contamination in the aquatic environment. Water Res. 2020.
- 38. Osuolale O, Okoh A. Human enteric bacteria and viruses in five wastewater treatment plants in the Eastern Cape, South Africa. J Infect Public Health. 2017;
- 39. Ahmed W, Angel N, Edson J, Bibby K, Bivins A, O’Brien JW, et al. First confirmed detection of SARS-CoV-2 in untreated wastewater in Australia: A proof of concept for the wastewater surveillance of COVID-19 in the community. Sci Total Environ. 2020;
- 40. Alpaslan Kocamemi B, Kurt H, Hacioglu S, Yarali C, Saatci AM, Pakdemirli B. First Data-Set on SARS-CoV-2 Detection for Istanbul Wastewaters in Turkey. medRxiv. 2020;
- 41. Deblonde T, Cossu-Leguille C, Hartemann P. Emerging pollutants in wastewater: A review of the literature. Int J Hyg Environ Health. 2011;
- 42. Dargnat C, Teil M-J, Chevreuil M, Blanchard M. Phthalate removal throughout wastewater treatment plant. Sci Total Environ. 2009;
- 43. Clara M, Windhofer G, Hartl W, Braun K, Simon M, Gans O, et al. Occurrence of phthalates in surface runoff, untreated and treated wastewater and fate during wastewater treatment. Chemosphere. 2010;
- 44. Díez B, Rosal R. A critical review of membrane modification techniques for fouling and biofouling control in pressure-driven membrane processes. Nanotechnol Environ Eng. 2020;5:1-21.
- 45. Tetteh EK, Rathilal S, Chetty M, Armah EK, Asante-Sackey D. Treatment of water and wastewater for reuse and energy generation-emerging technologies. IntechOpen; 2019.
- 46. Burggraaf AJ, Cot L. Fundamentals of inorganic membrane science and technology. Elsevier; 1996.
- 47. Obotey Ezugbe E, Rathilal S. Membrane Technologies in Wastewater Treatment: A Review. Membranes (Basel). 2020;10:89.
- 48. Pabby AK, Rizvi SSH, Requena AMS. Handbook of membrane separations: chemical, pharmaceutical, food, and biotechnological applications. CRC press; 2015.
- 49. Jiang K, Song B, Shi X, Song T. An overview of membrane computing. J Bioinforma Intell Control. 2012;1:17-26.
- 50. Gai H, Zhang X, Chen S, Wang C, Xiao M, Huang T, et al. An improved tar–water separation process of low–rank coal conversion wastewater for increasing the tar yield and reducing the oil content in wastewater. Chem Eng J. 2020;383:123229.
- 51. Drioli E, Stankiewicz AI, Macedonio F. Membrane engineering in process intensification—An overview. J Memb Sci. 2011;380:1-8.
- 52. Zheng X, Zhang Z, Yu D, Chen X, Cheng R, Min S, et al. Overview of membrane technology applications for industrial wastewater treatment in China to increase water supply. Resour Conserv Recycl. 2015;105:1-10.
- 53. Department of Environmental Affairs D. SOUTH AFRICA ENVIRONMENT OUTLOOK Executive summary | 1 2 nd South Africa Environment Outlook Executive summary A report on the state of the environment. Pretoria; 2016.
- 54. Sershen, Rodda N, Stenström TA, Schmidt S, Dent M, Bux F, et al. Water security in South Africa: Perceptions on public expectations and municipal obligations, governance and water re-use. Water SA [Internet]. South African Water Research Commission; 2016 [cited 2020 Aug 14];42:456-65. Available from: http://dx.doi.org/10.4314/wsa.v42i3.11Availableonwebsitehttp://www.wrc.org .
- 55. Chalivendra S. Bioremediation of wastewater using microalgae. 2014.
- 56. Kendrick M. Algal Bioreactors for nutrient removal and biomass production during the tertiary treatment of domestic sewage[Internet]. Loughborough University; 2011 Jan. Available from: https://repository.lboro.ac.uk/articles/thesis/Algal_bioreactors_for_nutrient_removal_and_biomass_production_during_the_tertiary_treatment_of_domestic_sewage/9456713
- 57. Hom-Diaz A, Jaén-Gil A, Bello-Laserna I, Rodríguez-Mozaz S, Vicent T, Barceló D, et al. Performance of a microalgal photobioreactor treating toilet wastewater: Pharmaceutically active compound removal and biomass harvesting. Sci Total Environ. Elsevier B.V.; 2017;592:1-11.
- 58. Fagan R, McCormack DE, Dionysiou DD, Pillai SC. A review of solar and visible light active TiO2 photocatalysis for treating bacteria, cyanotoxins and contaminants of emerging concern. Mater. Sci. Semicond. Process. 2016.
- 59. Gimeno O, García-Araya JF, Beltrán FJ, Rivas FJ, Espejo A. Removal of emerging contaminants from a primary effluent of municipal wastewater by means of sequential biological degradation-solar photocatalytic oxidation processes. Chem Eng J. 2016;
- 60. Xiong JQ , Kurade MB, Abou-Shanab RAI, Ji MK, Choi J, Kim JO, et al. Biodegradation of carbamazepine using freshwater microalgae Chlamydomonas mexicana and Scenedesmus obliquus and the determination of its metabolic fate. Bioresour Technol. 2016;
- 61. Quach-Cu J, Herrera-Lynch B, Marciniak C, Adams S, Simmerman A, Reinke RA. The effect of primary, secondary, and tertiary wastewater treatment processes on antibiotic resistance gene (ARG) concentrations in solid and dissolved wastewater fractions. Water (Switzerland). 2018;
- 62. Brown N, Shilton A. Luxury uptake of phosphorus by microalgae in waste stabilisation ponds: Current understanding and future direction. Rev. Environ. Sci. Biotechnol. 2014.
- 63. Monfet E, Unc A. Defining wastewaters used for cultivation of algae. Algal Res. 2017.
- 64. Shahot K, Idris A, Omar R, Yusoff HM. Review on biofilm processes for wastewater treatment. Life Sci J. 2014;
- 65. Houser JB, Venable ME, Sakamachi Y, Hambourger MS, Herrin J, Tuberty SR. Wastewater Remediation Using Algae Grown on a Substrate for Biomass and Biofuel Production. J Environ Prot (Irvine, Calif). 2014;
- 66. Mambo MP. Integrated Algae Pond Systems for the Treatment of Municipal Wastewater. 2014.
- 67. Powell N. Biological Phosphorus Removal by Microalgae in Waste Stabilisation Ponds. [Pamerston North]: Massey University; 2009.
- 68. Institute of Environmental Biotechnolgy. Evaluation of integrated algae pond systems for municipal wastewater treatment. !he Belmont Volley ww w P1lot. Scole lAPS Case Study • • 0 • [Internet]. Republic of South Africa: Water research Commission; 2016 [cited 2020 Aug 14]. Available from: www.wrc.org.za
- 69. Jacob-Lopes E, Teixeira T. Microalgae-Based Systems for Carbon Dioxide Sequestration and Industrial Biorefineries. Biomass. 2010.
- 70. Molina Grima E, Belarbi EH, Acién Fernández FG, Robles Medina A, Chisti Y. Recovery of microalgal biomass and metabolites: Process options and economics. Biotechnol Adv. 2003;
- 71. Płaczek M, Patyna A, Witczak S. Technical evaluation of photobioreactors for microalgae cultivation. E3S Web Conf. 2017.
- 72. Cozma P, Gavrilescu M. Airlift reactors: Applications in wastewater treatment. Environ Eng Manag J. 2012;
- 73. Elawwad A, Karam A, Zaher K. Using an algal photo-bioreactor as a polishing step for secondary treated wastewater. Polish J Environ Stud. 2017;
- 74. Lin CY, Nguyen MLT, Lay CH. Starch-containing textile wastewater treatment for biogas and microalgae biomass production. J Clean Prod. 2017;
- 75. Phasey J, Vandamme D, Fallowfield HJ. Harvesting of algae in municipal wastewater treatment by calcium phosphate precipitation mediated by photosynthesis, sodium hydroxide and lime. Algal Res. 2017;
- 76. Molinuevo-Salces B, Riaño B, Hernández D, García-González MC. Microalgae and wastewater treatment: Advantages and disadvantages. Microalgae Biotechnol Dev Biofuel Wastewater Treat. 2019.
- 77. Lavrinovičs A, Juhna T. Review on Challenges and Limitations for Algae-Based Wastewater Treatment. Constr Sci. 2018;
- 78. Logan BE, Hamelers B, Rozendal R, Schröder U, Keller J, Freguia S, et al. Microbial fuel cells: methodology and technology. Environ Sci Technol. 2006;40:5181-92.
- 79. Min B, Cheng S, Logan BE. Electricity generation using membrane and salt bridge microbial fuel cells. Water Res. 2005;39:1675-86.
- 80. Kim HJ, Park HS, Hyun MS, Chang IS, Kim M, Kim BH. A mediator-less microbial fuel cell using a metal reducing bacterium, Shewanella putrefaciens. Enzyme Microb Technol. 2002;30:145-52.
- 81. Kim JR, Min B, Logan BE. Evaluation of procedures to acclimate a microbial fuel cell for electricity production. Appl Microbiol Biotechnol. 2005;68:23-30.
- 82. Liu H, Logan BE. Electricity generation using an air-cathode single chamber microbial fuel cell in the presence and absence of a proton exchange membrane. Environ Sci Technol. 2004;38:4040-6.
- 83. Prasad J, Tripathi RK. Scale up sediment microbial fuel cell for powering Led lighting. Int J Renew Energy Dev. 2018;7:53.
- 84. Rabaey K, Verstraete W. Microbial fuel cells: novel biotechnology for energy generation. TRENDS Biotechnol. 2005;23:291-8.
- 85. Logan BE. Microbial Fuel Cells [Internet]. Hoboken, N.J.: Wiley-Interscience; 2008. Available from: http://search.ebscohost.com/login.aspx?direct=true&db=nlebk&AN=219803&site=eds-live
- 86. Lovley DR. Microbial fuel cells: novel microbial physiologies and engineering approaches. Curr Opin Biotechnol. 2006;17:327-32.
- 87. Ieropoulos IA, Greenman J, Melhuish C, Hart J. Comparative study of three types of microbial fuel cell. Enzyme Microb Technol. 2005;37:238-45.
- 88. Nishika GVYSA, Mongia SAADG, GulatiMarwah IKDR. A Short Review on Microbial Fuel Cell Technology and A Proposed approach for Generation of Electricity using Waste Water Treatment. 2015;
- 89. Moon H, Chang IS, Kang KH, Jang JK, Kim BH. Improving the dynamic response of a mediator-less microbial fuel cell as a biochemical oxygen demand (BOD) sensor. Biotechnol Lett. 2004;26:1717-21.
- 90. Zhang Y, Min B, Huang L, Angelidaki I. Electricity generation and microbial community response to substrate changes in microbial fuel cell. Bioresour Technol. 2011;102:1166-73.
- 91. Lu N, Zhou S, Zhuang L, Zhang J, Ni J. Electricity generation from starch processing wastewater using microbial fuel cell technology. Biochem Eng J. 2009;43:246-51.
- 92. Liu H, Ramnarayanan R, Logan BE. Production of electricity during wastewater treatment using a single chamber microbial fuel cell. Environ Sci Technol. 2004;38:2281-5.
- 93. Wei J, Liang P, Huang X. Recent progress in electrodes for microbial fuel cells. Bioresour Technol. 2011;102:9335-44.
- 94. Wen Q , Kong F, Ma F, Ren Y, Pan Z. Improved performance of air-cathode microbial fuel cell through additional Tween 80. J Power Sources. 2011;196:899-904.
- 95. Ren H, Rangaswami S, Lee H-S, Chae J. A micro-scale microbial fule cell (MFC) having ultramicroelectrode (UME) anode. 2013 IEEE 26th Int Conf Micro Electro Mech Syst. IEEE; 2013. p. 869-72.
- 96. Wang H, Ren ZJ. A comprehensive review of microbial electrochemical systems as a platform technology. Biotechnol Adv. 2013;31:1796-807.
- 97. Szöllősi A, Rezessy-Szabó JM, Hoschke Á, Nguyen QD. Novel method for screening microbes for application in microbial fuel cell. Bioresour Technol. 2015;179:123-7.
- 98. Kalathil S, Khan MM, Lee J, Cho MH. Production of bioelectricity, bio-hydrogen, high value chemicals and bioinspired nanomaterials by electrochemically active biofilms. Biotechnol Adv. 2013;31:915-24.
- 99. Kim I-S, Chae K-J, Choi M-J, Verstraete W. Microbial fuel cells: recent advances, bacterial communities and application beyond electricity generation. Environ Eng Res. 2008;13:51-65.
- 100. Hu Z. Electricity generation by a baffle-chamber membraneless microbial fuel cell. J Power Sources. 2008;179:27-33.
- 101. Shi CY. Mass flow and energy efficiency of municipal wastewater treatment plants. IWA Publishing; 2011.
© 2020 The Author(s). Licensee IntechOpen. This chapter is distributed under the terms of the Creative Commons Attribution 3.0 License , which permits unrestricted use, distribution, and reproduction in any medium, provided the original work is properly cited.
Continue reading from the same book
Edited by Iqbal Ahmed
Published: 01 December 2021
By Vandana Sakhre
849 downloads
By M. Amine Didi
461 downloads
By Yilmaz Yurekli
630 downloads
Articles on Wastewater
Displaying 1 - 20 of 92 articles.
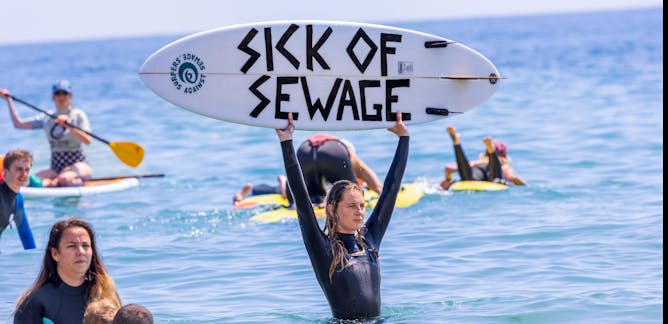
Eight ways to overhaul the UK’s inadequate sewer system
William Perry , Cardiff University
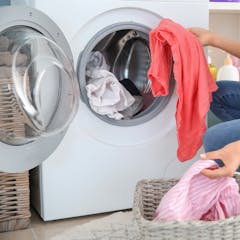
Australians are washing microplastics down the drain and it’s ending up on our farms
Shima Ziajahromi , Griffith University and Frederic Leusch , Griffith University
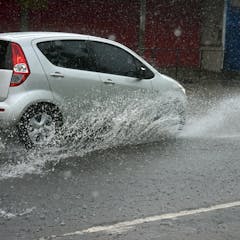
Our cities will need to harvest stormwater in an affordable and green way – here’s how
Buddhi Wijesiri , Queensland University of Technology
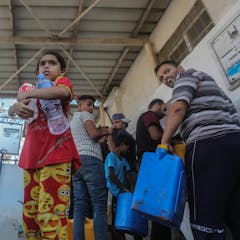
Collaborative water management can be a building block for peace between Israelis and Palestinians
Clive Lipchin , Tel Aviv University and Richard Friend , University of York
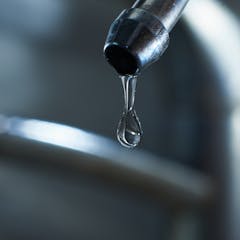
The dams are full for now – but Sydney will need new water supplies as rainfall becomes less reliable
Stuart Khan , UNSW Sydney
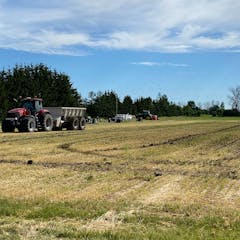
How microplastics are making their way into our farmland
Branaavan Sivarajah , Carleton University and Jesse Vermaire , Carleton University
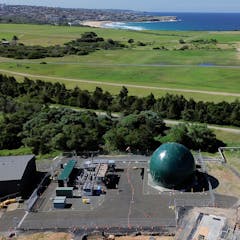
Here’s how wastewater facilities could tackle food waste, generate energy and slash emissions
Melita Jazbec , University of Technology Sydney ; Andrea Turner , University of Technology Sydney , and Ben Madden , University of Technology Sydney
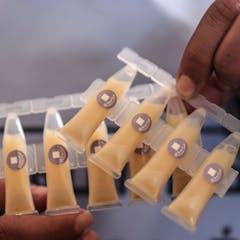
Cholera: vaccines can stop the spread, but the biggest deterrent is clean water
Edina Amponsah-Dacosta , University of Cape Town and Julie Copelyn , University of Cape Town
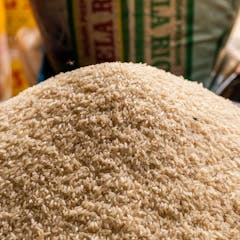
Nigeria is Africa’s leading rice producer, but still needs more - reusing wastewater for irrigation would boost farming
Christopher Oluwakunmi Akinbile , Federal University of Technology, Akure
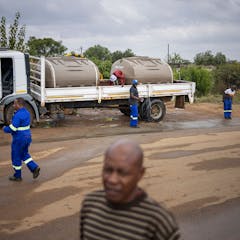
Water quality in South Africa: reports show what needs to be fixed, and at what cost
Kevin Winter , University of Cape Town
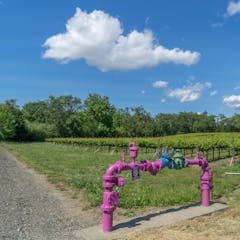
Treated wastewater in Victoria is still contaminated, study finds. So are we and the environment safe?
Mark Patrick Taylor , Macquarie University
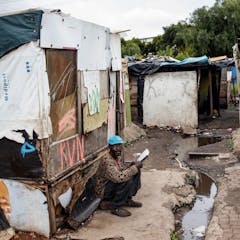
Cholera in South Africa: a symptom of two decades of continued sewage pollution and neglect
Anja du Plessis , University of South Africa
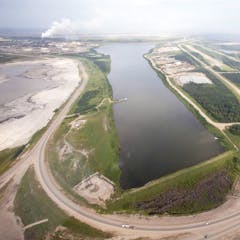
As Alberta’s oilsands continue leaking toxic wastewater, aquatic wildlife face new risks
Diane Orihel , Queen's University, Ontario ; Chloe Robinson , Queen's University, Ontario , and Chris K. Elvidge , Carleton University
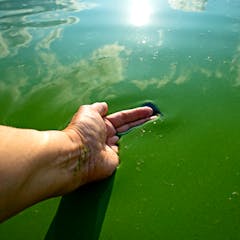
Floods of nutrients from fertilisers and wastewater trash our rivers. Could offsetting help?
Michele Burford , Griffith University
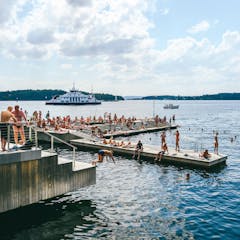
UK waters are too polluted to swim in – but European countries offer answers
Tanja Radu , Loughborough University
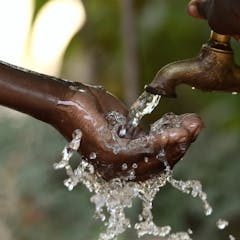
From waste to clean water: tiny carbon particles can do the job
Salam Titinchi , University of the Western Cape
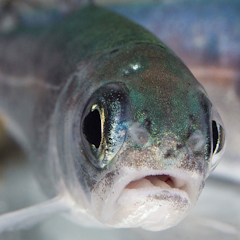
A fishy problem: How antidepressants may impact the health of our aquatic ecosystems
William Andrew Thompson , McMaster University
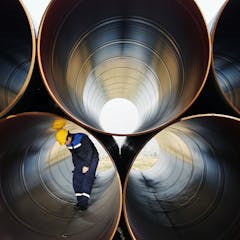
Already under fire politically, Three Waters is also threatened by NZ’s critical shortage of skilled engineers
Suzanne Wilkinson , Massey University and Rod Cameron , Massey University
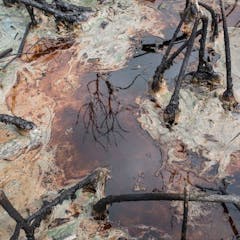
Treatment of wastewater in Nigeria’s oil fields is failing, raising the risk of health hazards
Kingsley Amakiri , University of Huddersfield
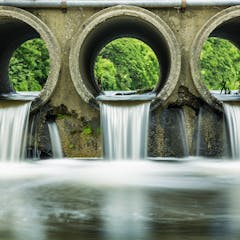
With the Three Waters reforms under fire, let’s not forget that safe and affordable water is a human right
Nathan Cooper , University of Waikato
Related Topics
- Agriculture
- Drinking water
- Wastewater treatment
- Water pollution
- Water treatment
Top contributors
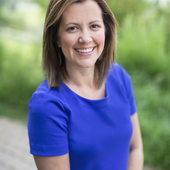
Assistant Professor and Canada Research Chair, Integrated Knowledge, Engineering and Sustainable Communities, University of Calgary
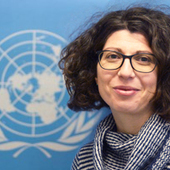
Academic Officer, Water Resource Management Unit lead, Institute for Integrated Management of Material Fluxes and of Resource (UNU-FLORES), United Nations University
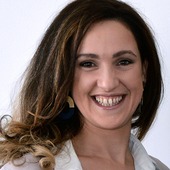
Associate Programme Officer, Institute for Integrated Management of Material Fluxes and of Resources (UNU-FLORES), United Nations University
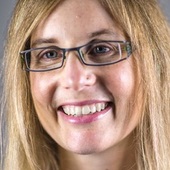
Assistant Professor, Department of Biology & School of Environmental Studies, Queen's University, Ontario
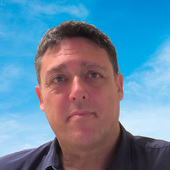
Professor of Civil & Environmental Engineering, UNSW Sydney
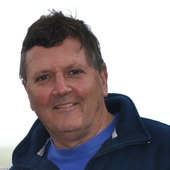
Senior Lecturer in Environmental & Geographical Science, University of Cape Town
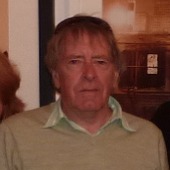
Adjunct Professor, Global, Urban and Social Studies, RMIT University
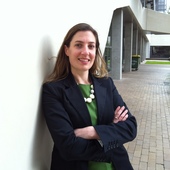
Researcher in sustainable food systems, The University of Melbourne
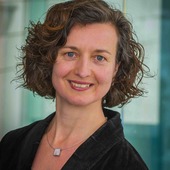
Senior Lecturer in Food Systems, The University of Melbourne
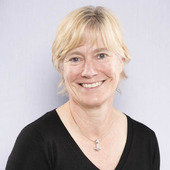
Professor - Australian Rivers Institute, and Dean - Research Infrastructure, Griffith University
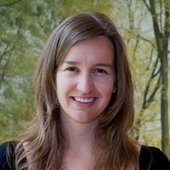
Research Fellow: Food and Urban Systems, University of Helsinki
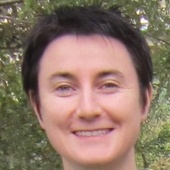
Food Systems Research and Partnerships, The University of Melbourne
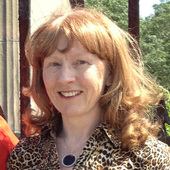
Professor of Geophysics, Durham University
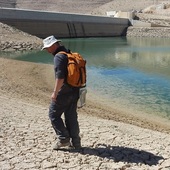
Reader / Associate Professor in Environmental Geoscience, Sheffield Hallam University
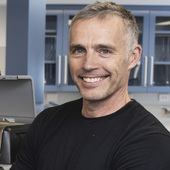
Chief Environmental Scientist, EPA Victoria; Honorary Professor, School of Natural Sciences, Macquarie University
- X (Twitter)
- Unfollow topic Follow topic
Recent research challenges in constructed wetlands for wastewater treatment: A review
- Related Documents
Evaluation of Small Wastewater Treatment Plants in the County of Århus – Denmark
Influent and effluent data from about 120 small wastewater treatment plants (100 - 2000 PE) have been collected and processed. Seven different types of plants are represented. The effluent quality and the treatment efficiency have been evaluated. The most common type of plant is mechanical/biological treatment plants. Some of them are nitrifying and some are also extended for chemical precipitation of phosphorus. Constructed wetlands and biological sandfilters are also represented among the small wastewater treatment plants.
Application of biochar as an innovative substrate in constructed wetlands/biofilters for wastewater treatment: Performance and ecological benefits
Bioaugmented constructed wetlands for efficient saline wastewater treatment with multiple denitrification pathways, piggery wastewater treatment with integrated constructed wetlands, area-based speciation kinetic analysis of the multipollutant removal in constructed wetlands to enhance its treatment efficiency.
In the present study area-based, pollutant removal kinetic analysis was considered using the Zero-order, first-order decay and efficiency loss (EL) models in the constructed wetlands (CWs) for municipal wastewater treatment....
Physiological Responses OfJuncus Effusus(Rush) To Chromium And Relevance For Wastewater Treatment In Constructed Wetlands
The potential for constructed wetlands for wastewater treatment and reuse in developing countries: a review, performance of four full-scale artificially aerated horizontal flow constructed wetlands for domestic wastewater treatment, an overview of water pollution and constructed wetlands for sustainable wastewater treatment in kathmandu valley: a review, constructed wetlands integrated with advanced oxidation processes in wastewater treatment for reuse, export citation format, share document.

An official website of the United States government
Here’s how you know
Official websites use .gov A .gov website belongs to an official government organization in the United States.
Secure .gov websites use HTTPS A lock ( Lock A locked padlock ) or https:// means you’ve safely connected to the .gov website. Share sensitive information only on official, secure websites.
JavaScript appears to be disabled on this computer. Please click here to see any active alerts .
- Wastewater Research
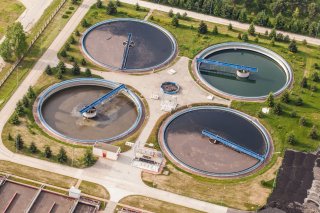
Wastewater management is a critical part of the anthropogenic water cycle that helps ensure that water is clean, safe to use, and protective of ecosystems. Wet weather runoff that flows from urban communities into wastewater collection systems is a significant challenge. Runoff from unpredictable strong storms or continuous heavy rain events can result in rapid changes in flow rates and volumes of wastewater that must be collected and treated, and can exceed design flows for treatment processes at the utility. In addition, the pipe system carrying the water to the facility can exceed its holding capacity, resulting sewer overflows. EPA's wastewater research includes development of methods for detecting and treating contaminants, assessment of antimicrobial resistance (AMR) in wastewater, and the development of methods to monitor municipal wastewater for SARS-CoV2 and other contaminants.
Sign Up for Invites & Updates
Webinar Invites Receive emails for upcoming webinars
Science Matters Newsletter Stay current with research highlights
Research Topics
- Wastewater Contaminants
- Alternative Wastewater Disinfectant Research
- Water Reuse
- Stormwater Management and Green Infrastructure
- Enhanced Aquifer Recharge
Models, Methods, and Tools
- EPA Science Models and Research Tools (SMaRT) Search
- Environmental Technologies Design Option Tool (ETDOT)
- Non-potable Environmental and economic Water Reuse calculator (NEWR)
- PFAS Analytical Methods Development and Sampling Research
- Water Modeling Tools for Decision Support
- Method 1622: Cryptosporidium in Water by Filtration/IMS/FA (2005)
- Method 1623: Giardia Report Form (pdf)
- Method 1622/1623 Spiking Suspension Enumeration Form: Cryptosporidium Giardia (pdf)
- Hemacytometer Data Sheet for Cryptosporidium Giardia (Circle One), July 1998 (1998) (pdf)
Related Resources
- EPA information on Municipal Wastewater
- EPA information on Industrial Wastewater
- Water Research Webinar: SARS CoV-2 in Wastewater Monitoring: Linking Research and Application to Meet Immediate Needs (September 20, 2020)
- Water Research Home
- Watersheds Research
- Nutrients and Harmful Algal Blooms Research
- Drinking Water Research
- Alternative Water Sources Research
- Stormwater Management Research
- PFAS Research
- Technical Support for Communities
- Water Research Grants
- Research Outputs
- Training, Outreach, and Engagement
- Today's news
- Reviews and deals
- Climate change
- 2024 election
- Fall allergies
- Health news
- Mental health
- Sexual health
- Family health
- So mini ways
- Unapologetically
- Buying guides
Entertainment
- How to Watch
- My Portfolio
- Stock Market
- Biden Economy
- Stocks: Most Actives
- Stocks: Gainers
- Stocks: Losers
- Trending Tickers
- World Indices
- US Treasury Bonds
- Top Mutual Funds
- Highest Open Interest
- Highest Implied Volatility
- Stock Comparison
- Advanced Charts
- Currency Converter
- Basic Materials
- Communication Services
- Consumer Cyclical
- Consumer Defensive
- Financial Services
- Industrials
- Real Estate
- Mutual Funds
- Credit Cards
- Balance transfer cards
- Cash-back cards
- Rewards cards
- Travel cards
- Personal Loans
- Student Loans
- Car Insurance
- Options 101
- Good Buy or Goodbye
- Options Pit
- Yahoo Finance Invest
- EV Deep Dive
- Fantasy football
- Pro Pick 'Em
- College Pick 'Em
- Fantasy baseball
- Fantasy hockey
- Fantasy basketball
- Download the app
- Daily fantasy
- Scores and schedules
- GameChannel
- World Baseball Classic
- Premier League
- CONCACAF League
- Champions League
- Motorsports
- Horse racing
- Newsletters
New on Yahoo
- Privacy Dashboard
Yahoo Finance
Water and wastewater treatment market to reach usd 623.2 billion by 2032, says datahorizzon research.
The water and wastewater treatment market size was valued at USD 346.7 Billion in 2023 and is expected to reach a market size of USD 623.2 Billion by 2032 at a CAGR of 6.7%.
Fort Collins, Colorado, April 20, 2024 (GLOBE NEWSWIRE) --
An increase in global population followed by urbanization propels the need for a water and wastewater treatment market
The global population and rapid urbanization have spurred demand for clean water. According to the United Nations, the world's population is projected to reach 9.7 billion by 2050, with 68% residing in urban areas. This population expansion and the proliferation of industrial activities have significantly strained existing water resources. Consequently, governments and organizations worldwide are making substantial investments in water and wastewater treatment infrastructure to ensure their citizens have access to clean water and mitigate environmental harm caused by untreated industrial discharges.
Stringent regulatory measures imposed by governments worldwide to combat water pollution and mandate proper wastewater treatment are major drivers propelling the growth of the water and wastewater treatment market. In the United States, federal laws such as the Clean Water Act and the Safe Drinking Water Act govern water pollution and drinking water quality standards. Similarly, the European Union has enacted the Water Framework Directive to safeguard and enhance water resource quality. These regulations necessitate the adoption of advanced water and wastewater treatment technologies, stimulating market expansion.
Request Sample Report:
https://bit.ly/43Za4is
Segmentation Overview:
The water and wastewater treatment market has been segmented into chemicals, equipment, end-use, and region.
The filtration segment accounts for a significant market share in 2023
The market segmentation based on equipment includes biological, filtration, sludge treatment, disinfection, and demineralization. Filtration holds a substantial share of the equipment segment of the water and wastewater treatment market. Filtration is crucial as it helps remove suspended solids, particulate matter, and other contaminants from the water.
Municipal end-use is expected to create numerous opportunities for industry developments
The water and wastewater treatment market is segmented by end-use into municipal and industrial. Municipal applications hold a significant share of the market. This can be attributed to the increasing global population, rapid urbanization, and the growing demand for clean and safe water for domestic consumption.
Ask For Discount
https://bit.ly/3JmPVcs
Water and Wastewater Treatment Market Report Highlights:
The water and wastewater treatment market growth is anticipated at a CAGR of 6.7% by 2032.
The rise in demand for clean water and preventive measures against water contamination drives the industry growth.
Asia-Pacific is projected to dominate in the forthcoming years, attributed to a rise in urbanization and an increase in population.
Some prominent players in the water and wastewater treatment market report include Veolia, SUEZ, Xylem, Evoqua Water Technologies, Ecolab, Pentair, Aquatech International, Kurita Water Industries, Alfa Laval, Danaher Corporation, Dow Chemical Company, and others.
Looking Exclusively For Region/Country Specific Report?
https://bit.ly/4cQyQ8m
About DataHorizzon Research:
DataHorizzon is a market research and advisory company that assists organizations across the globe in formulating growth strategies for changing business dynamics. Its offerings include consulting services across enterprises and business insights to make actionable decisions. DHR’s comprehensive research methodology for predicting long-term and sustainable trends in the market facilitates complex decisions for organizations.
Mail: [email protected]
Ph: +1-970-672-0390
Website: https://datahorizzonresearch.com/
Follow Us: LinkedIn
Recent Publications
Fatty Acid Market Size
Facades Market Growth
Reverse Osmosis Membrane Market
GCC Facades Market Forecast
UK Facades Market Analysis
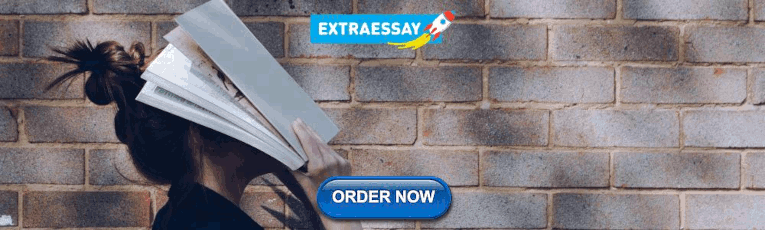
IMAGES
VIDEO
COMMENTS
7.2 Computational Fluid Dynamics in Wastewater Treatment. In recent years, computational fluid dynamics (CFD), a broadly used method, has been applied to biological wastewater treatment. ... Therefore, this review explores the ideas based on current and future research. Wastewater treatment includes very traditional methods by following primary ...
Here, we use a newly developed global wastewater collection and treatment dataset 11, further disaggregated by treatment level (primary, secondary, tertiary+), to delineate these wastewater ...
To accomplish adequate wastewater remediation, the greatest challenge, apart from policy and implementation fronts, lies in maximizing the overall eciency of wastewater treatment (WWT) systems. In light of this, the current review makes a unique eort to help navigate the challenge by summarizing the present scenario of
Feature papers represent the most advanced research with significant potential for high impact in the field. A Feature Paper should be a substantial original Article that involves several techniques or approaches, provides an outlook for future research directions and describes possible research applications. ... "Wastewater Treatment: Current ...
University researchers have shown that a transition to green wastewater-treatment approaches in the U.S. that leverages the potential of carbon-financing could save a staggering $15.6 billion and ...
The most recent research studies in using biosorption for wastewater treatment are ... Membrane methods play a significant role in wastewater treatment and have become a more promising option for ...
Switch to green wastewater infrastructure could reduce emissions and provide huge savings according to new research. ScienceDaily . Retrieved April 20, 2024 from www.sciencedaily.com / releases ...
Water Environment Research is a multidisciplinary water and wastewater research journal, publishing fundamental and applied research related to water quality. Abstract Biological approaches and coagulation are frequently used to reduce the chemical oxygen demand (COD) for treatment of ceramic effluent water.
Recent advances in wastewater treatment processes have resulted in high removal efficiencies for various hazardous pollutants. ... Future research should aim at developing new and low-cost ...
Overall, this Research Topic (RT) provided an appropriate platform for discussing current trends, developments, and applications of integrated treatment technologies in the degradation and detoxification of wastewater or pollutants, including new emerging contaminants, industrial discharges, and municipal wastewater treatment plants. Figure 1.
This report presents the global status on wastewater treatment and acceleration needs to achieve target 6.3 by 2030, based on the latest data on indicator 6.3.1 (total wastewater flows as well as flows from industrial sources and households). ... Download an infographic outlining wastewater sources, collecting systems and treatment; Explore the ...
The U.S. EPA completed a study and report that analyzed the long-term performance of five facilities in the U.S. and one facility in Canada that implemented innovative technologies or process enhancements designed to significantly intensify treatment or enhance the removal of nitrogen or phosphorus species.
The quality of freshwater and its supply, particularly for domestic and industrial purposes are waning due to urbanization and inefficient conventional wastewater treatment (WWT) processes. For decades, conventional WWT processes have succeeded to some extent in treating effluents to meet standard discharge requirements. However, improvements in WWT are necessary to render treated wastewater ...
Electrocatalytic methods are valuable tools for addressing water pollution and scarcity, offering effective pollutant removal and resource recovery. To investigate the current status and future trends of electrocatalysis in wastewater treatment, a detailed analysis of 9417 papers and 4061 patents was conducted using scientometric methods. China emerged as the leading contributor to ...
University researchers have shown that a transition to green wastewater-treatment approaches in the U.S. that leverages the potential of carbon-financing could save a staggering $15.6 billion and just under 30 million tonnes of CO2-equivalent emissions over 40 years. ... He said the results in the paper have supported new research at CSU with ...
Wastewater treatment plants are highly energy-intensive systems. This research uses Life Cycle Assessment (LCA) to determine the impacts generated during the operation of a wastewater treatment plant. Three different scenarios are analyzed: a baseline scenario that considers a conventional activated sludge treatment technology exploiting data from an existing plant located in central Italy, a ...
The Treatment of Wastewater, Recycling and Reuse. - Past, Present, and in the Future. Jyotsana Maura, Suchita Atreya, Anfal Arshi. Defence Institute of Bio-Energy Research (DIBER), DRDO, Haldwani ...
Wastewater treatment using microbes such as fungi, bacteria, microalgae, and others has caught the researcher's attention in recent years. The presence of a significant amount of nutrients such as nitrogen, phosphorus, and carbon in wastewater emanating from different sources can aid in the growth and survival of these microbes [ 5 ].
Sydney's 14 wastewater treatment plants could be modified to also accept food waste, research shows. The 'anaerobic digestion' process would produce energy as well as nutrients for reuse.
Şencan and Yalçuk (2019) evaluated the treatment efficiency of a small-scale hybrid HF-VF CW for potato processing plant wastewater in Turkey. The CW planted with Cyperus alternifolius achieved removals of 87%, 83%, 88% and 82% for COD, NH 4-N, TN and PO 4-P, respectively.The attempt to treat potato processing wastewater was reported only once before (Kadlec et al., 1997).
Hybrid zeolite-based ion-exchange and sulfur oxidizing denitrification for advanced slaughterhouse wastewater treatment. Journal of Environmental Sciences . 10.1016/j.jes.2021.06.002 . 2022 . Vol 113 . pp. 219-230. Author (s): Shuang Tong . Shaoxiang Zhang .
An analysis of the major trends that will impact industrial wastewater treatment in 2024. In the face of escalating environmental challenges and the surging demand for water resources, industrial wastewater treatment solutions stand at the forefront of global priorities. As the world grapples with population growth, urbanization and industrial ...
Evaluation of Small Wastewater Treatment Plants in the County of Århus - Denmark. Influent and effluent data from about 120 small wastewater treatment plants (100 - 2000 PE) have been collected and processed. Seven different types of plants are represented. The effluent quality and the treatment efficiency have been evaluated.
Wastewater Research. Wastewater management is a critical part of the anthropogenic water cycle that helps ensure that water is clean, safe to use, and protective of ecosystems. Wet weather runoff that flows from urban communities into wastewater collection systems is a significant challenge. Runoff from unpredictable strong storms or continuous ...
Abstract. Lawrence K. Wang and Mu-Hao Sung Wang (2023). New Technologies for Water and Wastewater Treatment" In: "Evolutionary Progress in Science, Technology, Engineering, Arts, and Mathematics ...
Switching to greener wastewater treatment approaches could slash carbon emissions and save the country $15.6 billion, a new study has found. Traditional point-source sewage treatment facilities ...
The treatment of petroleum refinery wastewater (PRWW) is of great interest in industrial wastewater management. This wastewater contains a diverse concentration of contaminants such as oil and grease, petroleum hydrocarbons, phenols, ammonia, and sulfides, as well as other organic and inorganic composites. Refinery wastewater treatment has been attempted through various processes, including ...
Among the most effective methods for removing these contaminants in recent times is the adsorption phenomenon. The presence of various contaminants in wastewater can be efficiently reduced by the simple, efficient, and affordable process of adsorption. Selection of suitable adsorbent is the crucial technique in this operation of adsorption.
DataHorizzon Research. The water and wastewater treatment market size was valued at USD 346.7 Billion in 2023 and is expected to reach a market size of USD 623.2 Billion by 2032 at a CAGR of 6.7% ...
Research; Neuroscience; Public Health. Addiction; Covid-19; ... once the only available treatment to prevent preterm birth, ... Medicare expects to spend $3.5 billion on new Alzheimer's drug in 2025